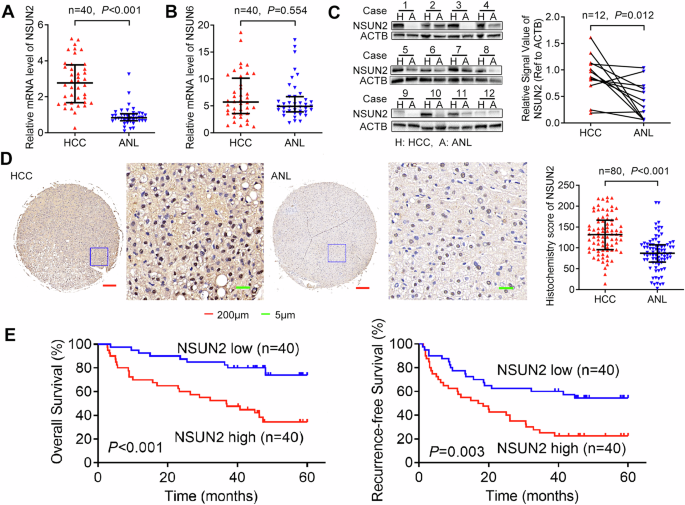
The rna m5c methyltransferase nsun2 promotes progression of hepatocellular carcinoma by enhancing pkm2-mediated glycolysis
- Select a language for the TTS:
- UK English Female
- UK English Male
- US English Female
- US English Male
- Australian Female
- Australian Male
- Language selected: (auto detect) - EN
Play all audios:

ABSTRACT Hepatocellular carcinoma (HCC) is one of the most common cancers worldwide. The 5-methylcytosine (m5C) RNA methyltransferase NSUN2 is involved in cell proliferation and metastasis
and is upregulated in a variety of cancers. However, the biological function and regulatory mechanism of NSUN2-mediated m5C modification have not been well studied in HCC. Our results showed
that NSUN2 is upregulated and associated with poor prognosis in HCC patients after hepatectomy. NSUN2 overexpression significantly promoted HCC growth and metastasis, whereas NSUN2
knockdown had the opposite effect. m5C RNA immunoprecipitation sequencing (m5C-RIP-Seq) revealed that m5C hypermethylation correlates with mRNA overexpression and that NSUN2-mediated m5C
hypermethylation promotes metabolism in HCC patients. Mechanistically, our data revealed that PKM2, a terminal enzyme in the glycolytic pathway, is a downstream target of NSUN2-mediated m5C
modification. Specifically, NSUN2 could stabilize PKM2 mRNA by increasing the m5C level of the m5C site C773 in the 3′-UTR of PKM2 mRNA. In addition, rescue assays revealed that NSUN2
promotes HCC glycolysis and progression by upregulating PKM2. In conclusion, this study revealed that NSUN2-mediated m5C modification promotes glycolysis and the progression of
hepatocellular carcinoma by stabilizing PKM2 mRNA, and provides a potential prognostic factor and therapeutic target for HCC patients. SIMILAR CONTENT BEING VIEWED BY OTHERS
METHYLTRANSFERASE LIKE 3 INHIBITION LIMITS INTRAHEPATIC CHOLANGIOCARCINOMA METABOLIC REPROGRAMMING AND POTENTIATES THE EFFICACY OF CHEMOTHERAPY Article 07 July 2023 HEPATOCELLULAR CARCINOMA
CELLS DOWNREGULATE PGAM2 VIA SIRT2-MEDIATED DEACETYLATION MODIFICATION TO ENHANCE AEROBIC GLYCOLYSIS Article Open access 16 May 2025 PRMT1-MEDIATED METHYLATION OF ME2 PROMOTES HEPATOCELLULAR
CARCINOMA GROWTH BY INHIBITING UBIQUITINATION Article Open access 11 November 2024 INTRODUCTION Hepatocellular carcinoma (HCC) is the sixth most common cancer worldwide and the third
leading cause of cancer death worldwide [1]. Surgical treatment, including hepatectomy and liver transplantation, is effective and can increase the chance of survival in HCC patients.
