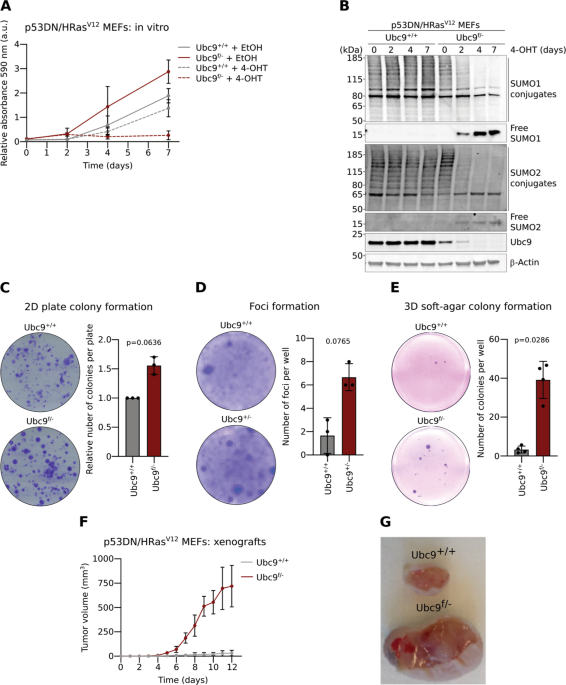
An unanticipated tumor-suppressive role of the sumo pathway in the intestine unveiled by ubc9 haploinsufficiency
- Select a language for the TTS:
- UK English Female
- UK English Male
- US English Female
- US English Male
- Australian Female
- Australian Male
- Language selected: (auto detect) - EN
Play all audios:

ABSTRACT Sumoylation is an essential posttranslational modification in eukaryotes that has emerged as an important pathway in oncogenic processes. Most human cancers display hyperactivated
sumoylation and many cancer cells are remarkably sensitive to its inhibition, thus supporting application of chemical sumoylation inhibitors in cancer treatment. Here we show, first, that
transformed embryonic fibroblasts derived from mice haploinsufficient for Ubc9, the essential and unique gene encoding the SUMO E2 conjugating enzyme, exhibit enhanced proliferation and
transformed phenotypes in vitro and as xenografts ex vivo. To then evaluate the possible impact of loss of one _Ubc9_ allele in vivo, we used a mouse model of intestinal tumorigenesis. We
crossed _Ubc9__+/−_ mice with mice harboring a conditional ablation of Apc either all along the crypt–villus axis or only in Lgr5+ crypt-based columnar (CBC) cells, the cell compartment that
includes the intestinal stem cells proposed as cells-of-origin of intestinal cancer. While _Ubc9__+/−_ mice display no overt phenotypes and no globally visible hyposumoylation in cells of
the small intestine, we found, strikingly, that, upon loss of Apc in both models, _Ubc9__+/−_ mice develop more (>2-fold) intestinal adenomas and show significantly shortened survival.
This is accompanied by reduced global sumoylation levels in the polyps, indicating that Ubc9 levels become critical upon oncogenic stress. Moreover, we found that, in normal conditions,
_Ubc9__+/−_ mice show a moderate but robust (15%) increase in the number of Lgr5+ CBC cells when compared to their wild-type littermates, and further, that these cells display higher degree
of stemness and cancer-related and inflammatory gene expression signatures that, altogether, may contribute to enhanced intestinal tumorigenesis. The phenotypes of Ubc9 haploinsufficiency
discovered here indicate an unanticipated tumor-suppressive role of sumoylation, one that may have important implications for optimal use of sumoylation inhibitors in the clinic. SIMILAR
CONTENT BEING VIEWED BY OTHERS UBXN2A SUPPRESSES THE RICTOR-MTORC2 SIGNALING PATHWAY, AN ESTABLISHED TUMORIGENIC PATHWAY IN HUMAN COLORECTAL CANCER Article 10 April 2023 THE AMINO ACID
TRANSPORTER SLC7A5 IS REQUIRED FOR EFFICIENT GROWTH OF KRAS-MUTANT COLORECTAL CANCER Article 07 January 2021 ENHANCED SREBP2-DRIVEN CHOLESTEROL BIOSYNTHESIS BY PKCΛ/Ι DEFICIENCY IN
INTESTINAL EPITHELIAL CELLS PROMOTES AGGRESSIVE SERRATED TUMORIGENESIS Article Open access 13 December 2023 INTRODUCTION SUMO modification has emerged as an essential and highly dynamic
posttranslational modification (PTM) that targets hundreds of cellular proteins (the “sumoylome”), thus affecting most, if not all, fundamental processes carried out by eukaryotic cells [1,
2]. As with other, “small-molecule” PTMs (phosphorylation, acetylation, methylation, etc.), the covalent attachment of the ~11 KDa SUMO peptide changes the interacting properties of its
target proteins and hence their functions as parts of larger molecular complexes or pathways. Numerous stresses and pathological conditions, notably infection and cancer, result in the rapid
modification, but also de-modification, of numerous substrates with, at times, profound effects on the composition of the cellular sumoylome [2,3,4,5,6,7]. Conversely, strong perturbation
of the SUMO system by inhibiting the activity of the unique E1 (SAE1/SAE2) or E2 (Ubc9) enzymes, for example, provokes significant cell stress that leads to growth arrest, senescence or
apoptosis [8,9,10,11]. As with stress, the cancerous state is associated with dysregulated cellular sumoylation dynamics stemming from the altered, mostly upregulated expression of SUMO
pathway components (i.e., SUMO, E1, E2, and SENPs) that affects numerous SUMO-targeted cellular factors, including key tumor suppressors and oncoproteins [6, 7]. The concept of “non-oncogene
addiction” [12, 13] has been suggested as a possible view of SUMO’s roles in cancer: the idea that with strong oncogenic signaling, for example by elevated Myc or Ras activity, the
stress-mitigating functions of sumoylation become indispensable for cancer cell survival [14,15,16]. Clearly, this makes SUMO modification an attractive target for cancer therapy,
particularly in cases such as Myc-driven cancers in which the oncoprotein itself remains out of range. Our understanding of the origins of colorectal cancer (CRC) has greatly benefited from
a large body of work, including elegant lineage tracing studies in mice [17]. These studies provided a detailed spatial and temporal description of the rapid, continuous and orderly
developmental progression that links the Lgr5+ intestinal stem cell, Paneth and transit-amplifying cell compartment with the differentiated epithelial cells to constitute the functional
crypt–villus anatomy of the small intestinal epithelium. Ninety percent of CRC cases follow a well-defined and ordered chain of genetic events starting with perturbation of APC/β-catenin
signaling followed by dysregulation of the KRAS, TP53, and/or PIK3CA pathways [18,19,20]. Significantly, the progression from normal intestinal mucosa, through aberrant crypt foci, small
intestinal adenomas or polyps to malignant tumors (but rarely to colonic tumors) is recapitulated in mouse models for the specific and inducible ablation of the Apc tumor suppressor [20].
