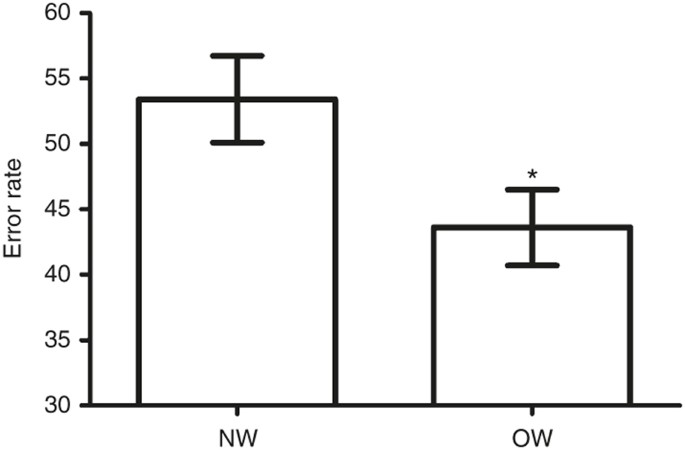
Potential effects of reward and loss avoidance in overweight adolescents
- Select a language for the TTS:
- UK English Female
- UK English Male
- US English Female
- US English Male
- Australian Female
- Australian Male
- Language selected: (auto detect) - EN
Play all audios:

ABSTRACT BACKGROUND: Reward system and inhibitory control are brain functions that exert an influence on eating behavior regulation. We studied the differences in inhibitory control and
sensitivity to reward and loss avoidance between overweight/obese and normal-weight adolescents. METHODS: We assessed 51 overweight/obese and 52 normal-weight 15-y-old Chilean adolescents.
The groups were similar regarding sex and intelligence quotient. Using Antisaccade and Incentive tasks, we evaluated inhibitory control and the effect of incentive trials (neutral, loss
avoidance, and reward) on generating correct and incorrect responses (latency and error rate). RESULTS: Compared to normal-weight group participants, overweight/obese adolescents showed
shorter latency for incorrect antisaccade responses (186.0 (95% CI: 176.8–195.2) vs. 201.3 ms (95% CI: 191.2–211.5), _P_ < 0.05) and better performance reflected by lower error rate in
incentive trials (43.6 (95% CI: 37.8–49.4) vs. 53.4% (95% CI: 46.8–60.0), _P_ < 0.05). Overweight/obese adolescents were more accurate on loss avoidance (40.9 (95% CI: 33.5–47.7) vs.
49.8% (95% CI: 43.0–55.1), _P_ < 0.05) and reward (41.0 (95% CI: 34.5–47.5) vs. 49.8% (95% CI: 43.0–55.1), _P_ < 0.05) compared to neutral trials. CONCLUSION: Overweight/obese
adolescents showed shorter latency for incorrect responses and greater accuracy in reward and loss avoidance trials. These findings could suggest that an imbalance of inhibition and reward
systems influence their eating behavior. SIMILAR CONTENT BEING VIEWED BY OTHERS ON THE EFFECTS OF IMPULSIVITY AND COMPULSIVITY ON NEURAL CORRELATES OF MODEL-BASED PERFORMANCE Article Open
access 10 September 2024 FOOD-RELATED IMPULSIVITY ASSESSED BY LONGITUDINAL LABORATORY TASKS IS REDUCED IN PATIENTS WITH BINGE EATING DISORDER IN A RANDOMIZED CONTROLLED TRIAL Article Open
access 15 April 2021 EATING DISORDER SYMPTOMS AND EMOTIONAL AROUSAL MODULATE FOOD BIASES DURING REWARD LEARNING IN FEMALES Article Open access 26 March 2025 MAIN There is increasing
appreciation of the complex neurobiology of obesity in which cognitive and motivational processes interact in appetitive behaviors. A recent model of obesity proposes that overeating
reflects an imbalance between neural circuits related to motivating behavior (reward mechanism) and prepotent response inhibition (1). Inhibitory control is the ability to voluntarily
inhibit dominant, automatic, prepotent, or incompatible responses in favor of a planned response (2). It is an aspect of executive function that is largely mediated by prefrontal cortical
function (orbitofrontal cortex and anterior cingulate gyrus) and modulated by the dopaminergic system (3). Inhibitory control is required for suppressing inappropriate/unwanted actions that
can interfere with attaining motor, cognitive, or socioemotional goals. It is involved in critical everyday tasks, from preventing impulsive actions to controlling the temptation to overeat
(4). Motivation for action also plays a crucial role in executive behavior. Motivation is influenced by reward processes, with complex brain circuits that involve the orbitofrontal cortex,
medial prefrontal cortex, insula, basal ganglia, hippocampus and amygdale, and dopaminergic systems in the midbrain (5). Rewards are stimuli such as objects or events that generate approach
