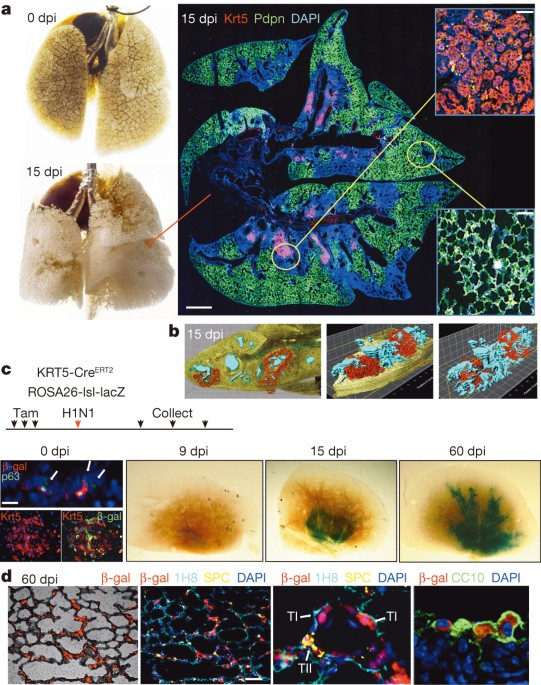
P63+krt5+ distal airway stem cells are essential for lung regeneration
- Select a language for the TTS:
- UK English Female
- UK English Male
- US English Female
- US English Male
- Australian Female
- Australian Male
- Language selected: (auto detect) - EN
Play all audios:

ABSTRACT Lung diseases such as chronic obstructive pulmonary disease1 and pulmonary fibrosis2 involve the progressive and inexorable destruction of oxygen exchange surfaces and airways, and
have emerged as a leading cause of death worldwide. Mitigating therapies, aside from impractical organ transplantation, remain limited and the possibility of regenerative medicine has lacked
empirical support. However, it is clinically known that patients who survive sudden, massive loss of lung tissue from necrotizing pneumonia3,4 or acute respiratory distress syndrome5,6
often recover full pulmonary function within six months. Correspondingly, we recently demonstrated lung regeneration in mice following H1N1 influenza virus infection, and linked distal
airway stem cells expressing Trp63 (p63) and keratin 5, called DASCp63/Krt5, to this process7. Here we show that pre-existing, intrinsically committed DASCp63/Krt5 undergo a proliferative
expansion in response to influenza-induced lung damage, and assemble into nascent alveoli at sites of interstitial lung inflammation. We also show that the selective ablation of DASCp63/Krt5
_in vivo_ prevents this regeneration, leading to pre-fibrotic lesions and deficient oxygen exchange. Finally, we demonstrate that single DASCp63/Krt5-derived pedigrees differentiate to type
I and type II pneumocytes as well as bronchiolar secretory cells following transplantation to infected lung and also minimize the structural consequences of endogenous stem cell loss on
this process. The ability to propagate these cells in culture while maintaining their intrinsic lineage commitment suggests their potential in stem cell-based therapies for acute and chronic
lung diseases. Access through your institution Buy or subscribe This is a preview of subscription content, access via your institution ACCESS OPTIONS Access through your institution
Subscribe to this journal Receive 51 print issues and online access $199.00 per year only $3.90 per issue Learn more Buy this article * Purchase on SpringerLink * Instant access to full
article PDF Buy now Prices may be subject to local taxes which are calculated during checkout ADDITIONAL ACCESS OPTIONS: * Log in * Learn about institutional subscriptions * Read our FAQs *
Contact customer support SIMILAR CONTENT BEING VIEWED BY OTHERS CLONED AIRWAY BASAL PROGENITOR CELLS TO REPAIR FIBROTIC LUNG THROUGH RE-EPITHELIALIZATION Article Open access 03 February 2025
HUMAN RESPIRATORY AIRWAY PROGENITORS DERIVED FROM PLURIPOTENT CELLS GENERATE ALVEOLAR EPITHELIAL CELLS AND MODEL PULMONARY FIBROSIS Article 24 February 2025 SINGLE-CELL DIVISION TRACING AND
TRANSCRIPTOMICS REVEAL CELL TYPES AND DIFFERENTIATION PATHS IN THE REGENERATING LUNG Article Open access 12 March 2024 ACCESSION CODES PRIMARY ACCESSIONS GENE EXPRESSION OMNIBUS * GSE60849
REFERENCES * Barnes, P. J. Chronic obstructive pulmonary disease. _N. Engl. J. Med._ 343, 269–280 (2000) Article CAS Google Scholar * Gross, T. J. & Hunninghake, G. W. Idiopathic
pulmonary fibrosis. _N. Engl. J. Med._ 345, 517–525 (2001) Article CAS Google Scholar * Kerem, E. et al. Bacteremic necrotizing pneumococcal pneumonia in children. _Am. J. Respir. Crit.
