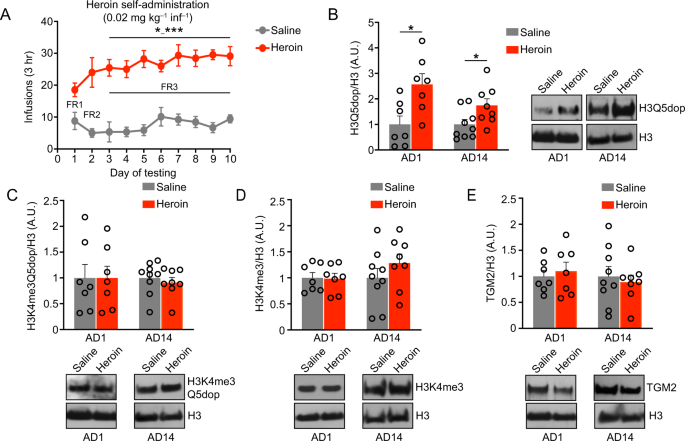
Histone h3 dopaminylation in ventral tegmental area underlies heroin-induced transcriptional and behavioral plasticity in male rats
- Select a language for the TTS:
- UK English Female
- UK English Male
- US English Female
- US English Male
- Australian Female
- Australian Male
- Language selected: (auto detect) - EN
Play all audios:

ABSTRACT Persistent transcriptional events in ventral tegmental area (VTA) and other reward relevant brain regions contribute to enduring behavioral adaptations that characterize substance
use disorder. Recent data from our laboratory indicate that aberrant accumulation of the newly discovered histone post-translational modification (PTM), H3 dopaminylation at glutamine 5
(H3Q5dop), contributes significantly to cocaine-seeking behavior following prolonged periods of abstinence. It remained unclear, however, whether this modification is important for relapse
vulnerability in the context of other drugs of abuse, such as opioids. Here, we showed that H3Q5dop plays a critical role in heroin-mediated transcriptional plasticity in midbrain regions,
particularly the VTA. In rats undergoing abstinence from heroin self-administration (SA), we found acute and persistent accumulation of H3Q5dop in VTA. Attenuation of H3Q5dop during
abstinence induced persistent changes in gene expression programs associated with neuronal signaling and dopaminergic function in heroin abstinence and led to reduced heroin-seeking
behavior. Interestingly, the observed changes in molecular pathways after heroin SA showed significant yet reversed overlap with the same genes altered in cocaine SA. These findings
establish an essential role for H3Q5dop, and its downstream transcriptional consequences, in heroin-induced functional plasticity in VTA. SIMILAR CONTENT BEING VIEWED BY OTHERS TRNA
EPITRANSCRIPTOMIC ALTERATIONS ASSOCIATED WITH OPIOID-INDUCED REWARD-SEEKING AND LONG-TERM OPIOID WITHDRAWAL IN MALE MICE Article Open access 08 February 2024 REGULATION OF DOPAMINE-DEPENDENT
TRANSCRIPTION AND COCAINE ACTION BY _GADD45B_ Article Open access 14 September 2020 COCAINE INDUCES PARADIGM-SPECIFIC CHANGES TO THE TRANSCRIPTOME WITHIN THE VENTRAL TEGMENTAL AREA Article
Open access 21 June 2021 INTRODUCTION Opioid Use Disorder (OUD) has dramatically increased to epidemic proportions in recent years, with heroin dependence growing more than 50% over the last
decade and accounting for more drug-related deaths [1] than any other substance of abuse [2,3,4,5]. Clinically, OUD is characterized by compulsive drug-seeking behaviors that can persist
for years and extend over the course of a lifetime, with one of the highest risks of relapse among substance use disorders [1,2,3,4,5]. Relapse vulnerability is a key diagnostic feature of
addiction, and represents a highly complex process involving multiple circuits mediated by dynamic alterations in cellular plasticity within the mesolimbic reward system, where aberrant
dopamine neurotransmission promotes maladaptive reward-processing and persistent deficits in motivated behaviors. Given the recurring, long-lasting nature of OUD, the field has begun to
place great emphasis on drug-induced epigenetic regulation as a potential key mechanism in opioid addiction and relapse [6,7,8,9,10]. To date, however, our understanding of the causal
mechanisms that mediate drug-induced transcriptional and cellular plasticity remains incomplete, particularly in the relatively understudied ventral tegmental area (VTA), which contains
critical dopaminergic projections to reward-processing striatal regions. Recently, our laboratory demonstrated that in addition to modulating downstream synaptic activity as a
neurotransmitter, dopamine itself plays a direct role in epigenetically coordinating gene expression within these dopaminergic cells in the VTA [11]. We previously found that certain
monoamine neurotransmitters, including dopamine, can serve as donors for covalent modifications on specific residues of substrate proteins (termed monoaminylations) through an enzymatic
process mediated by the writer-protein Transglutaminase 2 (TGM2) [12]. Among the identified substrate proteins for monoaminylation is histone H3, which is modified at glutamine 5 (Q5) on its
N-terminal tail. At this H3Q5 site, specific histone monoaminylation marks (e.g., serotonylation or dopaminylation) were found to alter gene expression, either directly or through
combinatorial interactions with other nearby histone PTMs, suggesting that these modifications may constitute an important epigenetic regulatory mechanism in monoaminergic neuromodulation
[12, 13]. In a recent report, we confirmed that histone H3 dopaminylation (H3Q5dop) has a key functional role in cocaine-mediated transcriptional plasticity, dopaminergic neurotransmission,
and cocaine-seeking behavior in a rodent model of drug relapse vulnerability [11]. Given the importance of H3Q5dop in mediating cocaine-induced transcriptional and behavioral plasticity,
here we investigated whether functional regulation by H3Q5dop represents an important mechanism in dependence for other addictive substances such as heroin, or if this phenomenon was
specific to cocaine. All drugs of abuse are thought to elicit changes in dopaminergic neurotransmission from VTA, although different classes of substances act on this system through distinct