to consummatory behavior, learning, positive outcomes, emotions, and hedonic feelings (6). The mesolimbic dopamine reward system plays a key role in giving incentive salience to preferred
food (7). Thus, reward-driven decision-making may play an important role in overeating behavior (8). For instance, loss of eating control is associated with more body fat gain over time (9)
and illicit drug use (10). Both inhibition and the assessment of potential rewards are crucial to decision-making (11). The model of neural circuit imbalance in obesity indicates that, in
vulnerable individuals, a high intake of high-calorie food could alter the ongoing balance between reward and inhibition circuits, resulting in an enhanced reinforcing value of food and a
relative weakening of cognitive control modulation. The disrupted balance would thus be a consequence of the resetting of reward thresholds and the weakening of the cortical top-down
circuits that regulate inhibition capacity, resulting in impulsive and compulsive food intake (12). There is neuroimaging support for this model. Overweight individuals who were presented
with pictures of high-calorie food showed increased neural activation of regions involved in reward circuits compared to normal weight (NW) controls (5). Our focus is on adolescence, a
period characterized by the hypersensitivity to potential rewards and immature cognitive control (13). This combination influences the decision-making process and favors involvement in risk
behaviors (11). Only a few studies have examined the association between inhibitory control, reward, and overweight/obesity (OW) in adolescents (14,15,16). Existing research for this age
group suggests that OW adolescents had poorer performance on response inhibition, cognitive flexibility, decision-making, and lower sensitivity to reward compared to NW adolescents (14,15).
Further, BMI showed a positive association with reward sensitivity in normal weight and overweight subjects that changed to a negative association in the obese group (16). Adolescents may
also be particularly sensitive to “motivation” from potential loss (17), but avoidance of loss has received even less attention than response to reward. OW has become a major public health
problem in Chile, with the prevalence in children and adolescents (females 27.1%, males 28.6%) among the highest levels in Latin America (18). In the current study, we assessed inhibitory
control and sensitivity to reward and loss avoidance in OW and NW Chilean adolescents. We hypothesized that OW adolescents would demonstrate lower inhibitory control and greater sensitivity
to reward and loss avoidance than NW adolescents. RESULTS The OW and NW groups were similar in background characteristics in infancy and childhood, although there was a tendency toward
higher self-reported prepregnancy maternal weight in the OW compared to NW group ( TABLE 1 ). By design, groups differed in anthropometric measurements in adolescence. LATENCY In the
Antisaccade task, OW adolescents showed shorter latencies to incorrect saccades than NW adolescents (_F_(1,102)=4.9, _P_ < 0.05) ( TABLE 2 ). There were no differences in latencies to
correct saccades (all _P_ > 0.714). In the Incentive task, there was no significant effect of group on latencies (_P_ > 0.289), nor was the interaction between group and trial type
significant for latencies (_P_ > 0.231). ACCURACY Considering all subjects in the Incentive task, there was a significant main effect of trial type (_F_(2,172) = 4.2, _P_ < 0.05).