Care Med._ 149, 242–244 (1994) Article CAS Google Scholar * Sawicki, G. S., Lu, F. L., Valim, C., Cleveland, R. H. & Colin, A. A. Necrotising pneumonia is an increasingly detected
complication of pneumonia in children. _Eur. Respir. J._ 31, 1285–1291 (2008) Article CAS Google Scholar * Herridge, M. S. et al. One-year outcomes in survivors of the acute respiratory
distress syndrome. _N. Engl. J. Med._ 348, 683–693 (2003) Article Google Scholar * Wilcox, M. E. et al. Radiologic outcomes at 5 years after severe ARDS. _Chest_ 143, 920–926 (2013)
Article Google Scholar * Kumar, P. A. et al. Distal airway stem cells yield alveoli _in vitro_ and during lung regeneration following H1N1 influenza infection. _Cell_ 147, 525–538 (2011)
Article CAS Google Scholar * Taubenberger, J. K. & Morens, D. M. The pathology of influenza virus infections. _Annu. Rev. Pathol._ 3, 499–522 (2008) Article CAS Google Scholar *
Perez-Padilla, R. et al. Pneumonia and respiratory failure from swine-origin influenza A (H1N1) in Mexico. _N. Engl. J. Med._ 361, 680–689 (2009) Article CAS Google Scholar * Matuschak,
G. M. & Lechner, A. J. Acute lung injury and the acute respiratory distress syndrome: pathophysiology and treatment. _Mo. Med._ 107, 252–258 (2010) PubMed PubMed Central Google Scholar
* Short, K. R., Kroeze, E. J., Fouchier, R. A. & Kuiken, T. Pathogenesis of influenza-induced acute respiratory distress syndrome. _Lancet Infect. Dis._ 14, 57–69 (2014) Article CAS
Google Scholar * Li, Y., Gudjonsson, J. E., Woods, T. L., Zhang, T., Johnston, A., Stoll, S. W. & Elder, J. T. Transgenic expression of S100A2 in hairless mouse skin enhances Cxcl13
mRNA in response to solar-simulated radiation. _Arch. Dermatol. Res._ 301, 205–217 (2009) Article CAS Google Scholar * Soriano, P. Generalized lacZ expression with the ROSA26 Cre reporter
strain. _Nature Genet._ 21, 70–71 (1999) Article CAS Google Scholar * Rheinwald, J. G. & Green, H. Serial cultivation of strains of human epidermal keratinocytes: the formation of
keratinizing colonies from single cells. _Cell_ 6, 331–343 (1975) Article CAS Google Scholar * Barrandon, Y. & Green, H. Three clonal types of keratinocyte with different capacities
for multiplication. _Proc. Natl Acad. Sci. USA_ 84, 2302–2306 (1987) Article ADS CAS Google Scholar * Saito, M. et al. Diphtheria toxin receptor-mediated conditional and targeted cell
ablation in transgenic mice. _Nature Biotechnol._ 19, 746–750 (2001) Article CAS Google Scholar * Senoo, M., Pinto, F., Crum, C. P. & McKeon, F. p63 is essential for the proliferative
potential of stem cells of stratified epithelia. _Cell_ 129, 523–536 (2007) Article CAS Google Scholar * Baskerville, A., Thomas, G., Wood, M. & Harris, W. J. Histology and
ultrastructure of metaplasia of alveolar epithelium following infection of mice and hamsters with influenza virus. _Br. J. Exp. Pathol._ 55, 130–137 (1974) CAS PubMed PubMed Central
Google Scholar * Verhoeven, D., Teijaro, J. R. & Farber, D. L. Pulse-oximetry accurately predicts lung pathology and the immune response during influenza infection. _Virology_ 390,
151–156 (2009) Article CAS Google Scholar * Vyalov, S. L., Gabbiani, G. & Kapanci, Y. Rat alveolar myofibroblasts acquire alpha-smooth muscle actin expression during bleomycin-induced
pulmonary fibrosis. _Am. J. Pathol._ 143, 1754–1765 (1993) CAS PubMed PubMed Central Google Scholar * Tsukui, T. et al. Qualitative rather than quantitative changes are hallmarks of
fibroblasts in bleomycin-induced pulmonary fibrosis. _Am. J. Pathol._ 183, 758–773 (2013) Article CAS Google Scholar * Lawson, W. E. et al. Characterization of fibroblast-specific protein
1 in pulmonary fibrosis. _Am. J. Respir. Crit. Care Med._ 171, 899–907 (2005) Article Google Scholar * Rock, J. R., Randell, S. H. & Hogan, B. L. Airway basal stem cells: a
perspective on their roles in epithelial homeostasis and remodeling. _Dis. Model. Mech._ 3, 545–556 (2010) Article CAS Google Scholar * Schmidt, D., Hubsch, U., Wurzer, H., Heppt, W.