The characterization of Lgr5+ crypt-based columnar (CBC) cells, that include the intestinal stem cells (ISCs) shown to act as cells-of-origin of intestinal cancer [21, 22], attests to the
value of these models. We have shown previously that complete loss of Ubc9, and hence of sumoylation, is early embryonic lethal in mice [10]. Similarly, acute loss of Ubc9 in adult mice
leads to rapid death caused by degeneration of the intestinal epithelium [23]. In this setting, lack of sumoylation primarily affects the crypt compartment by causing the disappearance of
the Lgr5+ CBC cells that leads to rapid disorganization of the crypt–villus axis, thereby severely compromising the genesis, function, positioning and survival of all differentiated
epithelial cells. Since sumoylation is essential for cell and animal viability, we analyzed here the role of the SUMO pathway in cancer-related processes using in vitro and in vivo Ubc9
haploinsufficient models. Loss of a single _Ubc9_ allele in transformed fibroblasts conferred enhanced proliferative and transformed phenotypes. In addition, while without any major
phenotypic effects in mice in normal conditions, Ubc9 haploinsufficiency was found to enhance intestinal tumorigenesis and to provoke earlier death in Apc-deficient backgrounds. Furthermore,
we found this phenotype to be associated with both increased numbers of crypt-based Lgr5+ CBC cells and exacerbated stemness, inflammatory and cancer-related gene expression programs in
this cellular context. These results uncover an unexpected oncosuppressive role of sumoylation with potential impact for cancer treatment. RESULTS UBC9 HAPLOINSUFFICIENCY PROMOTES CELL
GROWTH AND TRANSFORMED PHENOTYPES IN VITRO AND EX VIVO To address the role of Ubc9 in controlling cell proliferation and tumorigenesis, we used mice haploinsufficient for Ubc9 which harbour
a _floxed_ and a _null Ubc9_ allele (_Ubc9__f/_−) [23]. While _Ubc9_−_/−_ animals are embryonic lethal, _Ubc9__f/−_, like _Ubc9__+/−_ mice display no overt phenotype in normal conditions,
apart from a slight (10%) decrease in body size and body weight, when compared to wild-type (WT) littermates [10]. Murine embryo fibroblasts (MEFs) derived from a pool of three E12.5 WT or
_Ubc9__f/−_ embryos were transformed by retroviral transduction of a dominant-negative (DN) p53 mutant together with the HRASV12 oncogene. _Ubc9__f/−_ MEFs were found to grow significantly
faster than _Ubc9_+/+ MEFs and inducible deletion of the remaining _floxed Ubc9_ allele by 4-hydroxytamoxifen (4-OHT) [23] expectedly abrogated their proliferation capacity (Fig. 1a). This
pattern correlated with suppression of Ubc9 protein expression, reduction in global sumoylation and appearance of free SUMO1 and SUMO2/3 (hereafter SUMO2−) (Fig. 1b). To further characterize
the proliferative advantage of non-treated _Ubc9__f/−_ over _Ubc9_+/+ transformed MEFs, we analyzed cell cycle distribution and apoptosis rates at 4 days post-passage, when cells are still
non-quiescent but the difference in cell numbers is clearly evident (Fig. S1a). While the cell cycle distribution was very similar for cells of both genotypes (Fig. S1b), we found a slightly
larger fraction of apoptotic cells in _Ubc9__+/+_ (mean 9.8%) compared to _Ubc9__f/−_ (6.7%) cells (Fig. S1c), a difference, however, likely insufficient to fully explain the observed,
significant 1.7-fold difference in cell numbers after 4 days’ growth. To next see whether the growth difference could be related to the levels of the exogenous oncogenes, we compared the
expression of p53 and HRAS between both genotypes. RT-qPCR analysis showed that levels of endogenous _p53_ and _HRas_ are similar in both contexts (Fig. S1d). Interestingly, however,
expression of exogenous murine _p53DN_ and human _HRAS__V12_ mRNA was higher in _Ubc9__+/+_ cells (Fig. S1e), a finding also confirmed for HRAS proteins by Western blot (Fig. S1f). Together,
this suggests that the increased proliferation capacity displayed by mutant MEFs is not driven by higher oncogene activity but might rather be a consequence of a more transformation-prone
basal state in Ubc9 haploinsufficient MEFs. The ability of transformed cells to form colonies of clonal origin on plates and in agar, or to form foci when seeded at high densities, is
related to their capacity to grow and divide in an anchorage-dependent and anchorage-independent manner, or to lose contact inhibition, respectively [24,25,26]. Transformed _Ubc9__f/−_ MEFs
formed colonies and foci more efficiently than their WT counterparts, as seen by the 1.6-, 4- and 12-fold growth increase in 2D plate colony-, foci formation and 3D soft-agar colony assays,
respectively (Fig. 1c–e). To transpose these findings in vivo, we xenografted nude mice with transformed _Ubc9_+/+ and _Ubc9__f/−_ MEFs and found that Ubc9 haploinsufficiency greatly
enhanced tumor growth, consistent with the findings obtained in vitro (Fig. 1f–g). Altogether, these data suggest that mild Ubc9 downregulation confers a growth advantage consistent with the
establishment of an exacerbated transformed state. UBC9 HAPLOINSUFFICIENCY FAVORS POLYP FORMATION IN AN _APC__F/+_ INTESTINAL CANCER MOUSE MODEL We next investigated the effect of Ubc9
haploinsufficiency on tumor initiation and development in vivo, using the _Apc__f/+_ intestinal cancer mouse model. First, and in agreement with the situation in WT and _Ubc9__f/−_ MEFs
(compare “0” lanes in Fig. 1b), we found that global profiles of SUMO-conjugated proteins in whole intestinal extracts from WT and _Ubc9__+/−_ mice are indistinguishable (Fig. 2a), although
this does not exclude that effects on specific substrates go undetected by this global analysis. Of note, the Ubc9 protein level was reduced to about a half of normal levels in _Ubc9__f/−_
intestines, indicating the absence of compensatory mechanisms for Ubc9 expression in these animals (Fig. 2a). Furthermore, anatomopathological analysis of intestines from mice of both
genotypes did not reveal significant differences in the general architecture of crypts (Fig. S2a). Next, we generated _Ubc9;Villin-Cre__ERT2__;Apc__f/+_ mouse strains in which CreERT2
recombinase expression is driven by the intestine-specific _Villin_ promoter [27, 28]. After CreERT2 activation by intraperitoneal injections of 4-OHT, the _lox_P-flanked exon 14 in one
allele of the _Apc_ tumor suppressor is deleted all along the crypt–villus axis leading to the initial stage of the multi-step transformation process [19, 20] in either the WT or _Ubc9__+/−_
genetic background. Surprisingly, we found that 4-OHT treatment resulted in shortened survival of _Ubc9__+/−_ mice compared to their _Ubc9_+/+ counterparts (Fig. 2b). To determine whether
this effect was related to tumor incidence and/or load, we assessed the number of adenomatous polyps (≥0.5 mm in diameter) generated 12 weeks after 4-OHT treatment. We found that _Ubc9__+/−_