Compared to neutral trials, reward trials (44.1 vs. 54.0%, _P_ < 0.01) and loss avoidance trials (46.6 vs. 54.0%, _P_ < 0.01) error rates were lower. There was a significant effect of
group on error rate (_F_(1,86) = 4.3, _P_ < 0.05). Overall, OW participants had a lower error rate than NW participants ( FIGURE 1 ). This result in OW adolescents was driven by greater
accuracy in neutral and loss avoidance trials compared to the NW group ( FIGURE 2 ). When we analyzed separately the accuracy of each group, we found that OW adolescents showed lower error
rate in loss avoidance (_t_ = −2.4, _P_ < 0.01) and reward trials (_t_ = −2.2, _P_ < 0.05) compared to neutral trials, but accuracy was similar in loss avoidance and reward trials (_t_
= 0.7, _P_ = 0.709) ( FIGURE 2 ). NW subjects also showed a lower error rate in loss avoidance (_t_ = −1.5, _P_ = 0.05) and reward trials (_t_ = −3.1, _P_ < 0.01) compared to neutral
trials. In contrast to the OW group, the NW group error rates differed between loss avoidance and reward trials, being more accurate in the reward trials (_t_ = −2.0, _P_ < 0.05) ( FIGURE
2 ). DISCUSSION This study highlights the association between inhibitory control and OW and contributes to the understanding of the impact of reward and loss avoidance in OW adolescents.
Few studies have determined individual differences in inhibition and the modulation of reward and loss avoidance in OW adolescents (14,15,16,19). We found that OW adolescents had shorter
latency for incorrect saccades in inhibitory task and lower error rate in incentive task than NW adolescents. Short-latency saccades have been associated with higher tendency to make
inhibitory errors, suggesting deficits in the ability to inhibit eye-movement responses to distracting stimuli (20). When OW adolescents commit errors, they have shorter latencies suggesting
that when inhibition fails it may be due to greater impulsivity (20). Regarding error rate compared to the NW group, OW adolescents had better accuracy across incentive trials. This greater
performance in the OW group could reflect increased sensitivity to incentive trials leading to better performance. It might be possible that OW adolescents were already performing the task
at a high level during neutral trials and they were unable to perceive neutral trials as stimuli that did not involve a reward value, discerning these trials more “rewarding” (21) and
feeling motivated to do it better than NW subjects. While neutral trials did not carry an immediate incentive contingency, they were embedded within an Incentive task that may engage the
reward system (21). Studies using this task have shown that adolescents have worse Antisaccade performance than adults (22) but can perform at adult levels when incentives are added (17).
Adolescents showed greater activity in striatal function in parallel with increased recruitment of inhibitory control regions during rewarded trials relative to adults, suggesting a specific
profile of reward processing in adolescence that may underlie their ability to perform at adult levels when incentives are present (21,23). Within groups, both OW and NW participants were
more efficient in reward and loss avoidance compared to their corresponding neutral trials. However, NW subjects had lower performance in loss avoidance than reward trials, while OW
adolescents did not. This may reflect enhanced response for loss avoidance. Such a bias has been related to emotional internal conflicts (19), impaired outcome monitoring, inability to learn
from experiences, and alterations in motivation (24). The possible mechanisms underlying the relationship between OW, inhibition, and the modulation of potential rewards and losses are not
fully understood. Studies in youth and adults suggest decreased dopamine signaling (receptors and release) in striatal regions, which are linked to reward and habits and also to routines in
obesity (25). Diminished striatal dopamine receptors have been associated to altered metabolic activity in both orbitofrontal cortex and anterior cingulate gyrus in obese humans (26). These
brain regions contribute to inhibitory control, and dysfunction relates to compulsive behaviors (26) Compared to NW subjects, OW individuals exhibited gray matter volume reductions in the
