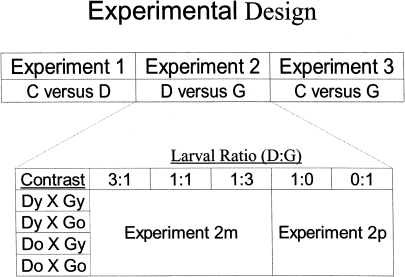
Effects of maternal age on larval competitiveness in house flies
- Select a language for the TTS:
- UK English Female
- UK English Male
- US English Female
- US English Male
- Australian Female
- Australian Male
- Language selected: (auto detect) - EN
Play all audios:

ABSTRACT At advanced ages, many insects lay smaller eggs with reduced viability, but adults produced by different maternal age classes are usually indistinguishable. In most species it is
not known if there are any significant differences between hatchlings from smaller, later eggs (i.e. those produced by old females) and those from larger, earlier eggs (i.e. those produced
by young females). For many insects, the best way to determine if such differences exist is to rear larvae from different maternal age classes together and compare their success. We tested
the effects of maternal age on the competitive ability of house fly larvae, using a modified replacement (substitution) design with pairwise comparisons of two maternal age classes from
three electrophoretically marked lines. For each comparison, known numbers of larvae were reared together at five ratios, including pure cultures, at densities high enough to ensure severe
competition. We measured the effects of maternal age on hatchling to adult survival, development time, and adult size. In general, older females produced larvae that had higher viability and
attained larger sizes, but developed more slowly. Maternal age effects were line-specific, suggesting that they are determined genetically, and there were significant interactions of
maternal age effects between pairwise line comparisons. Maternal age effects on performance in pure culture were not predictive of performance in mixed cultures. Competitor identity
significantly affected the success of each line and maternal age class, suggesting that use of tester strains to determine relative competitiveness of lines, or maternal age classes, is not
generally valid. The results are discussed with respect to the possible adaptive nature of maternal age effects in this species. SIMILAR CONTENT BEING VIEWED BY OTHERS JUVENILE RESPONSES TO
IMMUNE CHALLENGES ARE NOT CARRIED THROUGH TO SUBSEQUENT LIFE STAGES IN AN INSECT Article Open access 13 September 2024 INCREASED PUPAL TEMPERATURE HAS REVERSIBLE EFFECTS ON THERMAL
PERFORMANCE AND IRREVERSIBLE EFFECTS ON IMMUNE SYSTEM AND FECUNDITY IN ADULT LADYBIRDS Article Open access 12 August 2023 PUPAL SIZE AS A PROXY FOR FAT CONTENT IN LABORATORY-REARED AND
FIELD-COLLECTED _DROSOPHILA_ SPECIES Article Open access 27 July 2022 INTRODUCTION Offspring of old female insects (hereafter referred to as late offspring) are usually thought of as
inferior performers, relative to the offspring of young females (hereafter referred to as early offspring). Late offspring are often smaller as hatchlings, arising from smaller eggs with
lower average viability, than their earlier siblings do. They often have decreased growth rates, and larval viability (for reviews see Mousseau & Dingle, 1991a,b; Mousseau & Fox,
1998; McIntyre, 2000). Maternal age effects generally become attenuated as the offspring develop but in some cases they extend to later larval and even adult performance (e.g. adult size of
offspring: Rossiter, 1991; McIntyre & Gooding, 1998). But these later differences are usually measured as performance or fitness components of isolated groups or individuals. There have
been few studies of maternal age effects in which offspring of young and old females interact. Therefore, the intraspecific competitive fitness of offspring of different maternal ages is
essentially unknown in insects, in spite of the considerable body of research apparently addressing it. Most maternal age effects are considered nonadaptive because they are thought to
result from physiological constraints and deterioration (e.g. inability to adequately provision eggs at advanced ages: Murphy et al., 1983). However, many maternal effects are adaptive,
patterned responses of females to predictive cues resulting in improved offspring success (Mousseau & Fox, 1998). For example, the maternal age-related increase in the incidence of
diapause in many seasonal populations corresponds well with the increasing need for offspring to enter diapause as the season advances (Mousseau & Dingle, 1991a,b; Denlinger, 1998).