mice developed twofold more adenomas, with medians of 10 and 22 polyps per animal in small intestines of _Ubc9__+/+_ and _Ubc9__+/−_ mice, respectively (Figs. 2c, S2b). Hematoxylin and
eosin (H&E) staining of these samples further confirmed the increased frequency of both dysplasia and tumors in _Ubc9__+/−_ mice (Figs. 2d, S2c). No differences in the morphology and
general organization of tumors and dysplasia foci were found (Figs. 2d, S2b–d). Finally, the median size of _Ubc9__+/−_ polyps appears to be half that of WT (Fig. S2e), although, due to
rounding off the measurements to the nearest 1 mm increment, the real median size difference may likely be less pronounced. A control experiment to address the possibility that Ubc9
haploinsufficiency merely accelerates Apc loss, and hence tumorigenesis, revealed that reduction of Ubc9 levels, here carried out in human U2OS cells, likely does not affect homologous
recombination (HR), a process strictly required for tumorigenesis by loss of the second, WT Apc allele in the intestines of _Ubc9;Villin-CreERT2;Apc__f/+_ mice (Fig. S2h–j). This suggests
that the higher adenoma frequency seen in _Ubc9__+/−_ mice is unlikely due to enhanced HR-dependent inactivation of the native _Apc_ allele. Together, these data indicate that decreasing
Ubc9 levels to a half-dose promotes intestinal tumorigenesis. Moreover, they suggest that tumor initiation, rather than development, is enhanced in this process. _UBC9__+/+_ AND _UBC9__+/−_
MICE DISPLAY SIMILAR TRANSCRIPTOMIC PROFILES IN ADENOMATOUS POLYPS OR IN NORMAL INTESTINAL TISSUE We next sought to pinpoint the molecular pathways responsible for the stimulated intestinal
tumorigenesis observed in _Ubc9__+/−_ mice. For this, we first compared Ubc9 protein levels in normal (N) and polyp (P) intestinal samples from _Villin-Cre__ERT2__;Apc__f/+_ mice 12 weeks
after 4-OHT treatment. Higher c-Myc levels confirmed the transformed nature of polyp samples from both _Ubc9__+/+_ and _Ubc9__+/−_ genotypes (Fig. 2e). Besides the expected reduction in
_Ubc9__+/−_ samples, interestingly, Ubc9 levels were nevertheless higher in polyps than in adjacent normal tissue in both the WT and the _Ubc9__+/−_ contexts (Fig. 2e). Transcriptomic
analysis of dissected polyps as well as of neighboring normal intestinal tissues from these animals revealed a total of only 11 differentially-expressed genes (DEGs, |log2FC| > log2(1.5)
and adj. _p_ value (FDR) < 0.05), with 7 and 4 up- and downregulated, respectively, in _Ubc9__+/−_ polyps when compared to WT (Fig. S2f, Table S1). Similar analysis of the neighboring
normal intestinal tissue yielded only a set of 31 DEGs, of which 8 and 23 were up- and downregulated, respectively, in the _Ubc9__+/−_ animals (Fig. S2g, Table S2). Gene Ontology (GO)
analysis applied to these small lists of genes failed to discern any specific ontology term related to cancer development, suggesting that _Ubc9_ status has little or no effect on the
specific gene expression signatures of polyps or normal intestinal tissue, or that analysis of these “bulk” tissues may have failed to unmask cell type-specific transcriptional differences.
UBC9 HAPLOINSUFFICIENCY INCREASES TUMORIGENESIS UPON APC LOSS IN LGR5+ CBC CELLS Given the enhancing effect of reduced Ubc9 levels on intestinal tumorigenesis, yet the absence of a clear
transcriptomic effect in either bulk polyps or normal intestine, we focused subsequent analyses on the more restricted set of CBC cells that includes early progenitors, and importantly, the
intestinal stem cells [22]. For this, we generated _Ubc9;Lgr5-IRES-EGFP-Cre__ERT2__;Apc__f/+_ mouse strains in which deletion of one _lox_P-flanked _Apc_ allele is restricted to CBC cells
[21]. In line with previous results (Fig. 2b), _Ubc9__+/−_ 4-OHT-treated mice displayed shorter survival than their _Ubc__+/+_ counterparts (Figs. 3a, S3a). Moreover, mutant mice developed
>2-fold more adenomas (≥0.5 mm in diameter), albeit with smaller size, in the small intestine 16 weeks after 4-OHT treatment (Figs. 3b, S3b). It is noteworthy that transformation rates in
_Lgr5-IRES-EGFP-Cre__ERT2_ mice were approximately threefold lower than previously seen in _Ubc9;Villin-Cre__ERT2__;Apc__f/+_ animals, with medians of 2.5 and 6.5 polyps per mouse counted
in _Ubc9__+/+_ and _Ubc__+/−_ mice, respectively. This is also in line with the improved overall survival of _Ubc9;Lgr5-IRES-EGFP-Cre__ERT2__;Apc__f/+_ compared to
_Ubc9;Villin-Cre__ERT2__;Apc__f/+_ mice (compare Figs. 2b, 3a). Histological analysis again revealed no differences in morphology or general organization of lesions attributable to Ubc9
status (Fig. 3c). Altogether, these data show that loss of one _Ubc9_ allele also enhances tumor formation in vivo in an _Apc_-_null_ background limited to CBC cells. TRANSCRIPTOMIC ANALYSIS
OF LGR5+ CBC CELLS REVEALS A CANCER-RELATED AND PRO-INFLAMMATORY BASAL STATE UNDER UBC9 HAPLOINSUFFICIENCY Taking advantage of _Lgr5_-driven expression of EGFP in _Lgr5-IRES-EGFP-Cre__ERT2_
mice, we obtained fluorescence-activated cell sorting (FACS)-purified Lgr5-EGFP+ cells derived from normal (_Apc__+/+_) intestines from both _Ubc9__+/+_ and _Ubc9__+/−_ mice and performed a
comparative analysis of their transcriptomic profiles (Fig. S4a). We found 205 DEGs (|log2FC| > log2(1.5) and adj. _p_ value (FDR) < 0.05) in _Ubc9__+/−_ Lgr5-EGFP+ cells, when
compared to _Ubc9__+/+_ Lgr5-EGFP+ cells, of which 92 and 113 were up- and downregulated, respectively (Fig. 4, Table S3). Interrogation of curated gene expression databases, using as
template up- and downregulated genes separately, now revealed a group of DEGs that have been associated with different types of cancers. Among the upregulated genes were genes reported to
have increased expression in HRas-transformed rat fibroblasts (Fig. 4b, Tables S3, S4). Among downregulated DEGs in _Ubc9__+/−_ Lgr5-EGFP+ cells, up to 17% were genes found to be repressed
in early gastric cancer, colorectal adenocarcinoma, E2F1-overexpressing hepatocellular carcinoma and a subtype of basal breast cancer. Significantly, some downregulated genes (_Capn9,
Pnliprp1, Pnliprp2, Cgref1, Cyp2u1, Aldh1a1_, and _Car4_) had also been found previously [29] to be upregulated in intestinal crypts upon deletion of β-catenin (_CTNNB1;_ Fig. 4b, Tables S3,
S4). A further significant feature suggested by GO analysis of genes upregulated in _Ubc9__+/−_ Lgr5-EGFP+ cells were top-hit signatures for the inflammatory response, innate immune
response, genes involved in the response mediated by the membrane-bound toll-like receptors, complement activation and response to bacteria (Fig. 4b, Table S4). Genes from the
above-mentioned signatures represent 25% of the upregulated DEGs found in _Ubc9__+/−_ Lgr5-EGFP+ cells (Table S3), indicating that loss of one Ubc9 allele triggers a pro-inflammatory state