orbitofrontal cortex, postcentral and middle frontal gyrus (27,28). An association between higher BMI and lower metabolic activity in the dorsolateral prefrontal and cingulate brain regions
has also been reported (29). Obese subjects showing less activation of reward circuits were at increased risk for weight gain if they carried genetic risk for reduced dopamine signaling
(presence of dopamine D2 receptor gene allele). However, an increased activation in these brain regions predicted higher weight gain in subjects who did not have such a genetic risk factor
(30). Thus, OW subjects could have differences in brain circuits according to genetic factors that could make them vulnerable to overeating. Emerging evidence suggests that obesity and
addiction share the feature that individuals can express a desire to limit drugs or food consumption but persist despite knowing negative consequences (31). The ability to inhibit the urge
to eat desirable food varies among individuals and appears to be a factor that counteracts their vulnerability for overeating (32). Behavioral and neurobiological features described in obese
subjects, which are similar to patterns that characterize illicit drugs consumers (33), could implicate neuroadaptations in dopamine signaling as contributors to the disrupted functioning
of frontal cortical regions associated with OW (12). Overall, our data suggest differences in the inhibition process and reward response between OW and NW adolescents. However, we cannot
make inferences about causality given the cross-sectional nature of this study. Further, it might be important to use additional measures to determine nutritional status, like body
composition or biochemical indices. In summary, our results provide evidence of weaker inhibitory control and greater motivational sensitivity in OW individuals. They may be particularly
sensitive to incentives, affecting decision making and playing an important role in overeating behavior (7). In studying adolescents’ behaviors, it is important to understand the neural
circuits involved in the way that environmental stimuli influence decisions and actions. Such understanding should help inform strategies and interventions that aim to modify lifestyles
toward healthier behaviors. Our findings support the obesity-risk model proposing that overeating reflects an imbalance between circuits inhibiting prepotent responses and circuits of reward
(1). METHODS SUBJECTS The study included 103 adolescents who had neurophysiological evaluations in the Sleep and Functional Neurobiology Laboratory, INTA, University of Chile, at 15–16 y of
age. All were participants in an ongoing longitudinal study of the behavioral and developmental effects of iron-deficiency anemia in infancy. Detailed descriptions of the population and
study design (34) and findings during infancy and childhood have been published elsewhere (35,36). In brief, inclusion criteria for enrollment at the infancy phase of the study were healthy
full-term birth, birth weight ≥3.0 kg, without perinatal complications, and absence of acute or chronic illnesses. Infants with iron-deficiency anemia at 6, 12, or 18 mo were considered for
neurophysiological evaluations. Randomly chosen infants who were clearly nonanemic (venous Hb ≥ 115 g/l) also received evaluations. Participants were treated with oral iron for at least 6 mo
and had normal hemoglobin concentrations after treatment. No participant had iron-deficiency anemia at subsequent follow-ups. Parents provided signed informed consent, and adolescents gave
their written assent. The original and follow-up protocols were approved and reviewed annually by the Institutional Review Boards of the University of Michigan, Ann Arbor, and Institute of
Nutrition and Food Technology (INTA), University of Chile, Santiago. MATERIALS We used the Antisaccade task, which is a refined test of inhibitory control that has been well characterized in
animal and human studies (37) and quite widely used in the adolescent research field (2,17,21,23). To examine the effects of incentive types on response inhibition, we also used an
Incentive task with potential reward, loss avoidance, and neutral trials (17). Saccades are rapid eye movements that allow visual stimulus to be foveated and become the new target of