cellular mechanisms. In addition, the magnitude and temporal dynamics of dopamine transmission vary widely between drugs, even within class, highlighting the importance of delineating
substance-specific mechanisms in order to develop targeted therapeutic strategies to address the molecular changes associated with each drug [14]. In the present study, we investigated
specific roles for H3Q5dop in heroin-induced VTA transcriptional plasticity and drug-seeking behavior in a rodent model of relapse vulnerability. We found that abstinence from heroin SA
resulted in the accumulation of H3Q5dop in VTA, though this increase occurs more rapidly in response to heroin vs. cocaine abstinence. We further showed that aberrant H3Q5dop dynamics in VTA
contribute significantly to heroin-induced gene expression and drug-seeking behaviors. These findings indicate that H3Q5dop may represent a conserved epigenetic substrate underlying
addiction to distinct classes of abused drugs (e.g., cocaine and heroin), though the regulation of gene expression programs that lead to altered DA output from VTA may be specific to
heroin’s mechanism of action. MATERIALS AND METHODS ANIMALS Male Sprague-Dawley rats (Envigo; 250–300 g) were singly housed during self-administration experimental procedures, and maintained
on a 12 h reverse light/dark cycle with food and water available _ad libitum_. All procedures were done in accordance with NIH guidelines and the Institutional Animal Care and Use
Committees of the Icahn School of Medicine at Mount Sinai and the State University of New York at Buffalo. JUGULAR CATHETERIZATION For both heroin SA and saline SA, rats were implanted with
chronic indwelling jugular catheters, as previously reported [15, 16]. Rats were given 5 d of recovery, during which time the catheters were flushed with 0.2 mL of a solution containing
enrofloxacin (4 mg/mL) and heparin saline (50 IU/mL in 0.9% sterile saline) to preserve catheter patency. All animals received an i.v. infusion of ketamine hydrochloride (0.5 mg/kg in 0.05
mL) one day prior to behavioral training to verify catheter patency. Rats that responded with a loss of muscle tone and righting reflexes were considered patent and were included in cohorts
for behavioral training. HEROIN SELF-ADMINISTRATION AND VIRAL SURGERIES Rats were allowed to recover from jugular catheterization surgery for 5 d, and then were trained to SA heroin (0.02
mg/kg/inf) or saline (as a non-reinforcement control) in operant chambers for 3 h each day for a period of 10 d. A response in the active hole nose poke resulted in an intravenous injection
of heroin (0.02 mg/kg/inf) or saline using a fixed ratio 1 (FR1) schedule of reinforcement on day 1 that was increased to FR3 on day 3 and maintained for the remainder of the experiment
[15]. Responses in the inactive hole resulted in no programmed consequences. Following training sessions, catheters were flushed, and rats were returned to their colony rooms. For viral
manipulations, all rats self-administered heroin or saline, and subsequently received intra-VTA bilateral injections of either AAV2-GFP (empty vector control), AAV2-H3.3WT, or AAV2-H3.3Q5A
on AD11 (see below for additional information on surgery coordinates). CUE-INDUCED SEEKING TEST For the cue-induced seeking behavior, on AD30, rats were returned to operant chambers for a
testing duration of 1 h. During the test duration, rats were subjected to extinction-like conditions whereby responses in an active hole delivered only the cue light previously associated
with drug availability. The total number of active responses in the active hole was an operational measure of heroin-seeking behavior [17, 18]. WESTERN BLOTTING AND ANTIBODIES VTA tissues
were collected from heroin SA rats (2 mm punches) on AD1, AD14 or AD30 and frozen. Nuclear fractions were purified via homogenization in Buffer A containing 10 mM HEPES (pH 7.9), 10 mM KCl,
1.5 mM MgCl2, 0.34 M sucrose, 10% glycerol, 1 mM EDTA, 1X protease inhibitor cocktail and 1X phosphostop inhibitor. After homogenization, 0.1% TritonX-100 was added, samples were incubated
on ice for 30 min and then centrifuged for 5 min at 1300 × _g_ at 4 °C. Supernatants (i.e., cytosolic fractions) were discarded, and nuclear pellets were resuspended in Buffer A to remove
any remaining cytosolic contamination, followed by centrifugation for 5 min at 1300 × _g_ at 4 °C. Supernatants were then discarded and pellets resuspended and sonicated in sample buffer
containing 0.3 M sucrose, 5 mM HEPES, 1% SDS and 1X protease inhibitor cocktail. Protein concentrations were measured with the DC protein assay kit (BioRad), and 10–15 ug of protein was
loaded onto 4–12% NuPage BisTris gels (Invitrogen) for electrophoresis. Proteins were next transferred to nitrocellulose membranes and blocked for 1 h in 5% milk in PBS + 0.1% Tween 20
(PBST), followed by primary antibody incubation for two days at 4 °C. The following antibodies were used in this manuscript: rabbit anti-H3Q5dop (1:200, ABE2588; Millipore), rabbit
anti-H3K4me3Q5dop (1:500, ABE2590; Millipore), rabbit anti-H3K4me3 (1:1000, lot #: GR273043-6, Abcam ab8580), mouse anti-Tgm2 (1:500, lot #: Gr3188112-4, Abcam ab2386) and rabbit anti-H3