However, owing to metastasis and recurrence after hepatectomy, the 5-year survival rate of HCC patients is still low. By studying the mechanism of the occurrence and development of HCC, it
is possible to discover new biomarkers and find effective therapeutic targets for HCC patients. Thus, further exploration of the molecular mechanism of HCC progression is necessary. To date,
172 types of RNA modifications have been identified [2]. Among them, RNA N6-methyladenosine (m6A) is the most pervasive, and many studies have reported its important role in HCC [3,4,5,6].
5-Methylcytosine (m5C) is another abundant RNA modification and is widespread in ribosomal RNAs (rRNAs), transfer RNAs (tRNAs), messenger RNAs (mRNAs), enhancer RNAs (eRNAs) and a number of
noncoding RNAs [7]. Studies have shown that m5C is widespread in human mRNAs [8]. Some researchers have reported that there is a significant difference in m5C mRNA between HCC and adjacent
noncancerous liver (ANL) tissues [9]. However, the authors did not conclude that the m5C level was upregulated or downregulated in HCC [9], and the functional role of m5C in the occurrence
and development of HCC is still unclear. Among the eight kinds of human RNA m5C methyltransferases (NSUN1-7 and TRDMT1), only NSUN2 and NSUN6 have been reported to methylate mRNAs [7, 10].
NOP2/SUN domain family member 2 (NSUN2), an RNA methyltransferase, can introduce m5C into cytoplasmic tRNAs and mRNAs and plays indispensable roles in phosphorylation, protein synthesis,
cell cycle progression, epidermal differentiation and tumorigenesis [11]. A previous study revealed that NSUN2 is highly expressed in a variety of tumours, and is related to the occurrence
and development of tumours [12]. Recently, Sun et al. reported that NSUN2 could promote the proliferation and invasion of HepG2 cells via m5C modification of the long noncoding RNA
(lncRNA)-H19 [13]. However, the clinical significance of NSUN2 expression in HCC patients, whether NSUN2 can affect HCC progression through m5C-modified mRNAs, and the underlying mechanism
remain largely unknown. In the present study, we utilised 125 pairs of HCC and corresponding ANL tissues and The Cancer Genome Atlas (TCGA) data to investigate the expression levels of the
m5C RNA methyltransferase NSUN2 in HCC. We demonstrated that NSUN2 is upregulated and associated with poor prognosis in HCC patients and promotes HCC growth and metastasis both in vitro and
in vivo. In addition, we used m5C RNA immunoprecipitation sequencing (m5C-RIP-seq) to investigate the distribution of m5C methylation in HCC mRNAs. The results revealed that m5C was
frequently hypermethylated in HCC and that both upregulated mRNAs and m5C-modified mRNAs were involved mainly in metabolism-associated signalling pathways. Furthermore, we revealed that
NSUN2-mediated m5C modification could promote glycolysis and the progression of HCC by upregulating PKM2, which provides a potential prognostic factor and therapeutic target for HCC patients
and is bound to constitute a breakthrough in clinical and experimental research. METHODS PATIENTS AND SAMPLES In total, 125 pairs of HCC and corresponding ANL tissues (Cohorts 1, 2 and 3),
which were obtained from at least 2 cm away from the tumour border and were proven to lack tumour cells by microscopy, were obtained from surgical resections of patients without preoperative
treatment at Eastern Hepatobiliary Surgery Hospital (Shanghai, China). Human specimen collection was approved by the Ethics Committee of Eastern Hepatobiliary Surgery Hospital. Written
informed consent was obtained from each patient according to the policies of the committee. The resected samples were independently identified by two pathologists. The samples used for RNA-
sequencing, RT-qPCR and western blot analysis were freshly frozen, and the samples used for immunohistochemistry (IHC) were formalin-fixed and paraffin-embedded. Among them, 40 pairs (Cohort
1) were used for RT-qPCR and western blotting. Eighty pairs (Cohort 2) were subjected to IHC and the correlations between NSUN2 expression and the clinical characteristics of HCC patients