in Lgr5+ CBC cells. _UBC9__+/−_ CRYPTS HARBOR MORE LGR5+ CBC CELLS We next assessed whether Ubc9 haploinsufficiency could be associated with changes in intestinal crypts in
_Lgr5-IRES-EGFP-Cre__ERT2_ mice. In order to evaluate the localization of both Lgr5+ and Paneth cells, intestines from _Ubc9__+/+_ and _Ubc9__+/−_ mice were prepared for confocal microscopy
and analyzed for EGFP and lysozyme, respectively. No differences were detected in the general architecture or localization of Lgr5-EGFP+ cells and Paneth cells within crypts from mice of
both genotypes (Figs. 5a, S2a). Strikingly, blinded counting, however, revealed an elevated number of small intestinal Lgr5-EGFP+ CBC cells in _Ubc9__+/−_ crypts (medians of 21 and 24 per
crypt for _Ubc9__+/+_ and _Ubc9__+/−_, respectively; Fig. 5a, b). This trend was consistent all along the small intestine but was not seen in colonic crypts (Fig. S5a, b). Thus, Ubc9
haploinsufficiency leads to a modest (15%) but significant expansion of the cell compartment containing intestinal stem cells. In addition, comparison of our transcriptomic data with a
previously identified Lgr5+ stem cell signature gene set [30] revealed a significant enrichment of the signature genes in the Ubc9_+/−_ CBC cells, suggesting a higher stemness state in
mutant cells compared to the WT (Fig. 5c, Table S5). ENHANCED SUMOYLATION IN INTESTINAL CRYPTS AND POLYPS Finally, we analyzed the sumoylation status in partially-purified villi and
crypt-based cells from _Ubc9__+/+_ and _Ubc9__+/−_ animals. For this, washed, longitudinally-opened intestines were first scraped to release villi cells and then treated with EDTA to
liberate crypt-based cells for analysis by western blotting. As expected, expression of markers for undifferentiated cells, c-Myc [31], and differentiated cells, Hoxa5 [32], was higher and
lower, respectively, in crypt-enriched compared to villi-containing samples (Fig. 5d). In line with this observation, mRNA expression of β-catenin/Wnt pathway components known to be highly
expressed in crypts, such as c-Myc, Smoc2 and Axin2 [30] was also found to be elevated (Fig. S5c, “C”), whereas expression of the differentiation marker Krt20 [33] was found to be higher in
villi-enriched samples (Fig. S5c, “V”), thus also validating the proper separation of cell populations. Significantly, total free SUMO and SUMO conjugate levels were higher in crypt-enriched
cell fraction (Fig. 5d, “C”), with, however, no clear differences between _Ubc9__+/+_ and _Ubc9__+/−_ samples, as also seen in whole intestine (Fig. 2a). Higher Ubc9 (see also Fig. 2e) and
free SUMO levels were similarly observed in adenomatous polyps (P) when compared to normal tissue (N) in both _Ubc9__+/+_ and _Ubc9__+/−_ mice in an Apc-loss background (Fig. S5d).
Remarkably, while this was accompanied by increased levels of SUMO conjugates in polyps from WT mice, we failed to detect such globally enhanced sumoylation in the tumors from _Ubc9__+/−_
mice, indicating that, in the latter, Ubc9 levels appear limiting for efficient sumoylation. Together, these results point towards a greater global SUMO pathway activity in both the
proliferative crypt compartment and in the dysplastic polyp tissue. Of note, this is consistent with findings in human colon adenocarcinomas that also exhibit elevated Ubc9 protein
expression [34] and almost twofold increases in median transcript levels in tumors (_n_ = 275) compared to non-tumor (_n_ = 349) tissues (GEPIA database,
http://gepia.cancer-pku.cn/index.html, queried for UBE2I (Ubc9)) [35]. DISCUSSION Here we have shown that Ubc9 haploinsufficiency enhances tumorigenesis and lethality in Apc loss-driven
mouse models of intestinal neoplasia. This surprising outcome is associated with a moderate but detectable (15%) increase in the number of Lgr5+ CBC cells, which, in addition, display a
higher degree of stemness and cancer-, inflammation- and innate immunity-related gene expression signatures. We also found that Ubc9 haploinsufficiency enhances the proliferative capacities
of transformed MEFs both in vitro and ex vivo. Together, these studies establish an as yet unappreciated tumor-suppressive activity for sumoylation. A basic question emerging from these
results is whether Ubc9 haploinsufficiency indeed translates into reduced cellular sumoylation, given that globally visible SUMO conjugate levels appear largely unaffected by loss of one
_Ubc9_ allele, notably in transformed MEFs and healthy intestine (Figs. 1b, 2a). It is difficult to imagine, however, that the observed phenotypes under haploinsufficiency result from
compromised sumoylation-independent Ubc9 activity. Rather, we suggest the involvement of specific “under-modified” substrate conjugates, whose detection is masked here by other,
abundantly-modified proteins in such global analysis. The identification of these specific substrates, although technically challenging, may thus shed important light on the pathways and
molecular mechanisms involved. Moreover, while Ubc9 haploinsufficiency does not always reduce sumoylation visibly, it does reduce sumoylation capacity, as seen most clearly in polyps, a
tumor state highly demanding in sumoylation (Fig. S5d). This indicates that different cell types make different demands on the SUMO system and, conversely, that they might therefore display
different sensitivities to its reduction. The smaller polyp size under Ubc9 haploinsufficiency (Figs. S2e, S3b) indeed suggests that insufficient sumoylation capacity may be a handicap for
tumor growth. Why, in contrast, Ubc9 haploinsufficiency in transformed MEFs results in enhanced proliferation (Fig. 1) could be explained by both the reduction of an as yet uncharacterized
growth-inhibiting function of sumoylation, that may also apply to Lgr5+ CBC cells (Fig. 5a, b), and to a reduced sensitivity to inhibition of these different cell types. In both cases our
results show that reduced sumoylation capacity, when mild, is not necessarily associated with reduced proliferative capacity. Our findings that _Ubc9__+/−_ Lgr5+ CBC cells display a
pro-inflammatory state under normal conditions (Fig. 4b and Tables S3, S4) are in line with sumoylation acting as a general repressor of the inflammatory response, as shown in myeloid cells
[36]. Inhibition of Ubc9 has been shown to activate pro-inflammatory regulators, such as RelA, cFos, cJun, and IFN-γ in cultured HCT-8 epithelial cells, and to lead to decreased amounts of
the anti-inflammatory IL-10 cytokine in primary intestinal epithelial cells [37]. Lower sumoylation rates, possibly due to enhanced SENP7 desumoylase activity, were also detected in patients
with inflammatory bowel disease and were described as a prerequisite for the onset of inflammation in colons from mice with dextran sodium sulfate- (DSS-) induced colitis [37, 38].