Furthermore, in species that experience consistent environmental changes during the life of individuals, maternal age effects that improve offspring performance may exist. If, as a result of
seasonal changes or resource depletion, density consistently becomes challenging to their offspring as females age, maternal effects could evolve to avoid or otherwise cope with higher
larval density. An initial step in demonstrating that a maternal age effect is an adaptive response to increasing density is to show that old mothers produce larvae that are more successful
in competition in or avoidance of high density. A common approach used to study maternal age effects is to raise isolated groups of offspring from mothers of various ages and compare
performance among these groups. This approach can not reveal maternal age effects on larval competition, which is an important fitness component in species that have significant larval
interactions. As pointed out by Lewontin (1955) ‘The supposition that one can predict the relative viabilities of genotypes from their absolute viabilities in pure culture is really a
supposition that there is no interaction between these genotypes in exploiting the resources.’ The critical experiment is to raise offspring from different aged females in competition with
each other, as we report in this paper. A common method for comparing competitive ability of two phenotypically indistinguishable strains is to compare both strains to a third,
phenotypically distinct, ‘tester’ strain (e.g. Santos et al., 1992). However, to extend the logic of Lewontin (1955), this assumes that the different genotypes interact with each other in a
common fashion. This assumption is unwarranted, unless there is a single major determinant of competitive success. Studies on the bionomics of house fly larvae suggest that there are two
particularly important phases in their development: initial medium conditioning by hatchlings and growth of the third (final) instar. Survival and adult size of house flies are determined by
the performance of larvae during both of these phases. House fly larvae are bacterial feeders that actively condition their medium through the release of waste ammonium that stimulates
yeast and bacterial growth (Bryant, 1969; Schmidtmann & Martin, 1992). Insufficient early larval density may result in poor medium conditioning and death of larvae through starvation or
fungal takeover of the medium (Bryant & Sokal, 1967). However, with very high initial density, high early mortality can lead to increased total survival and larger flies through reduced
density during the growth phase of the final larval instar (Bryant & Sokal, 1968; Kence & Jdeidi, 1997). Flies developing at elevated larval density are smaller, take longer to
develop, and have lower survival (Sullivan & Sokal, 1963; Bryant & Sokal, 1967). Most of the negative effects of high density occur during the final larval instar, probably as a
result of food depletion (Sullivan & Sokal, 1963). The outcome of larval competition in house flies can not be predicted reliably from performance in pure culture and it depends upon
density, the identity of the competing strains, and the ratio of the strains (Sokal & Sullivan, 1963; Bhalla & Sokal, 1964; Sullivan & Sokal, 1965). In the current study we
confirm these results and extend them to the impact of maternal age on competitive ability. We tested genetically marked strains against each other to address a single main question: Are
there maternal age effects on competitive ability in house flies? This approach allowed us to address the following two corollaries: Do maternal age effects vary between strains? Are
maternal age effects competitor-dependent? The results are discussed with respect to the possible adaptive nature of maternal age effects in house flies and the use of tester strains to
determine relative competitive ability. MATERIALS AND METHODS THE FLIES The history and maintenance of our house fly colony was reported in McIntyre & Gooding (1995, 2000). Three
electrophoretically marked lines were selected from this colony. We used electrophoretic markers because they are more likely to be neutral than are morphological markers, some of which
affect development and competition in house flies (Sokal & Sullivan, 1963; Bhalla & Sokal, 1964; Sullivan & Sokal, 1965). The selected lines differed at either the glutathione
reductase locus (_Gr_), the octanol dehydrogenase locus (_Odh_), or at both loci. In this, and other papers describing work on these _M. domestica_ colonies, the genetically marked lines are
designated C (homozygous _Gr__s_, _Odh__f_), D (homozygous _Gr__s_, _Odh__s_) and G (homozygous _Gr__f_, _Odh__f_). Polyacrylamide gel electrophoresis was used during selection and later to
determine the origin of flies from the competition experiments. We used 7% gels at pH 8.9. Each gel was stained in 25 mL of 50 mM TRIS pH 7.2 containing 9 mg NADP, 9 mg nitro blue
tetrazolium (NBT), 3 mg phenazine methosulphate (PMS), plus substrate; for GSH this was 3 mg reduced glutathione and for ODH 8 drops of octanol. Under these conditions the positions of the
bands, relative to the bromophenol blue front (Rf) values were 0.70 for _Gr__s_, 0.74 for _Gr__f_, 0.35 for _Odh__s_ and 0.39 for _Odh__f_. At _Odh_ and _Gr_ there were actually two very
similar fast alleles. Because we were interested only in using these as markers we did not distinguish between the fast alleles in these experiments. COMPETITION EXPERIMENTS: DESIGN AND
SETUP In order to obtain eggs and larvae for each experiment, populations of ‘young’ and ‘old’ flies were established so the youngest and oldest flies within each age group differed in age