& Aufderheide, M. Development of an _in vitro_ human nasal epithelial (HNE) cell model. _Toxicol. Lett._ 88, 75–79 (1996) Article CAS Google Scholar * Uhlen, M. et al. Towards a
knowledge-based Human Protein Atlas. _Nature Biotechnol._ 28, 1248–1250 (2010) Article CAS Google Scholar * Evans, M. J., Cabral, L. J., Stephens, R. J. & Freeman, G. Transformation
of alveolar type 2 cells to type 1 cells following exposure to NO2 . _Exp. Mol. Pathol._ 22, 142–150 (1975) Article CAS Google Scholar * Sugihara, H., Toda, S., Miyabara, S., Fujiyama, C.
& Yonemitsu, N. Reconstruction of alveolus-like structure from alveolar type II epithelial cells in three-dimensional collagen gel matrix culture. _Am. J. Pathol._ 142, 783–792 (1993)
CAS PubMed PubMed Central Google Scholar * Barkauskas, C. E. et al. Type 2 alveolar cells are stem cells in adult lung. _J. Clin. Invest._ 123, 3025–3036 (2013) Article CAS Google
Scholar * Desai, T. J., Brownfield, D. G. & Krasnow, M. A. Alveolar progenitor and stem cells in lung development, renewal and cancer. _Nature_ 507, 190–194 (2014) Article ADS CAS
Google Scholar * Xian, W. & McKeon, F. Adult stem cells underlying lung regeneration. _Cell Cycle_ 11, 887–894 (2012) Article CAS Google Scholar * Becker, K., Jährling, N., Saghafi,
S. & Dodt, H. U. Immunostaining, dehydration, and clearing of mouse embryos for ultramicroscopy. _Cold Spring Harb. Protoc._ 2013, 743–744 (2013) PubMed Google Scholar * Keller, A. et
al. GeneTrailExpress: a web-based pipeline for the statistical evaluation of microarray experiments. _BMC Bioinformatics_ 9, 552 (2008) Article Google Scholar * Subramanian, A. et al. Gene
set enrichment analysis: a knowledge-based approach for interpreting genome-wide expression profiles. _Proc. Natl Acad. Sci. USA_ 102, 15545–15550 (2005) Article ADS CAS Google Scholar
* Mootha, V. K. et al. PGC-1 alpha responsive genes involved in oxidative phosphorylation are coordinately downregulated in human diabetes. _Nature Genet._ 34, 267–273 (2003) Article ADS
CAS Google Scholar Download references ACKNOWLEDGEMENTS This work was supported by grants from the Joint Council Office of the Agency for Science Technology Research Agency (ASTAR),
Singapore (W.X., F.M.), Defense Advanced Research Projects Agency (DARPA, N66001-09-1-2121 to F.M.), the Johnson & Johnson ASTAR Joint Program (W.X., F.M.) and support from Connecticut
Innovations (W.X., F.M.). We thank M. LaLande, B. Lane and H. Hui Ng for support, G. Wright, B. Tennent, B. Knowles and T. McLaughlin for comments on the manuscript, J. Hammer for artwork,
P. Kraus for blastocyst injections, and H. Ahmad and K. L. Goh for technical assistance. We thank H. Green for advice and support. AUTHOR INFORMATION AUTHORS AND AFFILIATIONS * Genome
Institute of Singapore, A-STAR, 138672 Singapore, Wei Zuo, Ting Zhang, Daniel Zheng'An Wu, Shou Ping Guan, Audrey-Ann Liew, Siew Joo Lim, Wa Xian & Frank McKeon * The Jackson
Laboratory for Genomic Medicine, Farmington, 06032, Connecticut, USA Yusuke Yamamoto, Xia Wang, Wa Xian & Frank McKeon * Advanced Cell Technologies, Marlborough, 01752, Massachusetts,
USA Matthew Vincent * The Jackson Laboratory, Bar Harbor, 04609, Maine, USA Mark Lessard * Department of Pathology, Brigham and Women’s Hospital, Harvard Medical School, Boston, 02115,