Significantly, previous studies also indicate a link between increased CBC cell numbers, as seen here, and inflammation. For example, a small increase in the number of Lgr5+ CBC cells and a
significant rise in BrdU+ proliferating cells per crypt in the large intestine has been described in mice administrated with the inflammatory agent DSS [39]. Similarly, intestinal organoids
treated with low doses of pro-inflammatory IL-22 [40] or the chronic colitis-associated cytokine TNF-α [39], displayed a small but significant increase in the percentage of Lgr5+ CBC cells.
These observations indicate that mild and non-pathogenic levels of inflammation may indeed promote CBC cell renewal, consistent with our results (Figs. 5a, b, S5a) and the known roles of
inflammation in tumorigenesis and tissue regeneration [41, 42]. Conditions, such as Ubc9 haploinsufficiency that increases the number of stem cells together with promoting a pro-inflammatory
state, would thereby also increase the number of cells with tumor-initiating potential, and hence tumorigenesis. Recent findings showing that sumoylation represents a significant barrier to
cell fate change [43, 44] may provide a further explanation for increased CBC cell number (Figs. 5a, b, S5a). While CBC cells dividing symmetrically at the crypt base represent the normal
source of intestinal stem cells for tissue maintenance, mounting evidence indicates that during injury, lost stem cells in the crypt can be replenished from the “+4” “reserve” cell
population [45], from committed secretory progenitor cells [46], from Paneth and enteroendocrine lineage precursors [47, 48] or from early enterocyte lineage (absorptive) cell precursors
[49]. It would thus be interesting to determine if Ubc9 haploinsufficiency, like injury, increases such cellular plasticity leading to increased CBC cell number. Moreover, in this scenario,
this may also contribute to a second function, enhanced inflammatory signaling, shown necessary for the conversion of such non-stem cells to cells with tumor-initiating potential [50].
Finally, our finding of a tumor-suppressive function of sumoylation appears at odds with previously established roles of sumoylation in cancer. Indeed, the pro-tumorigenic role of
sumoylation is inferred from the enhanced levels of SUMO pathway enzymes, and hence, sumoylation dynamics, and from the accrued sensitivity to inhibition of global sumoylation seen in many
cancer cells [reviewed in ref. 7]. It must be borne in mind, however, that, as shown here, Ubc9 haploinsufficiency increases the frequency of tumor initiation events, as seen by the
increased number of polyps. Indeed, under normal (_Apc__+/+_) conditions, reduced (i.e., haploinsufficient) Ubc9 activity appears sufficient to ensure cell functionality. However, when cells
face stress, such as loss of Apc tumor suppressor activity, this may become a handicap for maintaining the controlled nonmalignant state, in line also with the observed cancer-related
transcriptome signature. Together, our findings support a view of sumoylation as a buffering system against stress. As such, this system must first be overcome (aided experimentally by Ubc9
haploinsufficiency) during the initiation phase of tumorigenesis, to be then rewired, or even enhanced, to serve in mechanisms that ultimately protect the cancerous state. Given the
“druggability” of the SUMO pathway [16], deeper understanding of these dose- and cell type dependent, seemingly paradoxical consequences of targeting the SUMO system may have important
therapeutic implications. MATERIALS AND METHODS MICE _Ubc9__+/−_ [10] and _Ubc9__f/−__;Rosa26Cre__ERT2_ [23] mice have been described previously. _Ubc9__+/−__;Villin-Cre__ERT2__;Apc__f/+_
mice were obtained by crossing _Ubc9__+/−_ with _Villin-Cre__ERT2_ mice [28] and then with _Apc_f/+ mice [27]. _Ubc9__+/−__;Lgr5-IRES-EGFP-Cre__ERT2_ animals were obtained by crossing
_Ubc9__+/−_ with _Lgr5-IRES-EGFP-Cre__ERT2_ mice [21]. _Ubc9__+/−__;Lgr5-IRES-EGFP-Cre__ERT2__;Apc__f/+_ mice were created by crossing _Ubc9__+/−__;Lgr5-IRES-EGFP-Cre__ERT2_ with _Apc__f/+_
mice [27]. See Supplementary Information for details. HISTOLOGY Paraffin-embedded 4 µm sections were stained using H&E solution and blindly analyzed by a pathologist. See Supplementary
Information for details. CELL CULTURE AND GROWTH OF TRANSFORMED MEFS MEFs were obtained from three _Ubc9__f/−__;Rosa26Cre__ERT2_ or _Ubc9_+/+_;Rosa26Cre__ERT2_ embryos at day E12.5 and
pooled. Low-passage _Rosa26-Cre__ERT2_ p53DN and HRASV12-transformed MEFs were treated with 4-hydroxytamoxifen (4-OHT; Sigma-Aldrich) or ethanol (EtOH) as control for the indicated time for
growth experiments and were also used for 2D plate colony formation (low density), foci formation (high density), and 3D soft-agar colony formation assays. See Supplementary Information for
details. HOMOLOGOUS RECOMBINATION REPORTER ASSAY HR was determined as described previously [51] using a DR-GFP reporter stably integrated into U2OS human osteosarcoma cells. See
Supplementary Information for details. WESTERN BLOTTING Protein extracts from cultured cells were obtained by lysing the cells directly in Laemmli buffer (Bio-Rad, Hercules CA, USA). Protein
extracts from tissues were obtained by immersing the tissue in RIPA buffer in Lysing Matrix D-containing tubes (MP Biomedicals, Illkirch, France). See Supplementary Information for details.