(1:50000, lot #: GR293151-1, Abcam ab1791). Membranes were then washed 3X in PBST (10 min) and incubated for 1 h with horseradish peroxidase conjugated anti-rabbit (BioRad 170-6515, lot #:
64033820) or anti-mouse (GE Healthcare UK Limited NA931V, lot #: 9814763) secondary antibodies (1:10000; 1:50000 for anti-H3 antibody, BioRad) in 5% milk/PBST at RT. After three more washes
with PBST, bands were detected using enhanced chemiluminescence (ECL; Millipore). Densitometry was used to quantify protein bands using Image J Software (NIH), and proteins were normalized
to total H3. H3 measurements were obtained from the same membrane as the protein of interest after stripping the primary antibody for 10 min in Restore Stripping Buffer (Thermo #21059). AAV
CONSTRUCTS AND VIRAL INFUSION Adeno-associated virus H3.3 constructs [empty vs. wildtype (WT) vs. (Q5A)-Flag-HA] were generated and validated, as previously described [11, 12, 19]. All three
vectors contain a GFP fluorescent tag to allow visualization of the injection site during tissue dissection. The H3.3Q5A vector used in these studies, which does not affect adjacent
H3K4me3, inhibits the expression of all modifications on Q5. However, appreciable signal for other monoaminyl marks (e.g., H3Q5ser) is not observed in VTA vs. H3Q5dop [11]. After 24 h
following the last day of heroin vs. saline SA (day 11), animals were anaesthetized with a ketamine/xylazine solution (60/5 mg/kg) i.p., positioned in a stereotaxic frame (Kopf instruments)
and 1 μl of viral construct was infused bilaterally into VTA using the following coordinates; anterior-posterior (AP) −4.9, medial-lateral [20] +2.1, dorsal-ventral [21] −7.6. After surgery,
rats received meloxicam (1 mg/kg) s.c. and topical antibiotic treatments for ~3 days. Of note, previous reports comparing heroin cue-seeking behavior at AD14 vs. AD30 have found no
significant differences in heroin-seeking behavior between these two extended abstinence time points [22]. Furthermore, the present experiments demonstrated robust heroin-seeking behavior at
AD30 (see Fig. 3). Therefore, to ensure that we captured gene expression changes at a timepoint corresponding to maximal transgene expression from our AAV serotype, (which begins at 7 d
post-infusion and increases to maximal expression at 28d) all tissue collections or behavioral testing commenced 30 d after viral surgery to allow for maximal expression of the viral
constructs, as previously validated [11]. RNA ISOLATION AND SEQUENCING Rat brains were removed and immediately snap frozen. Brains were then sectioned to 100 uM thick coronal slices on a
cryostat at −20 °C, and 2 mm punches were dissected from virally infected VTA using GFP-fluorescence illuminated via a NIGHTSEA Dual Fluorescent Protein flashlight (#DFP-1). Total RNA was
then extracted from punches using Trizol (Thermo Fisher #15596026)-chloroform, followed by cleanup and elution into RNAase-free water using Qiagen minelute (#74204) columns, as per
manufacturer instructions. Following elution, RNA samples were enriched for mRNA via polyA tail selection beads, and mRNA libraries were prepared using the Illumina Truseq RNA Library Prep
Kit V2 (#RS-122-2001). Libraries were pooled and sequenced on the Illumina Novaseq platform. RNA-seq data were pre-processed and analyzed, as previously described [11]. Briefly, FastQC
(Version 0.72) was performed on the concatenated replicate raw sequencing reads from each library to ensure minimal PCR duplication and sequencing quality. Reads were aligned to the rn6
genome using HISAT2 (Version 2.1.0) and annotated against Ensembl v90. Multiple-aligned reads were removed, and remaining transcript reads were counted using featurecounts (Version 2.0.1).
Technical variation between samples and batch effects were calculated and normalized using the RUVr model of RUVseq (Version 4.1.1). DESEQ2 [23] (Version 2.11.40.6) was then used to
normalize read counts between the groups, and to perform pairwise differential expression analyses. Differentially expressed (DEx) genes were defined at FDR cutoff <0.05, Log2FC ± 0.2.
Threshold free Rank-Rank Hypergeometric Overlap (RRHO) maps were generated to visualize transcriptome-wide gene expression concordance patterns as previously described [24], using RRHO2
(Version 1.0). DE genes were overlapped (Venny) to identify the subset of DE genes reversed between heroin H3.3Q5A and both heroin empty and heroin H3.3 WT. Odds Ratio analyses were carried
out on DE gene lists using the _GeneOverlap_ R package version 1.26.0 [25]. Processed read count data for cocaine comparisons was extracted from our previous publication [11], deposited in
the National Center for Biotechnology Information Gene Expression Omnibus database under accession number GSE124055. In order to determine if differential gene expression comparisons showed
significant overlaps with annotated gene sets, we performed GO Enrichment Analyses using the EnrichR package (Version 3.0) against EnrichR’s gene set databases Panther Pathway, GO Biological
Process 2021, and GO Molecular Function 2021. Enrichments were ranked by adj. _p_ value [26], computed using Fisher’s exact test followed by Benjamini-Hochberg (BH) correction. Multivariant
plots display top ranked gene sets, Y-axis = rank from 1–5 for each term, plotted according to -log10(adj._p_ value) on the X-axis, and the combined score of the enrichment (bubble size).