Massachusetts, USA Christopher P. Crum & Wa Xian * Department of Medicine, National University Health System, 119228 Singapore, Wa Xian & Frank McKeon * Department of Genetics and
Developmental Biology, University of Connecticut Health Center, Farmington, 06030, Connecticut, USA Wa Xian Authors * Wei Zuo View author publications You can also search for this author
inPubMed Google Scholar * Ting Zhang View author publications You can also search for this author inPubMed Google Scholar * Daniel Zheng'An Wu View author publications You can also
search for this author inPubMed Google Scholar * Shou Ping Guan View author publications You can also search for this author inPubMed Google Scholar * Audrey-Ann Liew View author
publications You can also search for this author inPubMed Google Scholar * Yusuke Yamamoto View author publications You can also search for this author inPubMed Google Scholar * Xia Wang
View author publications You can also search for this author inPubMed Google Scholar * Siew Joo Lim View author publications You can also search for this author inPubMed Google Scholar *
Matthew Vincent View author publications You can also search for this author inPubMed Google Scholar * Mark Lessard View author publications You can also search for this author inPubMed
Google Scholar * Christopher P. Crum View author publications You can also search for this author inPubMed Google Scholar * Wa Xian View author publications You can also search for this
author inPubMed Google Scholar * Frank McKeon View author publications You can also search for this author inPubMed Google Scholar CONTRIBUTIONS Experiments were performed by W.Z., D.Z.W.,
S.P.G. and A.-A.L. Experimental design and conception were done by W.Z., M.V., C.P.C., W.X. and F.M.; T.Z., W.Z., X.W., S.J.L. and Y.Y. performed microarrays and computational analysis, and
provided methodological advice. M.L. and W.Z. performed the serial reconstructions of infected lung. CORRESPONDING AUTHORS Correspondence to Wa Xian or Frank McKeon. ETHICS DECLARATIONS
COMPETING INTERESTS The authors declare no competing financial interests. ADDITIONAL INFORMATION Datasets generated for this study have been submitted to the National Center for
Biotechnology Information Gene Expression Omnibus (GEO) database under superseries GSE60849. EXTENDED DATA FIGURES AND TABLES EXTENDED DATA FIGURE 1 LINEAGE TRACING OF KRT5+ CELLS. A, Left,
immunofluorescence images of sections of 15 dpi lung with staining patterns of antibodies to pan-leukocyte marker CD45 and the type I pneumocyte marker Pdpn with DNA counterstained with
DAPI. Right, immunofluorescence images of pan-leukocyte marker CD45 and the type II pneumocyte marker SPC. Scale bar, 150 μm. B, X-gal staining (blue) to reveal lacZ-dependent
β-galactosidase activity in whole lungs after 15 and 40 days post infection following long time gaps between induction of lacZ labelling by tamoxifen and influenza infection-induced lung
damage. The similarity of this long gap labelling and the short gap labelling presented in Fig. 1 argues against prolonged actions of tamoxifen in these lineage-labelling protocols.