FLOW CYTOMETRY AND FLUORESCENCE-ACTIVATED CELL SORTING (FACS) Analysis of cell cycle and apoptosis in transformed MEFs was carried out using the Muse Cell Analyzer (Millipore, Hayward CA,
USA) and the Muse Cell Cycle and Muse Annexin V and Dead Cell kits, respectively (Luminex, Austin TX, USA). Purification of _Lgr5-EGFP__+_ CBC cells from normal non-treated
_Ubc9;Lgr5-IRES-EGFP-Cre__ERT2_ mice and preparation of villi- and crypt-enriched populations were performed adapting previously-described protocols [30, 52]. Single EGFPhigh, CD24middle,
EpCampositive, and PInegative CBC cells were sorted using MoFlo Astrios Cell Sorter (Beckman Coulter). See Supplementary Information for details. QUANTITATIVE PCR RNA was purified using
Trizol (Molecular Research Center, Cincinnati OH, USA) according to manufacturer’s recommendations. Quantitative real-time PCR was performed using the primer sets specified in Supplementary
Table S6. TRANSCRIPTOME PROFILING RNA profiling was performed using GeneChip MoGene 2.0 ST Array (Applied Biosystems) and normalized and analyzed using open-source Bioconductor packages on R
[53, 54]. Differentially-expressed genes (adj. _p_ value (FDR) < 0.05 and |log2FC| > 1.5) were used to challenge curated databases [55,56,57,58,59,60,61]. For GSEA analysis, all
differentially and non-differentially-expressed genes in FACS-purified EGFPhigh CBC cells were queried against a previously published intestinal stem cell signature [30]. See Supplementary
Information for details. DETECTION OF LGR5-EGFP+ CBC CELLS Cryo-sections (50 μm thick) of intestines from _Ubc9;Lgr5-EGFP-IRES-Cre__ERT2_ were analyzed for EGFP and Lysozyme expression by
confocal microscopy and blindly analyzed using Fiji (ImageJ). See Supplementary Information for details. STATISTICS Cell-based experiments were carried out with at least three biological
replicates. Experiments involving mice were performed in at least six animals per group. Statistical analysis was performed with GraphPad Prism 8 software. See Supplementary Information for
details. DATA AVAILABILITY Transcriptomic data from this study were deposited in the GEO database (https://www.ncbi.nlm.nih.gov/geo/) under accession numbers GSE146106 (Fig. 4) and GSE146039
(Fig. S2). CODE AVAILABILITY Computer code can be accessed upon request. REFERENCES * Flotho A, Melchior F. Sumoylation: a regulatory protein modification in health and disease. Annu Rev
Biochem. 2013;82:357–85. CAS PubMed Google Scholar * Hendriks IA, Vertegaal AC. A comprehensive compilation of SUMO proteomics. Nat Rev Mol Cell Biol. 2016;17:581–95. CAS PubMed Google
Scholar * Golebiowski F, Matic I, Tatham MH, Cole C, Yin Y, Nakamura A, et al. System-wide changes to SUMO modifications in response to heat shock. Sci Signal. 2009;2:ra24. PubMed Google
Scholar * Stankovic-Valentin N, Melchior F. Control of SUMO and Ubiquitin by ROS: signaling and disease implications. Mol Asp Med. 2018;63:3–17. CAS Google Scholar * Jackson SP, Durocher
D. Regulation of DNA damage responses by ubiquitin and SUMO. Mol Cell. 2013;49:795–807. CAS PubMed Google Scholar * Bettermann K, Benesch M, Weis S, Haybaeck J. SUMOylation in
carcinogenesis. Cancer Lett. 2012;316:113–25. CAS PubMed Google Scholar * Seeler JS, Dejean A. SUMO and the robustness of cancer. Nat Rev Cancer. 2017;17:184–97. CAS PubMed Google
Scholar * Seufert W, Futcher B, Jentsch S. Role of a ubiquitin-conjugating enzyme in degradation of S- and M-phase cyclins. Nature. 1995;373:78–81. CAS PubMed Google Scholar * Hayashi T,
Seki M, Maeda D, Wang W, Kawabe Y, Seki T, et al. Ubc9 is essential for viability of higher eukaryotic cells. Exp Cell Res. 2002;280:212–21. CAS PubMed Google Scholar * Nacerddine K,
Lehembre F, Bhaumik M, Artus J, Cohen-Tannoudji M, Babinet C, et al. The SUMO pathway is essential for nuclear integrity and chromosome segregation in mice. Dev Cell. 2005;9:769–79. CAS
PubMed Google Scholar * Neyret-Kahn H, Benhamed M, Ye T, Le Gras S, Cossec JC, Lapaquette P, et al. Sumoylation at chromatin governs coordinated repression of a transcriptional program
essential for cell growth and proliferation. Genome Res. 2013;23:1563–79. CAS PubMed PubMed Central Google Scholar * Luo J, Solimini NL, Elledge SJ. Principles of cancer therapy:
oncogene and non-oncogene addiction. Cell. 2009;136:823–37. CAS PubMed PubMed Central Google Scholar * Nagel R, Semenova EA, Berns A. Drugging the addict: non-oncogene addiction as a
target for cancer therapy. EMBO Rep. 2016;17:1516–31. CAS PubMed PubMed Central Google Scholar * Kessler JD, Kahle KT, Sun T, Meerbrey KL, Schlabach MR, Schmitt EM, et al. A
SUMOylation-dependent transcriptional subprogram is required for Myc-driven tumorigenesis. Science. 2012;335:348–53. CAS PubMed Google Scholar * Yu B, Swatkoski S, Holly A, Lee LC, Giroux
V, Lee CS, et al. Oncogenesis driven by the Ras/Raf pathway requires the SUMO E2 ligase Ubc9. Proc Natl Acad Sci USA. 2015;112:E1724–33. CAS PubMed PubMed Central Google Scholar * He X,
Riceberg J, Soucy T, Koenig E, Minissale J, Gallery M, et al. Probing the roles of SUMOylation in cancer cell biology by using a selective SAE inhibitor. Nat Chem Biol. 2017;13:1164–71. CAS
PubMed Google Scholar * Clevers H. The intestinal crypt, a prototype stem cell compartment. Cell. 2013;154:274–84. CAS PubMed Google Scholar * Kinzler KW, Vogelstein B. Lessons from
hereditary colorectal cancer. Cell. 1996;87:159–70. CAS PubMed Google Scholar * Pino MS, Chung DC. The chromosomal instability pathway in colon cancer. Gastroenterology. 2010;138:2059–72.
CAS PubMed Google Scholar * Jackstadt R, Sansom OJ. Mouse models of intestinal cancer. J Pathol. 2016;238:141–51. PubMed Google Scholar * Barker N, van Es JH, Kuipers J, Kujala P, van
den Born M, Cozijnsen M, et al. Identification of stem cells in small intestine and colon by marker gene Lgr5. Nature. 2007;449:1003–7. CAS PubMed Google Scholar * Barker N, Ridgway RA,
van Es JH, van de Wetering M, Begthel H, van den Born M, et al. Crypt stem cells as the cells-of-origin of intestinal cancer. Nature. 2009;457:608–11. CAS PubMed Google Scholar * Demarque
MD, Nacerddine K, Neyret-Kahn H, Andrieux A, Danenberg E, Jouvion G, et al. Sumoylation by Ubc9 regulates the stem cell compartment and structure and function of the intestinal epithelium
in mice. Gastroenterology. 2011;140:286–96. CAS PubMed Google Scholar * Alvarez A, Barisone GA, Diaz E. Focus formation: a cell-based assay to determine the oncogenic potential of a gene.
J Vis Exp. 2014. https://doi.org/10.3791/51742. * Borowicz S, Van Scoyk M, Avasarala S, Karuppusamy Rathinam MK, Tauler J, Bikkavilli RK, et al. The soft agar colony formation assay. J Vis
Exp. 2014. https://doi.org/10.3791/51998. * Rafehi H, Orlowski C, Georgiadis GT, Ververis K, El-Osta A, Karagiannis TC. Clonogenic assay: adherent cells. J Vis Exp. 2011.
https://doi.org/10.3791/2573. * Colnot S, Decaens T, Niwa-Kawakita M, Godard C, Hamard G, Kahn A, et al. Liver-targeted disruption of Apc in mice activates beta-catenin signaling and leads
to hepatocellular carcinomas. Proc Natl Acad Sci USA. 2004;101:17216–21. CAS PubMed PubMed Central Google Scholar * el Marjou F, Janssen KP, Chang BH, Li M, Hindie V, Chan L, et al.
Tissue-specific and inducible Cre-mediated recombination in the gut epithelium. Genesis. 2004;39:186–93. CAS PubMed Google Scholar * Fevr T, Robine S, Louvard D, Huelsken J.