The combined score is derived from both the _p_ value and the z-score (deviation from expected rank based on gene set size) calculated by the following formula: c = ln(p) * z. Fill color
represents the database source of terms. For Fig. S2 displaying gene expression changes in heroin vs. saline groups, we also implemented EnrichR’s gene set databases PanglaoDB Augmented
2021, which is a single-cell RNA-sequencing database for identifying cell-type enrichment, and GO Biological Process 2021. Enrichments were ranked by adj._p_ value [26]. Multivariant plots
display top ranked gene sets, Y-axis = rank from 1–5 for Cell-type and Gene ontology (GO BP) gene sets, plotted according to signed -log10(adj._p_ value) on the X-axis to indicate
directionality of expression of genes analyzed, and the number of DEx genes overlapping with the gene set (bubble size), and the comparison source of DEx genes (fill color). STATISTICAL
ANALYSES For western blot comparisons, Student’s _t_ tests were performed to compare saline vs. heroin conditions at different time points. For all behavioral testing involving more than two
treatments and time points, Two-way ANOVAs were performed with subsequent Dunnett’s (for heroin SA escalation) or Tukey’s post hoc analyses for multiple comparisons (MC) (as indicated
throughout the text). In molecular analyses, all animals used were included as separate _n_s (samples were not pooled). Significance was determined at _p_ < 0.05. All data are represented
as mean ± SEM. RESULTS H3Q5DOP IS INCREASED DURING BOTH ACUTE AND PROLONGED ABSTINENCE IN VTA FOLLOWING HEROIN SA Given that histone dopaminylation (H3Q5dop), but not combinatorial H3
lysine 4 (K4) trimethyl (me3) Q5dop, was previously found to accumulate in VTA during prolonged, but not acute, abstinence from cocaine SA [11], we hypothesized that chronic exposures to
heroin may similarly alter the deposition of H3Q5dop in this brain region. To assess this, we employed a heroin SA regimen wherein rats received i.v. infusions of heroin or saline (as a
non-reinforcement control) paired with a light cue (Fig. 1A) for a period of 10 d, during which they escalated their heroin intake (Two-Way ANOVA, effect of time x condition F [9, 144] =
2.573, _P_ = 0.0089, followed by Dunnett’s MC test for d1 vs. d5-d10; _p_ = 0.03-_p_ = 0.0008) [15]. Following SA, rats underwent forced abstinence in their home cages for 1 d vs. 14 d, the
latter of which represents a time period where cue-induced heroin seeking is expressed [27]. On abstinence day (AD) 1 or AD14, VTA tissues were collected and western blotting was performed
to quantify histone dopaminylation levels (both H3Q5dop and H3K4me3Q5dop), as well as expression of an associated histone PTM (e.g., H3K4me3) and the writer Transglutaminase 2 enzyme. We
found that H3Q5dop levels significantly increased (Fig. 1B) in VTA during acute abstinence (AD1) from heroin SA vs. saline SA, _via_ Student’s _t_ test (t = 2.952, df = 12, _p_ = 0.012).
This increase persisted to extended abstinence periods (AD14), via Student’s _t_ test (_t_ = 2.298, df = 15, _p_ = 0.03). Neither H3K4me3Q5dop nor total H3K4me3 (Fig. 1C, D) showed increases
at either time point (Student’s _t_ test, _p_ > 0.05). The writer enzyme for H3Q5dop was unaffected in its expression during periods of abstinence from heroin (Student’s _t_ test, _p_
> 0.05) (Fig. 1E). Together, these data indicate that accumulation of histone dopaminylation in VTA occurs during abstinence from heroin. Interestingly, heroin-induced accumulation of
H3Q5dop was found to occur more rapidly after opioid exposure vs. cocaine, with significant increases detected as soon as AD1. This increase persisted to AD14, suggesting that H3Q5dop may
contribute, at least in part, to the opioid-induced transcriptional and behavioral alterations underlying relapse-like behaviors associated with drug exposure. H3Q5DOP REGULATES HEROIN
ASSOCIATED GENE EXPRESSION PROGRAMS DURING PROLONGED ABSTINENCE To explore the functional consequences of H3Q5dop accumulation during heroin abstinence, we used an AAV2 vector that expresses
the H3 variant, H3.3, with a glutamine-to-alanine substitution at position 5 (H3.3Q5A), which cannot be dopaminylated. This H3.3Q5A construct has been shown to strongly express the mutant
H3.3Q5A protein in a nuclear-specific manner in adult neurons, resulting in downregulation of H3Q5dop [11]. Importantly, we have previously shown that viral expression of this H3.3Q5A mutant
prevents the accumulation of H3Q5dop observed after long-term abstinence from cocaine SA [11]. Therefore, in order to directly evaluate the impact of reducing H3Q5dop accumulation on gene
expression programs during prolonged abstinence from heroin, we delivered one of three AAV viral vectors: H3.3QA; H3.3 wildtype (WT); or an empty GFP control vector [11, 12] intra-VTA,
following heroin SA and a 30 d period of enforced abstinence (Fig. 2A), [11]. On AD30, infected VTA tissues were microdissected and processed for RNA-seq. We first utilized a threshold free
Rank-Rank Hypergeometric Overlap (RRHO) analysis [24] to characterize the relationship between gene expression patterns across the entire transcriptome for viral group comparisons (Fig. 2B).
RRHO revealed a significant overlap in genes that are upregulated in both heroin empty vs. saline empty and in heroin H3.3 WT vs. saline H3.3 WT (Fig. 2C top panel), indicating that these
two heroin SA conditions showed highly similar increases in gene expression compared to corresponding saline groups. In order to determine effects of the H3.3Q5A mutant in the context of
heroin, we plotted the transcriptome of heroin H3.3Q5a _vs_. both heroin empty and heroin H3.3 WT vectors (Fig. 2C_,_ middle and bottom panels). For both comparisons, we identified a robust
pattern of anti-correlation, demonstrating that the H3.3Q5A mutant induced reversed gene expression patterns compared to heroin empty vector and heroin H3.3 WT groups. To profile specific
heroin-induced gene expression changes, we employed pairwise comparisons to identify differentially expressed (DEx) genes between heroin groups (empty vector and H3.3 WT) vs. saline groups
(empty vector and H3.3 WT), defined at a false discovery rate (FDR) of <0.05, log2FC ± 0.2. We identified 736 upregulated and 1005 downregulated DEx genes between the heroin empty vs.
saline empty conditions, and 303 upregulated and 823 downregulated DEx genes between heroin H3.3 WT and saline H3.3 WT comparisons, respectively (SI Appendix, Table S1). There were only 29
DEx genes between empty vs. H3.3 WT, confirming that the majority of gene expression changes induced by the H3.3Q5A mutant are not due to exogenous expression of the histone H3.3 protein.