attention (17). Eye movements or saccades in these tasks were assessed with an eye-tracking system (Eye-Trac 6; Applied Science Laboratories, Bedford, MA). This system uses a corneal
reflection method with bright pupil technology. The point-of-gaze is determined by relating the corneal reflection of an infrared beam, which is projected to the eye to the center of the
illuminated pupil rotating with each eye movement. Stimuli were presented using E-Prime software (Psychology Software Tools, Pittsburgh, PA) and displayed on a computer monitor in front of
the participant. PROCEDURE Participants were in a darkened room facing the stimulus monitor, seated comfortably 60 cm away from the monitor center. At the beginning of the experimental
session, a 9-point calibration procedure was performed. Before each task, carefully standardized instructions were provided. Testing began after participants demonstrated understanding of
procedures. _Antisaccade task._ This task probes the ability to exert cognitive control of behavior by exerting voluntary suppression of a prepotent saccadic response. Subjects must inhibit
an eye movement towards a visual stimulus and instead make a planned movement to its mirror location. In this task participants were required to fixate a central stimulus for 0.5 to 6.0 s,
after which a peripheral target appeared for 1.0 s at one of four locations randomly presented (4 or 8 degrees to the left or right of center fixation). Adolescents were instructed to look
in the opposite location of the peripheral target (whose location was unpredictable) as quickly and accurately as possible. Forty-eight experimental trials were presented. _Incentive task._
This task explores if the presence of trials with incentives of “reward,” “loss avoidance,” or “neutral” alters task performance (17). The saccade component is the same as described above,
but in this task each trial began with 2- or 3-s presentation of one of three possible incentive trials: * (i) Reward trial: A computer image of a bill of one thousand Chilean pesos (1.71
USD) indicated a monetary gain if he/she performed the trial correctly. An incorrect response did not result in “money loss”. * (ii) Loss avoidance trial: A torn bill of one thousand Chilean
pesos indicated a monetary loss if an error was made. Correct response did not result in “money gain”. * (iii) Neutral trial: A green rectangle indicated no incentive, i.e., no money was
“gained” or “lost” and the amount of money remained the same, regardless of performance. Following the fixation cue, indicating that an antisaccade had to be subsequently performed, a
peripheral target appeared for 1.0 s at one of six locations (9, 6, or 3 degrees to the left or right of the center fixation). Finally, a central stimulus appeared for 1.0 s to center gaze
before the next trial. The protocol included 20 reward trials, 20 loss avoidance trials, and 20 neutral trials, presented in random order. Participants were encouraged to perform the task as
well as they could regardless of incentive trial and as quickly as possible. The first eye movement with velocity ≥ 30º/s was classified as a correct or incorrect response. Variables of
interest included correct saccade (movement made toward the opposite visual field of the peripheral target) and incorrect saccade (movement made toward the peripheral target). Latencies to
initiate responses and error rates were also obtained for each trial type (error rate = (number of errors/number of opportunities) *100). Performance on the Incentive task was evaluated
comparing error rate and latencies by group and trial type. The effect of reward and loss avoidance trials compared to neutral trials in each group was interpreted as the modulation of
reward and loss avoidance in inhibitory control. EYE-TRACKING DATA PROCESSING Eye movement data were scored off-line using ILAB software (Northwestern University Medical School and V. A.
Healthcare System, Chicago, IL) (38) and MATLAB (MathWorks, Natick, MA), which calculated the direction, latency, and accuracy of saccades. Eye movement latencies < 70 ms were excluded in
the analysis. Before final classification of saccades, performance on each trial was checked to identify blink artifacts and occasional failures of the software that detected saccades.