by a maximum of 48 h and the mean difference between flies from different age groups was 14 days. The left wings of a sample of 16 surplus females from each group were slide-mounted and
measured as described below. Eggs were collected twice weekly, beginning at age seven days, from each group of mothers. The eggs used for the competition experiments were taken from 24 ±
1-d-old ‘old’ mothers (the sixth egg collection) and 10 ± 1-d-old ‘young’ mothers (the second egg collection). We chose these ages because 10-d-old females produce the largest eggs with the
highest viabilities, and 24-d-old females produce significantly smaller eggs with lower viabilities but females are not postreproductive and have not begun to suffer significant mortality
(McIntyre & Gooding, 2000). The relative larval competitiveness of young and old house flies were examined using a modified replacement (substitution) series experiment (Mather &
Caligari, 1981; Novak et al., 1993) (Fig. 1), i.e. the groups of interest were reared with each other at several ratios with the same overall initial density. In order to investigate
maternal age effects, our design was more complex because (i) we cannot simultaneously produce offspring from the same mother(s) at two different ages and (ii) we cannot distinguish
offspring from the same kind of mother. For each pairwise combination of marked lines we reared larvae from young and old females of each line in all possible combinations at five seeding
ratios. Because of the fundamental difference between intra-and intergroup competition each experiment contains a logical subdivision into mixed cups (i.e. cups with larvae from two
colonies; Exp. 1m, 2m, and 3m) and pure cups (i.e. cups with larvae from only one colony; Exp. 1p, 2p, and 3p). Throughout this paper we designate the genetically marked lines of _M.
domestica_ as ‘C’, ‘D’ and ‘G’, and the offspring of 10-d-old females as ‘young’ (abbreviated ‘y’) and those of 24-d-old females as ‘old’ (abbreviated ‘o’). We also use these designations in
combination; for example ‘Dy’ identifies offspring from young females of line D and ‘Go’ identifies offspring of old females from line G (Fig. 1). Eggs were collected, on cotton soaked in
evaporated milk from 09.00 to 16.00 hours, at which time they were separated from the adult flies. A sample of eggs was frozen at −20°C in 0.85% saline for later measurement (eggs can be
frozen for up to 1 years this way without significant alteration of size or shape; McIntyre 2000). A second sample of 50 eggs was incubated for 24 h at 25°C. Hatch rate was recorded for
these eggs. The remainder of the eggs were incubated, on the milk-soaked cotton, until 09.00 hours the following morning to allow hatching. Three replicate cups for each combination of line,
age, and ratio were seeded with a total of 60 vigorous larvae in 3 g of larval medium. This high larval density ensures that larvae experience stress and competition. In preliminary
experiments these conditions resulted in ∼70% larval mortality. At emergence, each fly was frozen at −20°C. Flies from the pure cups were dried for 72 h at 60°C and weighed to the nearest
0.1 mg. Both wings of all flies were mounted in Euparol, between two slides clamped with elastic bands to flatten the wings. Flies from mixed cups were electrophoresed to determine their
parentage. WING MEASUREMENT/DRY MASS REGRESSION Four landmarks (the branching point of veins R1 and R3, the intersection of veins C and R1, the intersection of veins C and M1+2, and the
intersection of the posterior crossvein and vein M3+4) were digitized in _x_,_y_ coordinates for the left wing of each fly using a dissecting microscope and camera lucida with a Summasketch
III data tablet (Summagraphics, Seymour, Connecticut). The wing centroid size (square root of the summed squared distance of all landmark coordinates from the mean _x_ and _y_ coordinates of
the landmarks) was calculated and regressed against the teneral dry mass for flies from the pure cups. No significant effects of experiment, sex, line and maternal age on the slope of this
regression were found. Accordingly a single regression, based on all available data, was used to estimate the teneral mass of the flies from the mixed cups. DATA ANALYSES In the experimental
design presented above, two fundamentally different types of competition were studied. In ‘pure cups’, offspring from young or old females from only one line of flies were in competition
with each other. The data obtained permit comparisons of fitness/biological parameters of offspring of young and old females from three different lines, but the data for each group of flies
were derived from individuals that were competing only against others of the same strain and cohort. In mixed cups, larvae were competing against members of their own line and cohort at the
same time that they were competing against members of a different line; the mothers of the larvae from the competing lines may have been the same or different ages (Fig. 1). The data
obtained in mixed cup experiments provide fitness/biological data for two populations (that differed genetically and in some cases that differed also in the age of their mothers) that were
in direct competition with each other, as well as their own kind. Outcomes of these two types of competition will differ if distinct kinds of larvae differ in parameters that affect larval
performance under competition. We chose to keep the analysis of pure and mixed cups entirely separate, because they are essentially different experiments. It is possible to partially combine
the results into unbalanced analysis of variance (ANOVA) models (e.g. Novak et al., 1993), but it is not a completely satisfactory method and introduces several problems. Because the