Wnt/beta-catenin is essential for intestinal homeostasis and maintenance of intestinal stem cells. Mol Cell Biol. 2007;27:7551–9. CAS PubMed PubMed Central Google Scholar * Muñoz J,
Stange DE, Schepers AG, van de Wetering M, Koo BK, Itzkovitz S, et al. The Lgr5 intestinal stem cell signature: robust expression of proposed quiescent ‘+4’ cell markers. EMBO J.
2012;31:3079–91. PubMed PubMed Central Google Scholar * He TC, Sparks AB, Rago C, Hermeking H, Zawel L, da Costa LT, et al. Identification of c-MYC as a target of the APC pathway.
Science. 1998;281:1509–12. CAS PubMed Google Scholar * Ordonez-Moran P, Dafflon C, Imajo M, Nishida E, Huelsken J. HOXA5 counteracts stem cell traits by inhibiting Wnt signaling in
colorectal cancer. Cancer Cell. 2015;28:815–29. CAS PubMed Google Scholar * Moll R, Zimbelmann R, Goldschmidt MD, Keith M, Laufer J, Kasper M, et al. The human gene encoding cytokeratin
20 and its expression during fetal development and in gastrointestinal carcinomas. Differentiation. 1993;53:75–93. CAS PubMed Google Scholar * Moschos SJ, Jukic DM, Athanassiou C,
Bhargava R, Dacic S, Wang X, et al. Expression analysis of Ubc9, the single small ubiquitin-like modifier (SUMO) E2 conjugating enzyme, in normal and malignant tissues. Hum Pathol.
2010;41:1286–98. CAS PubMed Google Scholar * Tang Z, Li C, Kang B, Gao G, Li C, Zhang Z. GEPIA: a web server for cancer and normal gene expression profiling and interactive analyses.
Nucleic Acids Res. 2017;45:W98–W102. CAS PubMed PubMed Central Google Scholar * Decque A, Joffre O, Magalhaes JG, Cossec JC, Blecher-Gonen R, Lapaquette P, et al. Sumoylation coordinates
the repression of inflammatory and anti-viral gene-expression programs during innate sensing. Nat Immunol. 2016;17:140–9. CAS PubMed Google Scholar * Mustfa SA, Singh M, Suhail A,
Mohapatra G, Verma S, Chakravorty D, et al. SUMOylation pathway alteration coupled with downregulation of SUMO E2 enzyme at mucosal epithelium modulates inflammation in inflammatory bowel
disease. Open Biol. 2017;7 https://doi.org/10.1098/rsob.170024. * Suhail A, Rizvi ZA, Mujagond P, Ali SA, Gaur P, Singh M, et al. DeSUMOylase SENP7-mediated epithelial signaling triggers
intestinal inflammation via expansion of gamma-delta T cells. Cell Rep. 2019;29:3522–38.e3527. CAS PubMed Google Scholar * Bu P, Wang L, Chen KY, Srinivasan T, Murthy PK, Tung KL, et al.
A miR-34a-numb feedforward loop triggered by inflammation regulates asymmetric stem cell division in intestine and colon cancer. Cell Stem Cell. 2016;18:189–202. CAS PubMed PubMed Central
Google Scholar * Lindemans CA, Calafiore M, Mertelsmann AM, O’Connor MH, Dudakov JA, Jenq RR, et al. Interleukin-22 promotes intestinal-stem-cell-mediated epithelial regeneration. Nature.
2015;528:560–4. CAS PubMed PubMed Central Google Scholar * Grivennikov SI, Greten FR, Karin M. Immunity, inflammation, and cancer. Cell. 2010;140:883–99. CAS PubMed PubMed Central
Google Scholar * Karin M, Clevers H. Reparative inflammation takes charge of tissue regeneration. Nature. 2016;529:307–15. CAS PubMed PubMed Central Google Scholar * Cheloufi S, Elling
U, Hopfgartner B, Jung YL, Murn J, Ninova M, et al. The histone chaperone CAF-1 safeguards somatic cell identity. Nature. 2015;528:218–24. CAS PubMed PubMed Central Google Scholar *
Cossec JC, Theurillat I, Chica C, Bua Aguin S, Gaume X, Andrieux A, et al. SUMO safeguards somatic and pluripotent cell identities by enforcing distinct chromatin states. Cell Stem Cell.
2018;23:742–57.e748. CAS PubMed Google Scholar * Tian H, Biehs B, Warming S, Leong KG, Rangell L, Klein OD, et al. A reserve stem cell population in small intestine renders Lgr5-positive
cells dispensable. Nature. 2011;478:255–9. CAS PubMed PubMed Central Google Scholar * van Es JH, Sato T, van de Wetering M, Lyubimova A, Yee Nee AN, Gregorieff A, et al. Dll1+ secretory
progenitor cells revert to stem cells upon crypt damage. Nat Cell Biol. 2012;14:1099–104. PubMed PubMed Central Google Scholar * Buczacki SJ, Zecchini HI, Nicholson AM, Russell R,
Vermeulen L, Kemp R, et al. Intestinal label-retaining cells are secretory precursors expressing Lgr5. Nature. 2013;495:65–9. CAS PubMed Google Scholar * Basak O, van de Born M, Korving
J, Beumer J, van der Elst S, van Es JH, et al. Mapping early fate determination in Lgr5+ crypt stem cells using a novel Ki67-RFP allele. EMBO J. 2014;33:2057–68. CAS PubMed PubMed Central
Google Scholar * Tetteh PW, Basak O, Farin HF, Wiebrands K, Kretzschmar K, Begthel H, et al. Replacement of lost Lgr5-positive stem cells through plasticity of their enterocyte-lineage
daughters. Cell Stem Cell. 2016;18:203–13. CAS PubMed Google Scholar * Schwitalla S, Fingerle AA, Cammareri P, Nebelsiek T, Goktuna SI, Ziegler PK, et al. Intestinal tumorigenesis
initiated by dedifferentiation and acquisition of stem-cell-like properties. Cell. 2013;152:25–38. CAS PubMed Google Scholar * Pierce AJ, Johnson RD, Thompson LH, Jasin M. XRCC3 promotes
homology-directed repair of DNA damage in mammalian cells. Genes Dev. 1999;13:2633–8. CAS PubMed PubMed Central Google Scholar * Sato T, Vries RG, Snippert HJ, van de Wetering M, Barker
N, Stange DE, et al. Single Lgr5 stem cells build crypt-villus structures in vitro without a mesenchymal niche. Nature. 2009;459:262–5. CAS PubMed Google Scholar * Carvalho BS, Irizarry
RA. A framework for oligonucleotide microarray preprocessing. Bioinformatics. 2010;26:2363–7. CAS PubMed PubMed Central Google Scholar * Ritchie ME, Phipson B, Wu D, Hu Y, Law CW, Shi W,
et al. limma powers differential expression analyses for RNA-sequencing and microarray studies. Nucleic Acids Res. 2015;43:e47. PubMed PubMed Central Google Scholar * Ashburner M, Ball
CA, Blake JA, Botstein D, Butler H, Cherry JM, et al. Gene ontology: tool for the unification of biology. The Gene Ontology Consortium. Nat Genet. 2000;25:25–9. CAS PubMed PubMed Central
Google Scholar * The Gene Ontology Consortium. The Gene Ontology resource: 20 years and still GOing strong. Nucleic Acids Res. 2019;47:D330–8. Google Scholar * Fabregat A, Jupe S, Matthews
L, Sidiropoulos K, Gillespie M, Garapati P, et al. The reactome pathway knowledgebase. Nucleic Acids Res. 2018;46:D649–55. CAS PubMed Google Scholar * Kanehisa M, Furumichi M, Tanabe M,
Sato Y, Morishima K. KEGG: new perspectives on genomes, pathways, diseases and drugs. Nucleic Acids Res. 2017;45:D353–61. CAS PubMed Google Scholar * Ogata H, Goto S, Sato K, Fujibuchi W,
Bono H, Kanehisa M. KEGG: Kyoto Encyclopedia of Genes and Genomes. Nucleic Acids Res. 1999;27:29–34. CAS PubMed PubMed Central Google Scholar * Subramanian A, Tamayo P, Mootha VK,
Mukherjee S, Ebert BL, Gillette MA, et al. Gene set enrichment analysis: a knowledge-based approach for interpreting genome-wide expression profiles. Proc Natl Acad Sci USA.