after hepatectomy were analysed. Five pairs (Cohort 3) were used for mRNA m5C dot blotting and m5C-RIP-sequencing. The detailed clinicopathological features are described in Supplementary
Table 1. FOLLOW-UP The patients in Cohort 2 received check-ups every 2–3 months after surgery during the first 24 months and every 3–6 months thereafter until December 2020. Physicians who
were blinded to the study performed the follow-up examinations. Serum AFP levels and abdominal ultrasound examinations were performed every month during the first year after surgery and
every 3–6 months thereafter. Computed tomography and/or magnetic resonance imaging were performed every 3–6 months or when recurrence was suspected. The diagnostic of recurrence was based on
the diagnosis criteria from the AASLD Practice Guidelines (http://www.aasld.org/practiceguidelines/Documents/Bookmarked%20Practice%20Guidelines/HCCUpdate2010.pdf). Once recurrence was
confirmed, further treatment was implemented on the basis of the tumour diameter, the number of tumours, the location of the tumour, and the extent of vessel invasion as well as liver
function and performance status. Recurrence-free survival (RFS) was calculated from the date of tumour resection until the detection of tumour recurrence, death from a cause other than HCC,
or the last follow-up visit. The overall survival (OS) was defined as the length of time between surgery and either the death of the patient or the last follow-up visit. MRNA M5C DOT
BLOTTING Using the PolyATtract® mRNA Isolation System III (Z5300), the mRNAs were extracted from the total RNA of five paired HCC and ANL tissues. The mRNA m5C dot blotting assay was
performed as previously described with some modifications [4, 14, 15]. Before use, the mRNA samples were heated at 80 °C for 2 min and then quenched at 4 °C for 5 min. One hundred nanograms
of mRNA per sample was spotted on a nylon membrane. After being suctioned under negative pressure for ~15 min, the membrane was crosslinked twice under an ultraviolet illuminator and then
soaked in blocking buffer for 30 min at room temperature. The membrane was incubated with m5C antibody (Abcam, ab10805) and diluted with blocking buffer at 4 °C overnight. The use of
anti-IgG pAb-HRP and chemiluminescence reagents (Omni-ECL™, SQ201) followed the manufacturer’s protocols. The signal was detected with ChemiDoc™ and ChemiDoc MP imaging systems (Bio-Rad).
M5C-RIP-SEQ AND M5C-RIP-QPCR mRNA m5C-RIP-seq was performed as previously described [9]. Briefly, the m5C-RIP-seq consisted of RNA extraction, RNA fragmentation, m5C-RIP (Abcam, ab10805),
library construction, sequencing and identification of m5C peaks. DiffReps software [16] was used to identify differentially regulated m5C peaks between HCC and ANL tissues. The m5C peaks
with _P_ ≤ 0.00001 and a fold change ≥2 (or ≤0.5) were considered to be upregulated (or downregulated). The methylation fold enrichment (FE) of each mRNA was subsequently acquired and
subjected to log2 conversion. The logFE value was used for clustering in CLUSTER 3.0 software [17]. The detailed information of the antibodies used in this study is listed in Supplementary
Table 2. m5C-RIP-qPCR was conducted as previously described [18]. Briefly, RNA extraction, RNA fragmentation, m5C-RIP (Abcam, ab10805) and RT-qPCR were performed. The primers used to target
the m5C peak of PKM2 mRNA are listed in Supplementary Table 3. MRNA-SEQ ANALYSIS Paired-end reads were harvested from the Illumina HiSeq 4000 sequencer and were quality controlled by Q30.
After 3′ adaptor-trimming and removal of low-quality reads via cutadapt software (v1.9.3), the high-quality reads were aligned to the human reference genome (UCSC hg19) with HISAT2 software
(v2.0.4). Guided by the Ensembl gtf gene annotation file, Cuffdiff software (v2.2.1, part of Cufflinks) was subsequently used to obtain the FPKMs as the expression profiles of the mRNAs, and
the fold change and _p_ value were calculated on the basis of the FPKMs. Differentially expressed mRNAs were identified with a fold-change cut-off of 2 and a _p_ value cut-off of 0.05.