experimental unit is the replicate cup, and not each fly produced, we calculated a single value from the flies emerging from each cup for sex ratio, viability, teneral mass, and development
time. To examine sex ratio we calculated the angular transformed proportion of male emergents for each replicate cup. Viability in pure cultures was analysed using angular transformed
survival. Viability in mixed cups was analysed using natural log-transformed Haldane’s _V_=(_a_/(_b_ + 1))/(_A_/_B_), where _a_ and _b_ are the number of adults emerging and _A_ and _B_ are
the number of larvae seeded into the culture from each line (Haldane, 1956; Santos et al., 1992). In order to reduce the number of cups for which we were unable to calculate _V_, because of
missing values, we chose the line with the fewest number of cups with zero emergence for the numerators in the calculation of _V_. For pure cups the use of mean mass was inappropriate
because the same number of larvae were not competing throughout the experiment in each cup, given differences in larval mortality, and we were unable to use the number of emergents to
correct for differences in effective larval density, since the assumptions of analysis of covariance were not met. We used natural log-transformed total biomass in ANOVAs of line by age.
Thus, we were assessing effects on productivity and not on fly size _per se_. For mixed cups we used the ratio of the mean mass of each type of fly. Ratios were calculated with the mean of
the more successful line, in terms of viability, as the numerator. Mean development time was analysed in pure cups because development time was not correlated with survival in these
experiments. For mixed cups we used the ratio of the mean development time of each type of fly, calculated in the same fashion as the ratio of mean mass. For each experiment we tested all
variables in separate two-way (line by maternal age) and three-way (parental age line A by parental age line B by seeding ratio) ANOVAs for pure cups and mixed cups, respectively. There were
several mixed cups in the study that produced emergents from only one line. This forced an unbalanced analysis because of missing values. RESULTS PARENTAL DIFFERENCES With the exception of
a single contrast (experiment 1, line D) there were no significant differences between the estimated masses of young and old females, but eggs from old females were significantly smaller
than those from young females in four out of seven cases after Bonferroni correction (Table 1). Eggs of old females had significantly lower viability than those of young females in two of
seven comparisons, but the difference was in the same direction in all cases (Table 1). After pooling data, egg hatch differed significantly between maternal ages (Young: 88.3%; Old: 76.6%;
(χ2=16.58, _P_ < 0.001, d.f.=1), lines (Line C: 93.0%; Line D: 76.7%; Line G: 80.5%; (χ2=22.82, _P_ < 0.001, d.f.=2), and experiments (Exp 1: 88.0%; Exp 2: 80.5%; Exp 3: 80.0%; (χ2=
6.021, _P_=0.049, d.f.=2). In a randomized block ANOVA with line and maternal age as factors and with experiments as blocks, mothers for experiment 1 were slightly smaller than were mothers
for the other experiments (_F_2,216=4.17, _P_=0.016, _R_2=0.036), but produced slightly larger eggs (_F_2,342=8.82, _P_=0.0002, _R_2= 0.047). There were no line, age, or interaction effects
for mass or egg volume. OVERALL SURVIVAL AND SEX RATIO Total survival in experiment 3(m + p) was 12.7%, much lower than the survival rates of 26.0 and 25.3% from experiments 1(m + p) and 2(m
+ p), respectively. In experiment 3m there were 10 cups that produced no adult and seven cups that produced flies from only one of the parental lines. The net result was greater apparent
variability within groups and uneven sample sizes within cells of the ANOVA. Of the 1910 flies that emerged in these experiments, 51.5% were male and the sex ratio did not depart
significantly from 0.5 (χ2=1.913, d.f.=1, _P_=0.166). Within experiments and in the pooled data there were no significant effects of experiment, line, or maternal age on sex ratio (analyses
not shown). Accordingly, sex was excluded from the analyses of viability, mass, or development time. PURE CUPS In pure cups, offspring of old mothers had higher viability and greater total
biomass than did offspring of young mothers (Fig. 2). Line C had higher survival than line D in experiment 1p, and lower survival than line G in experiment 3p, but in experiment 2p survival
of lines D and G did not differ. The maternal age effect differed significantly between lines in expts 2p and 3p (Fig. 2). Line and line-by-maternal age interaction did not affect biomass
except in experiment 1p, in which early line C larvae out-produced early line D larvae. There was no effect of maternal age on development time in expts 1p and 2p, but in experiment 3p late
offspring developed more rapidly than early offspring and this difference was much greater in line C than in line G (Fig. 2). MIXED CUPS Maternal age affected viability in five out of six
cases in the three mixed cup experiments, but the direction of the effect differed between lines and experiments (Table 2, Fig. 3). Figure 3 contains plots of the performance of each
maternal age for each line, relative to the two maternal ages from the competitor line. These plots are modifications of standard replacement plots (De Wit, 1960) designed to facilitate
comparison of competitive differences between maternal age classes. Late C was better than early C when in competition with late D but there was no difference when competing with early D
(Fig. 3a). Against line G, late C did better than early C, especially against late G (Fig. 3e). Early D was more competitive than late D against both ages of line C in experiment 1m (Fig.