Importantly, reducing levels of H3Q5dop with the mutant H3.3Q5A virus reversed over 50% of gene expression changes induced by extended heroin SA abstinence, compared to both heroin empty and
heroin H3.3 WT groups (SI Appendix, Fig. S1_A-B_). In order to determine which pathways were affected by blocking H3Q5dop, we next performed a Gene Ontology Enrichment Analysis (GO) on the
set of overlapping DEx genes (_n_ = 321) that were reversed between heroin H3.3Q5A vs. heroin empty and heroin H3.3 WT conditions (SI Appendix, Fig. S1C). We found significant associations
with genes involved in opioid neuropeptide pathways, dopamine receptor signaling, cytokine responses, synaptic transmission, and regulation of neuronal action potentials (Fig. 2C),
indicating that H3Q5dop accumulation during heroin abstinence may drive specific neuroadaptations related to altered plasticity in the VTA. Interestingly, while levels of the H3Q5dop PTM
itself appear to renormalize by AD30 (SI Appendix, Fig. S2), our RNA-seq data reveals broad transcriptional changes (~800 DEx genes) at this time point as a result of blocking dopaminylation
with the AAV H3.3Q5A virus throughout heroin abstinence, with expression starting at approximately AD7 and peaking at AD28 [28]. This indicates that H3Q5dop accumulation during the course
of abstinence drives substantial and persistent molecular changes in this region that precipitate long-lasting alterations in VTA function. Although the VTA is a critical source of
dopaminergic neurotransmission to the ventral striatum, this region had surprisingly not yet been profiled at the transcriptomic level in a volitional animal model of heroin dependence.
Therefore, we also performed GO analyses of DEx genes between heroin vs. saline groups, which revealed that extended abstinence from heroin induced long-term changes in VTA interneurons, as
well as in the generation of neuronal action potentials, myelination machinery, oligodendrocyte maturation, and ECM-based remodeling and plasticity (SI Appendix, Fig. S3). To determine if
extended heroin vs. cocaine abstinence induced similar gene expression changes in the VTA, we performed odds ratio analyses to measure the extent of overlap of DEx genes in both conditions,
using our previously published cocaine dataset [11]. Interestingly, we identified a significant pattern of opposing gene expression, with robust overlaps identified between genes that were
upregulated in heroin and downregulated in cocaine (and vice versa) (SI Appendix, Fig. S4A), suggesting that these two substances likely act on similar pathways but in opposite directions
within the VTA. Furthermore, we also found that gene expression reversals induced by H3.3Q5A also displayed opposite directionality between heroin vs. cocaine (SI Appendix, Fig. S4B),
indicating that H3Q5dop likely functions as a mediator of general neural plasticity within this brain region, rather than as a downstream effector of a specific drug-induced pathway.
ACCUMULATION OF H3Q5DOP IN VTA CONTRIBUTES TO HEROIN-SEEKING AFTER EXTENDED ABSTINENCE Finally, to investigate the consequences of reducing H3Q5dop levels on heroin relapse-relevant
behaviors, we put an independent cohort of animals through chronic heroin self-administration, followed by intra-VTA viral infusions on AD1, using the same three viral vectors discussed
above (Fig. 3A, B). On AD30, animals were tested for heroin-seeking behavior in a cue-induced reinstatement paradigm. Preventing H3Q5dop accumulation in heroin SA animals significantly
reduced motivated heroin-seeking behaviors in heroin animals (Fig. 3C) via Two-way ANOVA, with significant effects of heroin (F [1,40] = 86.82, _p_ = <0.0001), virus (F [2,40] = 4.456,
_p_ = 0.017), and interaction (F [2,40] = 4.01, _p_ = 0.025) observed. Tukey’s MC tests revealed significant differences between heroin H3.3 Q5A vs. heroin empty (_p_ = 0.0007) and heroin
H3.3Q5A vs. heroin H3.3 WT (_p_ = 0.0288), and no significant effects between saline viral groups (_p_ > 0.05). Importantly, we have previously shown that this H3.3Q5A mutant histone does
not affect operant behavior for natural rewards [11]. Together, these data indicate that aberrant accumulation of H3 dopaminylation in VTA promotes a discrete transcriptional program
capable of mediating relapse associated vulnerability across pharmacologically distinct addictive substances. DISCUSSION As part of its mechanism of action, heroin exposures increase
neurotransmission from dopaminergic neurons in VTA to reward-processing projection regions in striatum, among other neuronal regions. These fluctuations in dopamine signaling are thought to
contribute to maladaptive plasticity and impaired decision-making observed during substance dependence. However, the mechanisms that mediate these processes at the epigenetic level are not
fully characterized. Here, we showed that H3Q5dop rapidly accumulates in rodent VTA at 24 h post-heroin SA, and that this increase persisted during protracted periods of heroin abstinence
(e.g., AD14). We next profiled gene expression changes that occurred after attenuating levels of H3Q5dop during heroin abstinence and identified highly significant overlap with regulation of
opioid and dopaminergic signaling, cytokine pathways, and synaptic transmission/regulation of neuronal action potential related processes. Finally, these transcriptomic changes coincided
with a significant decrease in drug-seeking behavior after 30 d of heroin abstinence. Together, these data indicate that H3Q5dop plays a critical role in promoting persistent functional
alterations that drive maladaptive behaviors related to heroin relapse vulnerability. Our findings also highlight the critical role of H3Q5dop in regulating epigenetic changes after chronic
exposures to, and abstinence from, different drugs of abuse (e.g., an opioid vs. a psychostimulant). However, we also observed key differences between the substances with respect to both the
dynamics and gene targets of H3Q5dop. First, we found that H3Q5dop is significantly increased in rodent VTA as soon as AD1 post-heroin SA, an increase that persists throughout more extended
periods of abstinence. In contrast, levels of H3Q5dop during cocaine abstinence remained unchanged 24 h after the last day of cocaine SA, and then slowly accumulated during long-term
withdrawal (e.g., AD30; a time point in which H3Q5dop is no longer globally elevated following heroin exposures). This temporal difference in H3Q5dop dynamics may reflect differences in