WESTERN BLOT ANALYSIS Radioimmunoprecipitation assay solution (Beyotime, Shanghai, China) was used to harvest lysates from HCC tissues and cells. Protein samples were run on 4%–20% sodium
dodecyl sulphate-polyacrylamide gel electrophoresis gels, transferred to polyvinylidene fluoride membranes, and blocked with 5% non-fat milk for 1 h. After incubation with primary antibodies
overnight at 4 °C, the membranes were incubated with secondary antibodies for 2 h. Finally, the protein bands were visualised with an enhanced chemiluminescent solution (Beyotime, Shanghai,
China) and detected via a chemiluminescence system (Bio-Rad, USA). The primary antibodies used are listed in Supplementary Table 2. IMMUNOHISTOCHEMISTRY AND TISSUE MICROARRAYS ANALYSIS A
tissue microarray (TMA) was constructed as described previously [19]. IHC was performed on the TMA through a two-step immunoperoxidase technique [20]. The immunohistochemical stains were
assessed by the histochemistry score [21] by three separate observers who had no knowledge of patient characteristics. The antibodies used are listed in Supplementary Table 2. REVERSE
TRANSCRIPTION REACTION AND RT-QPCR Total RNA was isolated with TRIzol reagent (Takara, Dalian, China). Complementary DNA (cDNA) was synthesised via a cDNA synthesis kit (Takara, Dalian,
China), and RT-qPCR of total RNA was performed via a real-time PCR system (Applied Biosystems, USA). The real-time PCRs were performed in triplicate. ACTB was used as an endogenous control.
The relative expression was calculated via the comparative ΔΔCt method. The primer sequences are presented in Supplementary Table 3. CELL LINES HepG2, SNU387, Huh7 and Hep3B cells were
obtained from the Chinese Academy of Sciences Cell Bank and were authenticated by short tandem repeat profiling. Using the LookOut® Mycoplasma PCR Detection Kit (Sigma-Aldrich), we conformed
that all the cell lines used in this study were free of contamination by mycoplasma. The cells were grown in Dulbecco’s modified Eagle’s medium with 10% foetal bovine serum (Gibco BRL). The
cells were maintained in an atmosphere of 5% CO2 in a humidified 37 °C incubator. IN VITRO CELL-BEHAVIOUR ASSAYS For the migration and invasion assays, Transwell filter chambers (Costar,
Corning, NY) were used according to the manufacturer’s instructions. Six random microscopic fields were counted per sample for each group. For the cell-proliferation assays, HCC cells were
seeded in 100 μL of growth medium in 96-well plates for various durations. Cell proliferation was evaluated by measuring cell viability with a Cell Counting Kit-8 assay (Dojindo
Laboratories, Kumamoto, Japan) according to the manufacturer’s instructions. These experiments were repeated at least three times independently. ANIMAL STUDIES The animal experiments in this
study conformed to the Animal Research: Reporting of In Vivo Experiments (ARRIVE) guidelines (http://www.nc3rs.org.uk/arrive-guidelines) and were approved by the Institutional Animal Care
and Use Committee of Shanghai University of Traditional Chinese Medicine (Shanghai, China). All the BALB/c nude mice used in this study were male, 5 weeks old, and purchased from the
Laboratory Animal Resources of the Chinese Academy of Sciences (Beijing, China). The mice were age-matched and then randomised into different groups. The mice were housed in laminar flow
cabinets under specific pathogen-free conditions at room temperature with a 12 h light/dark cycle, with food and water available ad libitum. Subcutaneous xenografts were established in the
bilateral armpits of nude mice via the indicated HCC cells (_n_ = 8 for each group). Tumour development was observed twice weekly with a calliper, and the tumour volume was calculated via
the following formula: (larger diameter × smaller diameter2)/2. The mice were sacrificed 4 weeks later, and pieces of tumour tissue were collected to establish orthotopic implanted models.