ANTHROPOMETRIC MEASURES Trained personnel made weight and height measurements using standardized techniques (without shoes, wearing underwear, and in the Frankfurt position) on the same
machine calibrated every day. Weight to the closest 0.1 kg and height to the closest 0.1 cm were measured using a SECA scale (model 700, Seca, Hamburg, Germany). BMI was calculated for each
participant as the ratio of weight in kilograms divided by the square of height in meters. Sex-and age-specific BMI percentiles and z-scores were calculated and categorized according to the
cut-off points recommended by the World Health Organization (39) as NW (BMI z-score ≥ −2 to < 1) and OW (BMI z-score ≥ 1, i.e., overweight and obese). DATA ANALYSIS We used multivariate
ANOVA to assess the relationship between group (NW and OW) and performance (error rate and latencies) on the Antisaccade task. We conducted repeated measures ANOVA to examine the main
effects and interaction of performance on the Incentive task and group (NW and OW). The within-participants factor was trial type (reward, loss avoidance, and neutral), and the
between-participants factor was group. _Post-hoc_ paired _t_-tests were conducted using Bonferroni correction for multiple comparisons. The model included sex, iron status in infancy, and
socioeconomic status as covariates. Statistical analyses were conducted with SPSS software version 19.0 (SPSS, Chicago, IL). All significance tests were two-tailed; a _P_ value < 0.05 was
considered statistically significant. STATEMENT OF FINANCIAL SUPPORT This study was supported by Chilean National Fund for Scientific and Technological Development grant 1110513, and US
National Institutes of Health (Bethesda, MD) grant HD33487. DISCLOSURES All authors have nothing to disclose. REFERENCES * Volkow ND, Wang GJ, Fowler JS, Telang F. Overlapping neuronal
circuits in addiction and obesity: evidence of systems pathology. _Philos Trans R Soc Lond, B, Biol Sci_ 2008;363:3191–200. Article Google Scholar * Ordaz SJ, Foran W, Velanova K, Luna B.
Longitudinal growth curves of brain function underlying inhibitory control through adolescence. _J Neurosci_ 2013;33:18109–24. Article CAS Google Scholar * Logue SF, Gould TJ. The neural
and genetic basis of executive function: attention, cognitive flexibility, and response inhibition. _Pharmacol Biochem Behav_ 2014;123:45–54. Article CAS Google Scholar * Mostofsky SH,
Simmonds DJ. Response inhibition and response selection: two sides of the same coin. _J Cogn Neurosci_ 2008;20:751–61. Article Google Scholar * Stoeckel LE, Kim J, Weller RE, Cox JE, Cook
EW 3rd, Horwitz B. Effective connectivity of a reward network in obese women. _Brain Res Bull_ 2009;79:388–95. Article Google Scholar * Schultz W. Dopamine signals for reward value and
risk: basic and recent data. _Behav Brain Funct_ 2010;6:24. Article Google Scholar * Berridge KC, Ho CY, Richard JM, DiFeliceantonio AG. The tempted brain eats: pleasure and desire
circuits in obesity and eating disorders. _Brain Res_ 2010;1350:43–64. Article CAS Google Scholar * Pignatti R, Bertella L, Albani G, Mauro A, Molinari E, Semenza C. Decision-making in
obesity: a study using the Gambling Task. _Eat Weight Disord_ 2006;11:126–32. Article CAS Google Scholar * Tanofsky-Kraff M, Cohen ML, Yanovski SZ, et al. A prospective study of
psychological predictors of body fat gain among children at high risk for adult obesity. _Pediatrics_ 2006;117:1203–9. Article Google Scholar * Ross HE, Ivis F. Binge eating and substance
use among male and female adolescents. _Int J Eat Disord_ 1999;26:245–60. Article CAS Google Scholar * Blakemore SJ, Robbins TW. Decision-making in the adolescent brain. _Nat Neurosci_
2012;15:1184–91. Article CAS Google Scholar * Volkow ND, Wang GJ, Baler RD. Reward, dopamine and the control of food intake: implications for obesity. _Trends Cogn Sci (Regul Ed)_
2011;15:37–46. Article CAS Google Scholar * Naneix F, Marchand AR, Pichon A, Pape JR, Coutureau E. Adolescent stimulation of D2 receptors alters the maturation of dopamine-dependent