dopaminergic activity in response to either substance, particularly during more acute periods of abstinence—for example, electrophysiology and fiber photometry studies have demonstrated that
while cocaine exposures lead to immediate inhibition of dopaminergic VTA neurons, heroin exposures strongly activate these projections [29]. Furthermore, neurons in striatum activate
distinct pathways in downstream projection regions in response to heroin vs. cocaine, suggesting that the reward system may display circuit-specific responses to different classes of drugs
of abuse [30, 31]. Another major finding revealed in our current study relates to VTA gene expression changes mediated by H3Q5dop in response to heroin vs. cocaine SA. Our RNA-seq analyses
found that heroin abstinence induced robust changes in genes involved with interneuron transmission activity, oligodendrocyte myelination, and ECM remodeling. These transcriptomic changes
correlate to current hypotheses regarding opiate disinhibition of GABAergic regulation of dopaminergic activity through local interneuron networks in the VTA, thereby leading to increased DA
output from the VTA [32, 33]. These findings also parallel our previous work demonstrating that exposures to heroin increase expression of oligodendrocyte genes and myelination pathways in
the PFC, a phenomena that has been confirmed in recent single-cell RNA-seq studies [34, 35]. Interestingly, we also found that heroin and cocaine appear to elicit significantly opposite
patterns of gene expression in the VTA, which has also been observed in other brain regions within the mesolimbic reward pathway in human tissues, and in preclinical models [36, 37]. Our
findings suggest that while both cocaine and heroin dependence converge on similar critical molecular pathways in the VTA, these substances alter reward circuitry function by driving gene
expression in opposing directions. Importantly, reducing accumulation of H3Q5dop with a dominant negative H3.3 mutant virus led to specific gene expression changes in molecular processes
mediating action potential generation, membrane conductance, and synaptic regulation, as well as signaling cascades directly related to opioid and dopaminergic pathways. The renormalization
of these gene expression alterations led to significantly reduced drug-seeking behavior following extended abstinence from heroin SA, suggesting that these pathways contribute to relapse
vulnerability in drug dependence. Together, our data show that H3Qdop accumulation may regulate a state of increased neuronal plasticity, derived from aberrant signals that disrupt the
balance of excitatory/inhibitory modulation of VTA activity. Given that chronic heroin use followed by withdrawal induces adaptations in dopaminergic neurotransmission and changes in
membrane ion conductance and ion channels [38], it is notable that reducing H3Q5dop acts to specifically alter these pathways, indicating that this modification may actively participate in
the molecular translation of heroin withdrawal to altered neural activity. Finally, our previous work established that the H3Q5dop modification may be an upstream epigenetic regulator of
dopaminergic neurotransmission in drug dependence. Our current findings suggest that manipulation of H3Q5dop during different stages of drug dependence may represent a useful tool to reveal
important gene expression profiles that are unique to specific classes of substances. Moving forward, delineating specific transcriptional signatures that effectively promote reward-circuit
remodeling for different drugs of abuse may provide insights to guide clinicians in tailoring therapeutic strategies towards the unique mechanisms of action for a given drug class. For
example, substances like nicotine and alcohol, which also converge on promoting dopamine outflow, have distinct molecular interactions with cellular substrates in the VTA. Further study of
H3Q5dop dynamics in the context of these different substances may reveal distinct epigenetic mechanisms that translate their respective receptor-binding signals into increased plasticity.
Importantly, recent reports of sex-specific dopamine activity after heroin exposure and increased heroin-seeking behaviors in females highlight the need to expand investigations of H3Q5dop
mechanisms in female subjects [39]. Overall, the data presented here demonstrate that heroin acts through H3Q5dop deposition as an epigenetic mechanism in the VTA to recruit specific
transcriptional programs that alter dopaminergic neurotransmission and drive drug-seeking behaviors during prolonged abstinence. DATA AVAILABILITY Data from RNA-seq experiments have been
deposited in the National Center for Biotechnology Information Gene Expression Omnibus (GEO) database under accession number GSE169628. We declare that the data supporting findings for this
study are available within the article. No restrictions on data availability apply. REFERENCES * Raghavendra V, Tanga F, DeLeo JA. Inhibition of microglial activation attenuates the
development but not existing hypersensitivity in a rat model of neuropathy. J Pharmacol Exp Therapeutics. 2003;306:624–30. Article CAS Google Scholar * Kuehn BM. Samhsa: pain medication
abuse a common path to heroin: experts say this pattern likely driving heroin resurgence. JAMA. 2013;310:1433–4. Article CAS Google Scholar * Dart RC, Surratt HL, Cicero TJ, Parrino MW,
Severtson SG, Bucher-Bartelson B, et al. Trends in Opioid Analgesic Abuse and Mortality in the United States. N Eng J Med. 2015;372:241–8. Article Google Scholar * Palombi LC, St Hill CA,
Lipsky MS, Swanoski MT, Lutfiyya MN. A scoping review of opioid misuse in the rural United States. Annals of epidemiology. 2018;28:641–52. Article Google Scholar * Madras BK. The surge of
opioid use, addiction, and overdoses: responsibility and response of the US health care system. JAMA Psychiatry. 2017;74:441–2. Article Google Scholar * Hyman SE, Malenka RC. Addiction and
the brain: the neurobiology of compulsion and its persistence. Nat Rev Neurosci. 2001;2:695–703. Article CAS Google Scholar * Nestler EJ. Psychogenomics: opportunities for understanding
addiction. J Neurosci. 2001;21:8324–7. Article CAS Google Scholar * Kalivas PW. The glutamate homeostasis hypothesis of addiction. Nat Rev Neurosci. 2009;10:561–72. Article CAS Google
Scholar * Wolf ME. The Bermuda triangle of cocaine-induced neuroadaptations. Trends Neurosci. 2010;33:391–8. Article CAS Google Scholar * Werner CT, Altshuler RD, Shaham Y, Li X.