For the pulmonary metastatic model, the indicated HCC cells were injected into the tail vein of nude mice (_n_ = 6 for each group). Metastases were detected via the IVIS@ Lumina II system
(Caliper Life Sciences, Hopkinton, MA) 10 min after the intraperitoneal injection of 4.0 mg of luciferin (Gold Biotech) in 50 μl of saline. RNA IMMUNOPRECIPITATION RNA immunoprecipitation
(RIP) experiments were performed via a Magna RIP™ RNA-Binding Protein Immunoprecipitation Kit (Millipore, USA) according to the manufacturer’s instructions. LUCIFERASE REPORTER ASSAY The
PKM-WT or PKM2-Mut plasmid was transfected into NSUN2-overexpressing or NSUN2-silenced HCC cells via Lipofectamine-mediated gene transfer. The relative luciferase activity was normalised to
Renilla luciferase activity 48 h after transfection. ACTINOMYCIN D ASSAY The actinomycin D assay was performed as previously described [22,23,24]. HCC cells were seeded equally in 5 wells in
24-well plates (5 × 104 cells per well). Twenty-four hours later, the cells were exposed to actinomycin D (2 µg/ml, Abcam, ab141058) for 0 h, 3 h or 6 h. After that, the cells were
harvested, and the relative RNA levels were analysed via RT-qPCR and normalised to the values measured in the mock treatment group (the 0 h group). METABOLISM MEASUREMENTS A glucose
colorimetric assay kit (K606-100, BioVision, USA) and a lactate colorimetric assay kit II (K627-100, BioVision, USA) were used to determine glucose uptake and lactate production,
respectively, according to the manufacturers’ protocols. The extracellular acidification rate (ECAR) and glycolytic proton efflux rate (glycoPER) were examined via a Seahorse XF glycolysis
stress test kit (103020-100, Seahorse Bioscience, North Billerica, MA, USA) and a Seahorse XF glycolytic rate assay kit (103344-100, Seahorse Bioscience) according to the manufacturer’s
protocol, respectively, and analysed via a Seahorse XFe 96 Extracellular Flux Analyzer (Seahorse Bioscience). Briefly, for the ECAR, glucose, oligomycin (an oxidative phosphorylation
inhibitor), and 2-deoxyglucose (2-DG, a glycolytic inhibitor) were sequentially added to the wells of a Seahorse XF96 cell culture microplate at the indicated time points. For glycoPER,
rotenone + antimycin A (Rot/AA) and 2-DG were sequentially added to the wells of a Seahorse XF96 cell culture microplate at the indicated time points. STATISTICAL ANALYSIS The investigators
were blinded to group allocation for animal studies. All the statistical analyses were performed via SPSS (v.23.0) and GraphPad Prism (v.8). Differences between groups were examined by
Student’s _t_-test, the Wilcoxon signed-rank test, the Mann–Whitney _U_ test or the chi-square test. Correlations were measured via Spearman correlation analysis. Survival curves were
generated via the Kaplan–Meier method, and differences were assessed via the log-rank test. Statistical significance was indicated by _p_ values less than 0.05. *_P_ < 0.05, **_P_ <
0.01, ***_P_ < 0.001. RESULTS NSUN2 IS UPREGULATED AND ASSOCIATED WITH POOR PROGNOSIS IN HCC PATIENTS AFTER HEPATECTOMY To determine the role of mRNA m5C modification in HCC, we evaluated
the expression levels of two major mRNA m5C methyltransferases, NSUN2 and NSUN6 (the only two known RNA m5C methyltransferases that methylate mRNA [7, 10]), in 40-paired HCC and ANL tissues
via RT-qPCR (Cohort 1). The results revealed that the mRNA level of NSUN2 was increased in HCC (Fig. 1A), whereas that of NSUN6 was no significantly different (Fig. 1B). Furthermore,
western blotting of 12 paired HCC and ANL tissues (randomly selected from Cohort 1) revealed that the protein level of NSUN2 was also upregulated in HCC (Fig. 1C). To determine the clinical
importance of high-NSUN2 expression in HCC, we detected the expression of NSUN2 in another cohort of 80 HCC patients (Cohort 2) with prognostic data via IHC (Fig. 1D). Moreover, NSUN2
protein was overexpressed in HCC. According to the median IHC score of NSUN2 in HCC tissues, we divided the 80 HCC patients into a high-NSUN2-expressing group and a low-NSUN2-expressing
group. We found that higher expression of NSUN2 was correlated with the presence of pathological satellites (_P_ = 0.025), larger tumour size (_P_ = 0.013) and microvascular invasion (_P_ =
0.041) (Table 1). These findings indicate that NSUN2 is related to HCC growth and metastasis. Furthermore, Kaplan–Meier’s survival curves revealed that higher expression of NSUN2 was