Tamoxifen is given at indicated times before infection and no-tamoxifen control is included. C, Immunofluorescence images of colonies of DASCs derived from tamoxifen-treated ROSA26-lsl-lacZ;
Krt5-CreERT2 mice stained with antibodies to keratin 5 (Krt5) or Krt5 and _E. coli_-specific β-galactosidase. D, Histological section of lung at 15 dpi stained with _E. coli_-specific
β-galactosidase antibody and markers of secretory cells (CC10+) and expanded stem cells (Krt5+). Scale bar, 50 μm. E, Whole-mount image of X-gal developed, uninfected lung from
ROSA26-lsl-lacZ; Krt5-CreERT2 which received tamoxifen treatments at −69, −66 and −63 days before dissection. F, Histological section of 60 dpi lung stained with _E. coli_-specific
β-galactosidase antibody and markers of type I pneumocytes (Pdpn+) and type II pneumocytes (SPC+). EXTENDED DATA FIGURE 2 CONDITIONAL DASCP63/KRT5 ABLATION MOUSE MODEL. A, Schematic of
_Krt6a_ locus, the targeting vector constructed to introduce the human diphtheria toxin receptor (DTR). B, The structure of the modified _Krt6a_ locus in embryonic stem cells screened by
Southern blot. C, Co-expression of Krt6 and DTR in Krt5+ pods in 15 dpi lung. D, Histogram showing resistance of wild-type, 12 dpi DASCp63/Krt5 to diphtheria toxin (DTox) and the sensitivity
of DASCp63/Krt5/DTR to diphtheria toxin. _n_ = 3 mice per group. Error bars, s.e.m. EXTENDED DATA FIGURE 3 PERSISTENT DAMAGE IN DASCP63/KRT5-ABLATED LUNGS. A, Gene set enrichment analysis
(GSEA) showing the overrepresentation of normal alveolar signature gene sets in WT rather than Krt6–DTR mouse lungs (whole lobes). For normal alveolar signature build up, laser capture
microdissection of frozen sections was used to dissect normal alveoli region from 0 dpi lung and damaged interstitial infiltrated region from 15 dpi lung for microarray analysis.
Differentially expressed genes (fold change > 5, _P_ < 0.01) were used to develop normal alveolar gene expression signatures. B, Top panel, histological analysis of lung densities
using anti-Pdpn antibodies (red) and anti-CD45 (green) to reveal type I pneumocytes and leukocyte infiltration, respectively. Left, wild-type mice showing apparently normal lung region
adjacent to interstitial density having Pdpn+ network but lacking CD45+ infiltrates. Right, Krt6–DTR lung showing apparently normal region adjacent to zone of damaged interstitial lung
lacking Pdpn+ network but having CD45+ infiltrates. Bottom panel, H&E staining of the same histological region. Scale bar, 100 μm. EXTENDED DATA FIGURE 4 NETWORKS OF TYPE I PNEUMOCYTES
IN 30 DPI MOUSE LUNG. A, Histological analysis of lung densities using anti-Pdpn antibodies (red) and anti-SPC (green) to reveal type I and type II pneumocytes respectively. Left, wild-type
mice showing apparently normal lung region adjacent to interstitial density having Pdpn+ network but lacking SPC+ cells. Right, Krt6–DTR lung showing normal region adjacent to zone of
damaged interstitial lung lacking both pneumocytes. Scale bar, 100 μm. B, Top panel, histological analysis of wild-type lung densities using anti-Pdpn antibodies (red) and anti-Aqp5 (green)
type I pneumocyte markers showing the interstitial density having Pdpn/Aqp5 double-positive network. Bottom panel, wild-type mice show apparently normal lung region adjacent to interstitial
density having Pdpn+ network but the density lack expression of another type I pneumocyte marker, Hopx. Scale bar, 100 μm. EXTENDED DATA FIGURE 5 FAILURE OF REGENERATION IN
DASCP63/KRT5-ABLATED LUNGS. A, Histological section through 30 dpi DASC-ablated lung (Krt6–DTR +DTox) showing normal region (Pdpn+) adjacent to interstitial density positive for α-SMA and
weakly positive for Masson’s trichrome (MT) staining for fibrosis. Scale bar, 100 μm. B, Histological section through 30 dpi control lung showing normal region (Pdpn+) and interstitial
density (Pdpn−) which are both negative for α-SMA. C, Expression heat map of selected, differentially expressed genes (_P_ < 0.05) comparing wild-type mouse lungs with DASC-ablated mouse
lungs at 30 dpi. Scale bar, 100 μm. EXTENDED DATA FIGURE 6 CLONING AND _IN VITRO_ DIFFERENTIATION OF TBSCP63/KRT5 AND DASCP63/KRT5. A, Histogram of cloning efficiency of TBSCs and DASCs on
irradiated 3T3-J2 cells per 1 million tracheal or distal airway cells derived from respective tissues of adult mice. Tissues derived from 3 mice. Error bars, s.e.m. B, Immunofluorescence
images of sections of TBSC and DASC air–liquid interface cultures using an antibody to the type I pneumocyte marker Pdpn (green). Sections were counterstained with DAPI (blue). EXTENDED DATA
FIGURE 7 TRANSPLANTATION OF DASCLACZ. A, Immunofluorescence characterization of DASCs isolated from Krt5-CreERT2;ROSA26-lsl-lacZ mice following Cre activation with 4OH-tamoxifen. From left,
colony stained with antibodies to p63 (green) and Krt5 (red), p63 (green) and _E. coli_ β-galactosidase (red), Krt5 (red) and CC10 (green), and Krt5 (red) and SPC (green). B, Whole mount
image of lung 90 days after infection without stem cell transplantation. C, Left, bright field/immunofluorescence image of section of lung at 90 dpi following transplantation of DASClacZ
stained with antibodies to β-galactosidase (red). Right panels, immunofluorescence images of co-staining of transplanted DASClacZ with antibodies to Pdpn, SPC, or CC10 at high magnification.