Epigenetic Mechanisms in Drug Relapse. Biol Psychiatry. 2021;89:331–8. Article CAS Google Scholar * Lepack AE, Werner CT, Stewart AF, Fulton SL, Zhong P, Farrelly LA, et al.
Dopaminylation of histone H3 in ventral tegmental area regulates cocaine seeking. Science. 2020;368:197–201. Article CAS Google Scholar * Farrelly LA, Thompson RE, Zhao S, Lepack AE, Lyu
Y, Bhanu NV, et al. Histone serotonylation is a permissive modification that enhances TFIID binding to H3K4me3. Nature. 2019;567:535–9. Article CAS Google Scholar * Zhao S, Chuh KN, Zhang
B, Dul BE, Thompson RE, Farrelly LA, et al. Histone H3Q5 serotonylation stabilizes H3K4 methylation and potentiates its readout. Proceedings of the National Academy of Sciences. 2021;118. *
Gerasimov MR, Ashby CR Jr, Gardner EL, Mills MJ, Brodie JD, Dewey SL. Gamma–vinyl GABA inhibits methamphetamine, heroin, or ethanol–induced increases in nucleus accumbens dopamine. Synapse.
1999;34:11–9. Article CAS Google Scholar * Martin JA, Werner CT, Mitra S, Zhong P, Wang Z-J, Gobira PH, et al. A novel role for the actin-binding protein drebrin in regulating opiate
addiction. Nat Commun. 2019;10:1–11. Article Google Scholar * Mitra S, Gobira PH, Werner CT, Martin JA, Iida M, Thomas SA, et al. A role for the endocannabinoid enzymes monoacylglycerol
and diacylglycerol lipases in cue–induced cocaine craving following prolonged abstinence. Addict Biol. 2021:e13007. * Shaham Y, Shalev U, Lu L, De Wit H, Stewart J. The reinstatement model
of drug relapse: history, methodology and major findings. Psychopharmacol. 2003;168:3–20. Article CAS Google Scholar * Shalev U, Grimm JW, Shaham Y. Neurobiology of relapse to heroin and
cocaine seeking: a review. Pharmacol Rev. 2002;54:1–42. Article CAS Google Scholar * Lepack AE, Bagot RC, Peña CJ, Loh Y-HE, Farrelly LA, Lu Y, et al. Aberrant H3. 3 dynamics in NAc
promote vulnerability to depressive-like behavior. Proc Natl Acad Sci. 2016;113:12562-7. * Okbay A, Baselmans BM, De Neve J-E, Turley P, Nivard MG, Fontana MA, et al. Genetic variants
associated with subjective well-being, depressive symptoms, and neuroticism identified through genome-wide analyses. Nat Genet. 2016;48:624–33. Article CAS Google Scholar * Habib N,
McCabe C, Medina S, Varshavsky M, Kitsberg D, Dvir-Szternfeld R, et al. Disease-associated astrocytes in Alzheimer’s disease and aging. Nat Neurosci. 2020;23:701–6. Article CAS Google
Scholar * Fanous S, Guez–Barber DH, Goldart EM, Schrama R, Theberge FR, Shaham Y, et al. Unique gene alterations are induced in FACS–purified Fos–positive neurons activated during
cue–induced relapse to heroin seeking. J Neurochem. 2013;124:100–8. Article CAS Google Scholar * Love MI, Huber W, Anders S. Moderated estimation of fold change and dispersion for RNA-seq
data with DESeq2. Genome Biol. 2014;15:1–21. Article Google Scholar * Cahill KM, Huo Z, Tseng GC, Logan RW, Seney ML. Improved identification of concordant and discordant gene expression
signatures using an updated rank-rank hypergeometric overlap approach. Sci Rep. 2018;8:1–11. Article CAS Google Scholar * Shen L, Sinai M GeneOverlap: Test and visualize gene overlaps. R
package version 1.20. 0. 2019. * Alhamdoosh M, Ng M, Wilson NJ, Sheridan JM, Huynh H, Wilson MJ, et al. Combining multiple tools outperforms individual methods in gene set enrichment
analyses. Bioinformatics. 2017;33:414–24. CAS PubMed Google Scholar * Pickens CL, Airavaara M, Theberge F, Fanous S, Hope BT, Shaham Y. Neurobiology of the incubation of drug craving.
Trends Neurosci. 2011;34:411–20. Article CAS Google Scholar * Reimsnider S, Manfredsson FP, Muzyczka N, Mandel RJ. Time course of transgene expression after intrastriatal pseudotyped
rAAV2/1, rAAV2/2, rAAV2/5, and rAAV2/8 transduction in the rat. Mol Ther. 2007;15:1504–11. Article CAS Google Scholar * Wei C, Han X, Weng D, Feng Q, Qi X, Li J, et al. Response dynamics
of midbrain dopamine neurons and serotonin neurons to heroin, nicotine, cocaine, and MDMA. Cell Discov. 2018;4:1–16. Article CAS Google Scholar * Yang H, de Jong JW, Tak Y, Peck J, Bateup
HS, Lammel S. Nucleus accumbens subnuclei regulate motivated behavior via direct inhibition and disinhibition of VTA dopamine subpopulations. Neuron. 2018;97:434–49. e4 Article CAS Google
Scholar * Al-Hasani R, McCall JG, Shin G, Gomez AM, Schmitz GP, Bernardi JM, et al. Distinct subpopulations of nucleus accumbens dynorphin neurons drive aversion and reward. Neuron.
2015;87:1063–77. Article CAS Google Scholar * Rogers J, Ghee S, See R. The neural circuitry underlying reinstatement of heroin-seeking behavior in an animal model of relapse.