3b), but in experiment 2m late D was better than early D against both age classes of G, with a nonsignificant trend for the difference to be greater at lower seeding ratios of G:D larvae
(Fig. 3d). Early G was slightly, but significantly, better than late G against both age classes of D (Fig. 3C). In experiment 3m early G were marginally better than old G in competition with
both age classes of C and there was a greater deficit of C emergents when the proportion of C larvae was high (Fig. 3F). There were few significant effects of maternal age on competitor
mass and development time ratios (Fig. 4). The pattern of effects is consistent with the observation that development time is negatively correlated with mass in these experiments (_r_=−0.25,
_n_=1900, _P_ < 0.0001). Early line D offspring achieved larger sizes and completed development more rapidly than their line C competitors (especially against late line C), but late D
offspring were smaller and developed more slowly than their line C competitors. In experiment 1m there was a small effect of seeding ratio on mass ratio, with line D 9% smaller than line C
at the 1:1 seeding ratio, but >6% larger at the other ratios (_F_2,22=3.72, _P_=0.041). In experiment 2m, even though there were no effects on mass ratios, young line G flies completed
development more rapidly than late line G did, relative to their line D competitors (_F_1,10=13.99, _P_=0.004). There was also a significant interaction between seeding ratio and maternal
age of line G for development time. Early G flies developed 24% more slowly than their D counterparts at the high G:D seeding ratio while late G flies took 3% longer to develop than did
their D counterparts at this ratio. At the other seeding ratios the development time ratios did not differ between maternal ages of line G (_F_2,10=7.76, _P_=0.010). In experiment 3m late
line G flies were significantly larger relative to their line C competitors than were early G flies (_F_1,10=6.76, _P_=0.027). There was also an interaction between seeding ratio and
maternal age of line G for mass ratio. Late G flies were almost 40% heavier than line C flies when the G:C seeding ratio was 1:3, but at other ratios and maternal ages G flies were
approximately 16% lighter than their C competitors (_F_1,10=6.58, _P_=0.028). DISCUSSION We have demonstrated that old female house flies can produce larvae that are more competitive, at
high density, than larvae from early females. In most cases, maternal age was positively associated with larval viability, mass, and development rate. Competitive ability and the
relationship of maternal age to competitive ability were strain specific. The strain- and mixture-specific nature of competitive ability has been demonstrated previously in house flies and
constitutes strong evidence that larval competitive ability is under genetic control (Sokal & Sullivan, 1963; Bhalla & Sokal, 1964; Sullivan & Sokal, 1965). Our results suggest
that maternal age effects on larval competitive ability are also under genetic control. Thus, maternal age effects in larval house flies are more than simple constraints of age-related
physiological deterioration and they may be adaptive in nature. House fly populations increase throughout the summer in temperate areas (Black & Krafsur, 1986). This implies that females
will generally oviposit into more crowded situations as they age. Because larval density has strong life history consequences, females may experience evolutionary pressure to produce better
competitors at advanced ages and the positive maternal age effects we observed on larval competitive ability may be a response to this pressure. The basis of the competitive advantage of
late larvae is unknown. Late larvae were less active than early larvae at the beginning of these experiments (qualitative observation) and they came from smaller eggs (Table 1). Egg size is
not related to egg energy content in house flies (McIntyre & Gooding, 2000) so late hatchlings may have disproportionately larger energy reserves. If initial nutrient levels are very low
then more active larvae might do well at low density because they will condition the medium more rapidly (assuming that activity correlates with medium conditioning) and will search for and
find sufficient food to keep them alive until bacterial growth accelerates. At high larval density, active larvae might exhaust their hatchling energy reserves and the initial food supply,
and starve before medium conditioning takes effect. Less active larvae might be initially less prone to starvation in poor media and could outlast more active larvae. However, less active
larvae might be at a disadvantage in nutrient-rich media and also less able to condition nutrient-poor media in the absence of more active larvae. This explanation fits our data for late D
offspring, which performed poorly in pure culture but performed well when a few competitors, that performed well in pure culture, were present (Table 2, Fig. 3). Similarly, _ge_ house fly