2005;102:15545–50. CAS PubMed PubMed Central Google Scholar * Chen J, Bardes EE, Aronow BJ, Jegga AG. ToppGene Suite for gene list enrichment analysis and candidate gene prioritization.
Nucleic Acids Res. 2009;37:W305–311. CAS PubMed PubMed Central Google Scholar Download references ACKNOWLEDGEMENTS This work was supported by grants from INCa, LNCC (Equipe labellisée),
Odyssey, ANR and ERC-AdG “SUMOSTRESS” and “SUMiDENTITY” to AD, and by the Josef Steiner Cancer Foundation and ERC-CoG “Cancer Recurrence” to JvR. IL was supported by Fondation ARC
(PDF20170505624). We thank Dr. Giulia Nigro and the Institut Pasteur UTechS facility for technical input and Drs. Bob Weinberg, Maria Jasin, and Sérgio F. de Almeida for providing reagents.
AUTHOR INFORMATION Author notes * Eleftheria Chalatsi Present address: Bio-Rad Laboratories, Marnes-la-Coquette, France * Juan P. Cerapio Present address: Centre de Recherches en
Cancérologie de Toulouse, Université de Toulouse, Toulouse, France * These authors contributed equally: Ignacio López, Eleftheria Chalatsi AUTHORS AND AFFILIATIONS * Nuclear Organization and
Oncogenesis Unit, INSERM U993, Equipe Labellisée Ligue Nationale Contre le Cancer, Institut Pasteur, 75015, Paris, France Ignacio López, Eleftheria Chalatsi, Alexandra Andrieux,
Pierre-François Roux, Juan P. Cerapio, Jacob-S. Seeler & Anne Dejean * Collège Doctoral, Sorbonne Université, 75005, Paris, France Eleftheria Chalatsi * Division of Molecular Pathology,
Oncode Institute, The Netherlands Cancer Institute, Amsterdam, The Netherlands Saskia I. J. Ellenbroek & Jacco van Rheenen * Experimental Neuropathology Unit, Institut Pasteur, 75015,
Paris, France Grégory Jouvion Authors * Ignacio López View author publications You can also search for this author inPubMed Google Scholar * Eleftheria Chalatsi View author publications You
can also search for this author inPubMed Google Scholar * Saskia I. J. Ellenbroek View author publications You can also search for this author inPubMed Google Scholar * Alexandra Andrieux
View author publications You can also search for this author inPubMed Google Scholar * Pierre-François Roux View author publications You can also search for this author inPubMed Google
Scholar * Juan P. Cerapio View author publications You can also search for this author inPubMed Google Scholar * Grégory Jouvion View author publications You can also search for this author
inPubMed Google Scholar * Jacco van Rheenen View author publications You can also search for this author inPubMed Google Scholar * Jacob-S. Seeler View author publications You can also
search for this author inPubMed Google Scholar * Anne Dejean View author publications You can also search for this author inPubMed Google Scholar CONTRIBUTIONS IL, EC, and AD planned the
studies. IL and EC performed most of the experiments and analyzed the data. SIJE analyzed the Lgr5+ CBC cell content by immunofluorescence and interpreted the data with JvR. AA contributed
to the mouse studies. P-FR and JPC performed the transcriptomic analysis, GJ the histological analysis. IL, J-SS and AD analyzed data and wrote the paper with input from JvR and SIJE.
CORRESPONDING AUTHORS Correspondence to Jacob-S. Seeler or Anne Dejean. ETHICS DECLARATIONS CONFLICT OF INTEREST The authors declare that they have no conflict of interest. ETHICAL APPROVAL
Mice were bred at the Pasteur Institute animal facility in specific-pathogen-free conditions and studies were conducted in accordance with institutional guidelines and French and European
law (Institut Pasteur animal experimentation ethics committee approval number 2016-0025). ADDITIONAL INFORMATION PUBLISHER’S NOTE Springer Nature remains neutral with regard to
jurisdictional claims in published maps and institutional affiliations. SUPPLEMENTARY INFORMATION SUPPLEMENTARY METHODS AND LEGENDS SUPPLEMENTARY FIGURE S1 SUPPLEMENTARY FIGURE S2
SUPPLEMENTARY FIGURE S3 SUPPLEMENTARY FIGURE S4 SUPPLEMENTARY FIGURE S5 SUPPLEMENTARY TABLE S1 SUPPLEMENTARY TABLE S2 SUPPLEMENTARY TABLE S3 SUPPLEMENTARY TABLE S4 SUPPLEMENTARY TABLE S5
SUPPLEMENTARY TABLE S6 RIGHTS AND PERMISSIONS OPEN ACCESS This article is licensed under a Creative Commons Attribution 4.0 International License, which permits use, sharing, adaptation,
distribution and reproduction in any medium or format, as long as you give appropriate credit to the original author(s) and the source, provide a link to the Creative Commons license, and
indicate if changes were made. The images or other third party material in this article are included in the article’s Creative Commons license, unless indicated otherwise in a credit line to
the material. If material is not included in the article’s Creative Commons license and your intended use is not permitted by statutory regulation or exceeds the permitted use, you will
need to obtain permission directly from the copyright holder. To view a copy of this license, visit http://creativecommons.org/licenses/by/4.0/. Reprints and permissions ABOUT THIS ARTICLE
CITE THIS ARTICLE López, I., Chalatsi, E., Ellenbroek, S.I.J. _et al._ An unanticipated tumor-suppressive role of the SUMO pathway in the intestine unveiled by Ubc9 haploinsufficiency.
_Oncogene_ 39, 6692–6703 (2020). https://doi.org/10.1038/s41388-020-01457-y Download citation * Received: 23 March 2020 * Revised: 20 August 2020 * Accepted: 04 September 2020 * Published:
18 September 2020 * Issue Date: 22 October 2020 * DOI: https://doi.org/10.1038/s41388-020-01457-y SHARE THIS ARTICLE Anyone you share the following link with will be able to read this
content: Get shareable link Sorry, a shareable link is not currently available for this article. Copy to clipboard Provided by the Springer Nature SharedIt content-sharing initiative