Neuroscience. 2008;151:579–88. Article CAS Google Scholar * De Vries TJ, Shippenberg TS. Neural systems underlying opiate addiction. J Neurosci. 2002;22:3321–5. Article Google Scholar *
Martin JA, Caccamise A, Werner CT, Viswanathan R, Polanco JJ, Stewart AF, et al. A novel role for oligodendrocyte precursor cells (OPCs) and Sox10 in mediating cellular and behavioral
responses to heroin. Neuropsychopharmacology. 2018;43:1385–94. Article CAS Google Scholar * Avey D, Sankararaman S, Yim AK, Barve R, Milbrandt J, Mitra RD. Single-cell RNA-seq uncovers a
robust transcriptional response to morphine by glia. Cell reports. 2018;24:3619–29. e4. Article CAS Google Scholar * Albertson DN, Schmidt CJ, Kapatos G, Bannon MJ. Distinctive profiles
of gene expression in the human nucleus accumbens associated with cocaine and heroin abuse. Neuropsychopharmacology. 2006;31:2304–12. Article CAS Google Scholar * Badiani A, Belin D,
Epstein D, Calu D, Shaham Y. Opiate versus psychostimulant addiction: the differences do matter. Nat Rev Neurosci. 2011;12:685–700. Article CAS Google Scholar * Mackler SA, Eberwine JH.
Cellular adaptation to opiates alters ion-channel mRNA levels. Proc Natl Acad Sci. 1994;91:385–9. Article CAS Google Scholar * George BE, Barth SH, Kuiper LB, Holleran KM, Lacy RT,
Raab-Graham KF, et al. Enhanced heroin self-administration and distinct dopamine adaptations in female rats. Neuropsychopharmacology. 2021:1-10. Download references ACKNOWLEDGEMENTS The NIDA
Drug Supply Program generously gifted the heroin used in these studies. FUNDING This work was supported by grants from the National Institutes of Health: DP1 DA042078 (IM), R01 DA015521
(DMD), R21 DA0488554 (DMD), U01 DA051947 (DMD), F31 MH124425 (SLF), F31 DA045428 (AEL) and F99 NS108543 (JAM), as well as awards from: Brain Research Foundation’s Fay/Frank Seed Grant Award
(IM). The authors have nothing to disclose AUTHOR INFORMATION Author notes * These authors contributed equally: Sasha L. Fulton, Swarup Mitra. AUTHORS AND AFFILIATIONS * Nash Family
Department of Neuroscience, Friedman Brain Institute, Icahn School of Medicine at Mount Sinai, New York, NY, 10029, USA Sasha L. Fulton, Ashley E. Lepack, Andrew F. Stewart & Ian Maze *
Department of Pharmacology and Toxicology, Program in Neuroscience, University at Buffalo, Buffalo, NY, 14214, USA Swarup Mitra, Jennifer A. Martin, Jacob Converse, Mason Hochstetler &
David M. Dietz * Department of Pharmacological Sciences, Icahn School of Medicine at Mount Sinai, New York, NY, 10029, USA Ian Maze * Howard Hughes Medical Institute, Icahn School of
Medicine at Mount Sinai, New York, NY, 10029, USA Ian Maze Authors * Sasha L. Fulton View author publications You can also search for this author inPubMed Google Scholar * Swarup Mitra View
author publications You can also search for this author inPubMed Google Scholar * Ashley E. Lepack View author publications You can also search for this author inPubMed Google Scholar *
Jennifer A. Martin View author publications You can also search for this author inPubMed Google Scholar * Andrew F. Stewart View author publications You can also search for this author
inPubMed Google Scholar * Jacob Converse View author publications You can also search for this author inPubMed Google Scholar * Mason Hochstetler View author publications You can also search
for this author inPubMed Google Scholar * David M. Dietz View author publications You can also search for this author inPubMed Google Scholar * Ian Maze View author publications You can
also search for this author inPubMed Google Scholar CONTRIBUTIONS SLF, SM, AEL, DMD, and IM designed research; SLF, SM, AEL, JAM, JC, MH, and AFS performed research; SLF, SM, AEL, DMD, and
IM analyzed data; SLF, SM, DMD, and IM wrote the paper. CORRESPONDING AUTHORS Correspondence to David M. Dietz or Ian Maze. ETHICS DECLARATIONS COMPETING INTERESTS The authors declare no
competing interests. ADDITIONAL INFORMATION PUBLISHER’S NOTE Springer Nature remains neutral with regard to jurisdictional claims in published maps and institutional affiliations.
SUPPLEMENTARY INFORMATION SUPPLEMENTAL MATERIAL EXTENDED DATA TABLE 1 RIGHTS AND PERMISSIONS OPEN ACCESS This article is licensed under a Creative Commons Attribution 4.0 International
License, which permits use, sharing, adaptation, distribution and reproduction in any medium or format, as long as you give appropriate credit to the original author(s) and the source,
provide a link to the Creative Commons license, and indicate if changes were made. The images or other third party material in this article are included in the article’s Creative Commons
license, unless indicated otherwise in a credit line to the material. If material is not included in the article’s Creative Commons license and your intended use is not permitted by
statutory regulation or exceeds the permitted use, you will need to obtain permission directly from the copyright holder. To view a copy of this license, visit
http://creativecommons.org/licenses/by/4.0/. Reprints and permissions ABOUT THIS ARTICLE CITE THIS ARTICLE Fulton, S.L., Mitra, S., Lepack, A.E. _et al._ Histone H3 dopaminylation in ventral
tegmental area underlies heroin-induced transcriptional and behavioral plasticity in male rats. _Neuropsychopharmacol._ 47, 1776–1783 (2022). https://doi.org/10.1038/s41386-022-01279-4
Download citation * Received: 10 May 2021 * Revised: 10 January 2022 * Accepted: 14 January 2022 * Published: 29 January 2022 * Issue Date: September 2022 * DOI:
https://doi.org/10.1038/s41386-022-01279-4 SHARE THIS ARTICLE Anyone you share the following link with will be able to read this content: Get shareable link Sorry, a shareable link is not
currently available for this article. Copy to clipboard Provided by the Springer Nature SharedIt content-sharing initiative