EXTENDED DATA FIGURE 8 PERSISTENT PROLIFERATION OF TRANSPLANTED DASC. Co-staining of antibodies to GFP (green) with the cell proliferation marker Ki67 (red) in sections of lung transplanted
with DASCGFP at 12 dpi lung (7 days post transplantation) and 60 dpi lung (55 days post transplantation). Top left, immunofluorescence image of lung following transplantation of DASCGFP (7
days post-transplantation;12 dpi) stained with anti-GFP (green) and the cell cycle marker Ki67 (red, in nucleus). Top right, bronchiole co-stained with antibodies to GFP and Ki67 from 7 days
post-transplantation lung. Bottom, staining of interstitial lung transplanted 55 days prior with DASCGFP with antibodies to GFP and Ki67. Arrows indicate cells co-expressing GFP and Ki67.
EXTENDED DATA FIGURE 9 STEM CELL TRANSPLANTATION REDUCES INTERSTITIAL DENSITIES IN DASCP63/KRT5-ABLATED LUNGS. A, Histological sections through entire lobe of Krt6–DTR mice with (left) and
without (right) diphtheria toxin treatment forty days post-influenza infection. B, Histogram of morphometric quantification of lung densities following 40 day influenza virus infection of
Krt6–DTR mice without diphtheria toxin (−DTox, mouse number _n_ = 3), with diphtheria toxin (+DTox, _n_ = 4), or with diphtheria toxin and transplanted DASCs (+DTox+DASC, _n_ = 4). Error
bars indicate s.e.m. and # indicates _P_ value = 0.029 by Wilcoxon rank-sum test. SUPPLEMENTARY INFORMATION RECONSTRUCTION OF 3-D PATTERN OF KRT5 STAINING WITH RESPECT TO BRONCHIOLES (BLUE)
IN PORTION OF 15DPI INFECTED LUNG FROM SERIAL SECTIONS. Reconstruction of 3-D pattern of Krt5 staining with respect to bronchioles (blue) in portion of 15dpi infected lung from serial
sections. (MP4 27357 kb) POWERPOINT SLIDES POWERPOINT SLIDE FOR FIG. 1 POWERPOINT SLIDE FOR FIG. 2 POWERPOINT SLIDE FOR FIG. 3 POWERPOINT SLIDE FOR FIG. 4 RIGHTS AND PERMISSIONS Reprints and
permissions ABOUT THIS ARTICLE CITE THIS ARTICLE Zuo, W., Zhang, T., Wu, D. _et al._ p63+Krt5+ distal airway stem cells are essential for lung regeneration. _Nature_ 517, 616–620 (2015).
https://doi.org/10.1038/nature13903 Download citation * Received: 30 March 2014 * Accepted: 30 September 2014 * Published: 12 November 2014 * Issue Date: 29 January 2015 * DOI:
https://doi.org/10.1038/nature13903 SHARE THIS ARTICLE Anyone you share the following link with will be able to read this content: Get shareable link Sorry, a shareable link is not currently
available for this article. Copy to clipboard Provided by the Springer Nature SharedIt content-sharing initiative