goal-directed behavior. _Neuropsychopharmacology_ 2013;3:1–9. Google Scholar * Verdejo-García A, Pérez-Expósito M, Schmidt-Río-Valle J, et al. Selective alterations within executive
functions in adolescents with excess weight. _Obesity (Silver Spring)_ 2010;18:1572–8. Article Google Scholar * Delgado-Rico E, Río-Valle JS, González-Jiménez E, Campoy C, Verdejo-García
A. BMI predicts emotion-driven impulsivity and cognitive inflexibility in adolescents with excess weight. _Obesity (Silver Spring)_ 2012;20:1604–10. Article Google Scholar * Verbeken S,
Braet C, Lammertyn J, Goossens L, Moens E. How is reward sensitivity related to bodyweight in children? _Appetite_ 2012;58:478–83. Article Google Scholar * Geier CF, Luna B. Developmental
effects of incentives on response inhibition. _Child Dev_ 2012;83:1262–74. Article Google Scholar * Rodríguez L. Situación nutricional del escolar y adolescente en Chile. _Rev Chil
Pediatr_ 2007;78:523–33. Google Scholar * Matton A, Goossens L, Braet C, Vervaet M. Punishment and reward sensitivity: are naturally occurring clusters in these traits related to eating and
weight problems in adolescents? _Eur Eat Disord Rev_ 2013;21:184–94. Article Google Scholar * Luna B, Velanova K, Geier CF. Development of eye-movement control. _Brain Cogn_
2008;68:293–308. Article Google Scholar * Geier CF, Terwilliger R, Teslovich T, Velanova K, Luna B. Immaturities in reward processing and its influence on inhibitory control in
adolescence. _Cereb Cortex_ 2010;20:1613–29. Article CAS Google Scholar * Luna B. Developmental changes in cognitive control through adolescence. _Adv Child Dev Behav_ 2009;37:233–78.
Article Google Scholar * Padmanabhan A, Geier CF, Ordaz SJ, Teslovich T, Luna B. Developmental changes in brain function underlying the influence of reward processing on inhibitory
control. _Dev Cogn Neurosci_ 2011;1:517–29. Article Google Scholar * Bischoff-Grethe A, McCurdy D, Grenesko-Stevens E, et al. Altered brain response to reward and punishment in adolescents
with Anorexia nervosa. _Psychiatry Res_ 2013;214:331–40. Article Google Scholar * Wang GJ, Volkow ND, Logan J, et al. Brain dopamine and obesity. _Lancet_ 2001;357:354–7. Article CAS
Google Scholar * Volkow ND, Wang GJ, Telang F, et al. Low dopamine striatal D2 receptors are associated with prefrontal metabolism in obese subjects: possible contributing factors.
_Neuroimage_ 2008;42:1537–43. Article Google Scholar * Yokum S, Ng J, Stice E. Relation of regional gray and white matter volumes to current BMI and future increases in BMI: a prospective
MRI study. _Int J Obes (Lond)_ 2012;36:656–64. Article CAS Google Scholar * Maayan L, Hoogendoorn C, Sweat V, Convit A. Disinhibited eating in obese adolescents is associated with
orbitofrontal volume reductions and executive dysfunction. _Obesity (Silver Spring)_ 2011;19:1382–7. Article Google Scholar * Volkow ND, Wang GJ, Telang F, et al. Inverse association
between BMI and prefrontal metabolic activity in healthy adults. _Obesity (Silver Spring)_ 2009;17:60–5. Article Google Scholar * Stice E, Yokum S, Bohon C, Marti N, Smolen A. Reward
circuitry responsivity to food predicts future increases in body mass: moderating effects of DRD2 and DRD4. _Neuroimage_ 2010;50:1618–25. Article CAS Google Scholar * Fandiño J, Moreira
RO, Preissler C, et al. Impact of binge eating disorder in the psychopathological profile of obese women. _Compr Psychiatry_ 2010;51:110–4. Article Google Scholar * Wang GJ, Volkow ND,
Telang F, et al. Evidence of gender differences in the ability to inhibit brain activation elicited by food stimulation. _Proc Natl Acad Sci USA_ 2009;106:1249–54. Article CAS Google
Scholar * Lillis J, Levin ME, Trafton JA. Elevated BMI and illicit drug use are associated with decreased ability to inhibit prepotent behaviors. _Addict Behav_ 2012;37:544–7. Article
Google Scholar * Lozoff B, De Andraca I, Castillo M, Smith JB, Walter T, Pino P. Behavioral and developmental effects of preventing iron-deficiency anemia in healthy full-term infants.