mutants are poor nutrient conditioners that perform well in the presence of larvae with normal nutrient conditioning activity (Bhalla & Sokal, 1964; Bryant, 1969). If the _Gr_f or _Odh_s
allele (or closely linked genes) affect the rate of ageing in female house flies then this might explain line differences in maternal age effects. But line G (homozygous for _Gr_f), which
was least affected by maternal age in competition, exhibited the largest maternal age effects on egg size and viability (Table 1). Line D (homozygous for _Odh_s), exhibited less difference
in egg hatch than other lines and egg size differences were also small, while D was the only line with early larvae to dramatically outperform old larvae in any experiment (1m). In this
line, less age-related change in egg parameters may be related to less extreme maternal age effects upon larvae, but this does not explain the very weak performance of offspring of young D
mothers in pure culture (expts 1p and 2p). One of the most salient features of this study is that the competitive effects of line, maternal age, and competition ratio could not be
generalized. Rather competitive ability varied among combinations of lines and also between pure cups and mixed cups as has been demonstrated previously in house flies and _Drosophila_ spp.
(Lewontin, 1955; Lewontin & Matsuo, 1963; Sokal & Sullivan, 1963; Bhalla & Sokal, 1964; Sullivan & Sokal, 1965). The difficulty in predicting performance in mixtures from
performance in pure culture has led to the use of tester strains to indirectly determine relative competitive abilities of genotypes (e.g. Santos et al., 1992). But, as argued in the
introduction, this requires that either there is a single major determinant of competitive success or the different genotypes interact with each other in a common fashion. These assumptions
are usually not demonstrated prior to use of the tester method. In _Drosophila_, for which the tester method is frequently used (e.g. Mueller, 1988; Santos et al., 1992; James &
Partridge, 1998), feeding rate is often highly correlated with larval success and is sometimes taken as the primary determinant of larval success (Bakker, 1961; Mueller, 1988). However, _D.
melanogaster_ strains are differentially susceptible to metabolic waste accumulation (Weisbrot, 1966; Botella et al., 1985), and subpopulations exhibit distinct, genetically based strategies
for the utilization of the larval medium before and after larval density and waste concentrations increase (Borash et al., 1998). In larval competition studies using _Drosophila_, yeast
growth has been viewed as an undesirable complication and many larval culturing methods provide killed yeast as food in sterile media (Bakker, 1961; Nunney, 1983), in spite of early
demonstrations that live yeast interact with _Drosophila_ larvae (e.g. Gordon & Sang, 1941). This eliminates a facet of the hatchling/microbial interaction known to be important in
species with similar larval ecology. Thus, in _Drosophila_ there is more than a single factor important in determining larval competitive ability. It is therefore inadvisable to adopt the
tester stock method unless the factors acting in a particular contrast are known to be identical to those in other comparisons of interest. Would the tester strain approach have been useful
here? In our competition experiments (mixed cups only) using larval survivorship as the measure of success and ignoring maternal age effects, there are three independent rankings of larval
competitive ability: D > C, G ≫ D, and G > C. If line C had been the tester stock we would have predicted that G ∼ D, which was not the case in experiment 2m. If line D were the tester
strain the resulting prediction is that _G_ ≫ C, which is in the correct direction, but the observed magnitude of the difference is smaller than predicted. If line G were the tester strain,
the prediction that C > D is in the wrong direction. Thus we have a correct qualitative prediction in only a single case, but the magnitude is less than expected. The use of tester
strain competition experiments is clearly suspect in house flies and probably also in other species with complex larval competition dynamics. We invite detractors to develop analytical
models demonstrating that the tester methodology is sound in cases with complex larval dynamics. Existing models of larval competition consider only the amount of food available and the
feeding rate (De Jong, 1976; Nunney, 1983), which reduces to a single larval trait (feeding rate). REFERENCES * Bakker, K. (1961). An analysis of factors which determine success in
competition for food among larvae of _Drosophila melanogaster_. _Arch Neerl Zool_, 14: 200–281. Article Google Scholar * Bhalla, S. C. and Sokal, R. R. (1964). Competition among genotypes
in the housefly at varying densities and proportions (the green strain). _Evolution_, 18: 312–330. Article Google Scholar * Black IV, W. C. and Krafsur, E. S. (1986). Population biology
and genetics of winter house fly (Diptera: Muscidae) populations. _Ann Entomol Soc Am_, 79: 636–644. Article Google Scholar * Borash, D. J., Gibbs, A. G., Joshi, A. and Mueller, L. D.
(1998). A genetic polymorphism maintained by natural selection in a temporally varying environment. _Am Nat_, 151: 148–156. Article CAS Google Scholar * Botella, L. M., Moya, A.,
Gonzalez, M. C. and Mensua, J. L. (1985). Larval stop, delayed development and survival in overcrowded cultures of _Drosophila melanogaster_: effect of urea and uric acid. _J Insect
Physiol_, 31: 179–185. Article CAS Google Scholar * Bryant, E. H. (1969). The fates of immatures in mixtures of two housefly strains. _Ecology_, 50: 1049–4069. Article Google Scholar *
Bryant, E. H. and Sokal, R. R. (1967). The fate of immature housefly populations at low and high densities. _Res Popul Ecol_, 9: 19–44. Article Google Scholar * Bryant, E. H. and Sokal, R.
R. (1968). Genetic differences affecting the fates of immature housefly populations at two densities. _Res Popul Ecol_, 10: 140–170. Article Google Scholar * de Jong, G. (1976). A model
of competition for food. I. Frequency dependent viabilities. _Am Nat_, 110: 1013–1027. Article Google Scholar * de Wit, C. R. (1960). On competition. _Versl Landb Ond_, 66: 1–82. Google
Scholar * Denlinger, D. L. (1998). Maternal control of fly diapause. In: Mousseau, T. A. and Fox, C. W. (eds) _Maternal Effects as Adaptations_, pp. 275–287. Oxford University Press, New
York. Google Scholar * Gordon, C. and Sang, J. H. (1941). The relation between nutrition and exhibition of the gene Antennaless (_Drosophila melanogaster_). _Proc R Soc B_, 130: 151–184.
Google Scholar * Haldane, J. B. S. (1956). Estimation of viabilities. _J Genet_, 54: 294–296. Article Google Scholar * James, A. C. and Partridge, L. (1998). Geographic variation in
competitive ability in _Drosophila melanogaster_. _Am Nat_, 151: 530–537. Article CAS Google Scholar * Kence, M. and Jdeidi, T. (1997). Effect of malathion on larval competition in house
fly (Diptera: Muscidae) populations. _J Econ Entomol_, 90: 59–65. Article CAS Google Scholar * Lewontin, R. C. (1955). The effects of population density and composition on viability in
_Drosophila melanogaster_. _Evolution_, 9: 27–41. Article Google Scholar * Lewontin, R. C. and Matsuo, Y. (1963). Interaction of genotypes detemining viability in _Drosophila busckii_.
_Proc Natl Acad Sci USA_, 49: 270–278. Article CAS Google Scholar * Mather, K. and Caligari, P. D. S. (1981). Competitive interactions in _Drosophila melanogaster_ II. Measurement of
competition. _Heredity_, 46: 239–254. Article Google Scholar * McIntyre, G. S. (2000). Maternal effects on house fly offspring quality. PhD thesis, University of Alberta. * McIntyre, G. S.
and Gooding, R. H. (1995). Pteridine accumulation in _Musca domestica_. _J Insect Physiol_, 41: 357–368, 10.1016/0022-1910(94)00105-P. Article CAS Google Scholar * McIntyre, G. S. and
Gooding, R. H. (1998). Effect of maternal age on offspring quality in tsetse (Diptera: Glossinidae). _J Med Ent_, 35: 210–215. Article CAS Google Scholar * McIntyre, G. S. and Gooding, R.
H. (2000). Egg size, contents, and quality: maternal-age and -size effects on house fly eggs. _Can J Zool_, 78: 1544–1551. Article Google Scholar * Mousseau, T. A. and Dingle, H. (1991a).