_Pediatrics_ 2003;112:846–54. PubMed Google Scholar * Peirano P, Algarin C, Chamorro R, Manconi M, Lozoff B, Ferri R. Iron deficiency anemia in infancy exerts long-term effects on the
tibialis anterior motor activity during sleep in childhood. _Sleep Med_ 2012;13:1006–12. Article Google Scholar * Algarín C, Nelson CA, Peirano P, Westerlund A, Reyes S, Lozoff B.
Iron-deficiency anemia in infancy and poorer cognitive inhibitory control at age 10 years. _Dev Med Child Neurol_ 2013;55:453–8. Article Google Scholar * Munoz DP, Everling S. Look away:
the anti-saccade task and the voluntary control of eye movement. _Nat Rev Neurosci_ 2004;5:218–28. Article CAS Google Scholar * Gitelman DR. ILAB: a program for postexperimental eye
movement analysis. _Behav Res Methods Instrum Comput_ 2002;34:605–12. Article Google Scholar * World Health Organization. Growth reference 5-19 years, 2007.
http://www.who.int/growthref/who2007_bmi_for_age/en/. * Kang Sim DE, Cappiello M, Castillo M, et al. Postnatal Growth Patterns in a Chilean Cohort: The Role of SES and Family Environment.
_Int J Pediatr_ 2012;2012:354060. Article CAS Google Scholar Download references ACKNOWLEDGEMENTS We would like to express our gratitude to the adolescents and parents whose participation
made this study possible. We also thank the technicians of the Sleep and Functional Neurobiology Laboratory of INTA, University of Chile, who contributed during the course of this study,
and drivers for providing careful transportation services to participants. AUTHOR INFORMATION AUTHORS AND AFFILIATIONS * Sleep and Functional Neurobiology Laboratory, Institute of Nutrition
and Food Technology (INTA), University of Chile, Santiago, Chile Sussanne Reyes, Patricio Peirano & Cecilia Algarín * Laboratory of Neurocognitive Development, Western Psychiatric
Institute and Clinic, University of Pittsburgh, Pittsburgh, Pennsylvania Beatriz Luna * Center for Human Growth and Development, University of Michigan, Ann Arbor, Michigan Betsy Lozoff
Authors * Sussanne Reyes View author publications You can also search for this author inPubMed Google Scholar * Patricio Peirano View author publications You can also search for this author
inPubMed Google Scholar * Beatriz Luna View author publications You can also search for this author inPubMed Google Scholar * Betsy Lozoff View author publications You can also search for
this author inPubMed Google Scholar * Cecilia Algarín View author publications You can also search for this author inPubMed Google Scholar CORRESPONDING AUTHOR Correspondence to Cecilia
Algarín. POWERPOINT SLIDES POWERPOINT SLIDE FOR FIG. 1 POWERPOINT SLIDE FOR FIG. 2 RIGHTS AND PERMISSIONS Reprints and permissions ABOUT THIS ARTICLE CITE THIS ARTICLE Reyes, S., Peirano,
P., Luna, B. _et al._ Potential effects of reward and loss avoidance in overweight adolescents. _Pediatr Res_ 78, 152–157 (2015). https://doi.org/10.1038/pr.2015.82 Download citation *
Received: 02 September 2014 * Accepted: 21 January 2015 * Published: 30 April 2015 * Issue Date: August 2015 * DOI: https://doi.org/10.1038/pr.2015.82 SHARE THIS ARTICLE Anyone you share the
following link with will be able to read this content: Get shareable link Sorry, a shareable link is not currently available for this article. Copy to clipboard Provided by the Springer
Nature SharedIt content-sharing initiative