Maternal effects in insect life histories. _Ann Rev Entomol_, 36: 511–534. Article Google Scholar * Mousseau, T. A. and Dingle, H. (1991b). Maternal effects in insects: examples,
constraints, and geographic variation. In: Dudley, E. C. (ed.) _The Unity of Evolutionary Biology_, Vol.II, pp.745–761. Dioscoridies Press, Portland, OR. Google Scholar * Mousseau, T. A.
and Fox, C. W. (1998). _Maternal Effects as Adaptations_. Oxford University Press, New York. * Mueller, L. D. (1988). Evolution of competitive ability in _Drosophila_ by density-dependent
natural selection. _Proc Natl Acad Sci USA_, 85: 4383–4386. Article CAS Google Scholar * Murphy, D. D., Launer, A. E. and Ehrlich, P. R. (1983). The role of adult feeding in egg
production and population dynamics of the checkerspot butterfly _Euphydryas editha_. _Oecologia_, 56: 257–263. Article Google Scholar * Novak, M. G., Higley, L. G., Christianssen, C. A.
and Rowley, W. A. (1993). Evaluating larval competition between _Aedes albopictus_ and _A. triseriatus_ (Diptera: culicidae) through replacement series experiments. _Environ Entomol_, 22:
311–318. Article Google Scholar * Nunney, L. (1983). Sex differences in larval competition in _Drosophila melanogaster_: The testing of a competition model and its relevance to
frequency-dependent selection. _Am Nat_, 121: 67–93. Article Google Scholar * Rossiter, M. C. (1991). Maternal effects generate variation in life history: consequences of egg weight
plasticity in the gypsy moth. _Funct Ecol_, 5: 386–393. Article Google Scholar * Santos, M., Fowler, K. and Partridge, L. (1992). On the use of tester stocks to predict the competitive
ability of genotypes. _Heredity_, 69: 489–495. Article Google Scholar * Schmidtmann, E. T. and Martin, P. A. W. (1992). Relationship between selected bacteria and the growth of immature
house flies, _Musca domestica_, in an axenic test system. _J Med Ent_, 29: 232–235. Article CAS Google Scholar * Sokal, R. R. and Sullivan, R. L. (1963). Competition between mutant and
wild-type house-fly strains at varying densities. _Ecology_, 44: 314–322. Article Google Scholar * Sullivan, R. L. and Sokal, R. R. (1963). The effects of larval density on several strains
of the house fly. _Ecology_, 44: 120–130. Article Google Scholar * Sullivan, R. L. and Sokal, R. R. (1965). Further experiments on competition between strains of house flies. _Ecology_,
46: 172–182. Article Google Scholar * Weisbrot, D. R. (1966). Genotypic interactions among competing strains and species of _Drosophila_. _Genetics_, 53: 427–435. CAS PubMed PubMed
Central Google Scholar Download references ACKNOWLEDGEMENTS This work improved by comments from two anonymous reviewers and was supported, in part, by a grant (A-3900) from the Natural
Sciences and Engineering Research Council of Canada, to R.H.G. AUTHOR INFORMATION AUTHORS AND AFFILIATIONS * Department of Biological Sciences, University of Alberta, Edmonton, AB T6G 2E9,
Canada G S McIntyre & R H Gooding Authors * G S McIntyre View author publications You can also search for this author inPubMed Google Scholar * R H Gooding View author publications You
can also search for this author inPubMed Google Scholar CORRESPONDING AUTHOR Correspondence to G S McIntyre. RIGHTS AND PERMISSIONS Reprints and permissions ABOUT THIS ARTICLE CITE THIS
ARTICLE McIntyre, G., Gooding, R. Effects of maternal age on larval competitiveness in house flies. _Heredity_ 85, 480–489 (2000). https://doi.org/10.1046/j.1365-2540.2000.00787.x Download
citation * Received: 28 March 2000 * Accepted: 21 August 2000 * Published: 01 November 2000 * Issue Date: 01 November 2000 * DOI: https://doi.org/10.1046/j.1365-2540.2000.00787.x SHARE THIS
ARTICLE Anyone you share the following link with will be able to read this content: Get shareable link Sorry, a shareable link is not currently available for this article. Copy to clipboard
Provided by the Springer Nature SharedIt content-sharing initiative KEYWORDS * ageing * larval competition * maternal effects * _Musca domestica_ * tester strains