associated with lower OS (_P_ < 0.001) and lower RFS (_P_ = 0.003) in HCC patients after hepatectomy (Fig. 1E). In addition, according to the TCGA database in GEPIA2 [25], higher NSUN2
mRNA levels also predict poorer OS in HCC patients (_P_ = 0.023, Supplementary Fig. 1). NSUN2 PROMOTES HCC GROWTH AND METASTASIS IN VITRO AND IN VIVO We then explored whether NSUN2 affects
HCC progression. For verification, we assessed the expression of NSUN2 mRNA in multiple HCC cell lines (Supplementary Fig. 2A). Using lentivirus, NSUN2 was stably overexpressed in the HCC
cell lines HepG2 and SNU387 (Supplementary Fig. 2B, C). We also stably silenced NSUN2 in the HCC cell lines Hep3B and Huh7 via lentivirus-mediated short hairpin RNAs (NSUN2-sh1, NSUN2-sh2
and NSUN2-sh3) (Supplementary Fig. 2B, C). Given that NSUN2-sh2 and NSUN2-sh3 were more effective than NSUN2-sh1 was, NSUN2-sh2 and NSUN2-sh3 were used for further study. CCK-8 and Transwell
assays revealed that forced expression of NSUN2 promoted the growth and metastasis of HCC (Fig. 2A, B) and that NSUN2 depletion inhibited the growth and metastasis of HCC (Fig. 2C, D). To
explore the role of NSUN2 in HCC growth in vivo, the NSUN2-overexpressing SNU387 cells and the corresponding negative control and the NSUN2-silenced (NSUN2-sh2) Hep3B cells and the
corresponding negative control were subcutaneously injected into nude mice. The tumour volume was evaluated every week until the nude mice were sacrificed after 4 weeks. NSUN2 overexpression
significantly promoted the growth of SNU387 cells, and NSUN2 knockdown inhibited the growth of Hep3B cells (Fig. 2E, F). Furthermore, to identify the function of NSUN2 in HCC metastasis in
vivo, we established a lung metastasis model by injecting NSUN2-overexpressing or NSUN2-silenced HCC cells into the tail veins of nude mice. Using an in vivo imaging system, the metastatic
process of the HCC cells in the lung was monitored for 5 weeks. As a result, the upregulation of NSUN2 increased the lung metastasis of HCC cells, whereas the downregulation of NSUN2
decreased lung metastasis (Fig. 2G, H). Overall, NSUN2 could induce HCC growth and metastasis in vitro and in vivo. NSUN2-MEDIATED M5C HYPERMETHYLATION PROMOTES METABOLISM IN HCC We next
explored whether the oncogenic role of NSUN2 is m5C-dependent in HCC. First, to detect the global mRNA m5C level in human HCC, we performed m5C dot blotting in five paired HCC and ANL
tissues (Cohort 3) (Fig. 3A). The global mRNA m5C level in HCC was greater than that in ANL tissues. Furthermore, m5C-RIP-seq of mRNAs from the five pairs of HCC and ANL tissues was
performed to elucidate the transcriptomic m5C profile of HCC. The m5C peaks in each sample are shown in Supplementary Tables 4 and 5. Using BEDTools, we identified 27839 unique m5C peaks in
11986 mRNAs in HCC tissues (Supplementary Table 6) and 26837 unique m5C peaks in 11430 mRNAs in ANL tissues (Supplementary Table 7). Among them, 10684 m5C peaks in 8737 mRNAs were detected
in both HCC and ANL tissues (Fig. 3B, C). The m5C levels of mRNAs in HCC and ANL were compared, and 3156 methylation peaks in 2696 mRNAs in HCC were identified as upregulated (Supplementary
Table 8), whereas 1998 methylation peaks in 1756 mRNAs were detected as downregulated (Supplementary Table 9) (Fig. 3D). Moreover, mRNA-sequencing (mRNA-Seq) of the same cohort revealed 3137
upregulated mRNAs (Supplementary Table 10) and 542 downregulated mRNAs (Supplementary Table 11) in HCC compared with ANL. Taken together, these findings indicate that m5C in mRNAs is
frequently hypermethylated in HCC. The joint analysis of the mRNA m5C-RIP-seq and mRNA-Seq data revealed that the expression of mRNAs in HCC (normalised to that in ANL tissues) was slightly
positively correlated with (Spearman _r_ = 0.316, _P_ < 0.001) their m5C levels (Fig. 3E). Moreover, among the 3156 upregulated m5C peaks, the expression of 499 (15.81%) corresponding
mRNAs was upregulated in HCC, whereas that of only 53 (1.68%) was downregulated in HCC (Fig. 3F). Among the 1998 downregulated m5C peaks, 85 (4.25%) corresponding mRNAs were upregulated in
HCC, and 88 (4.40%) were downregulated in HCC. These results indicate that m5C hypermethylation was positively correlated with mRNA overexpression in HCC. KEGG pathway analyses revealed that
the mRNAs with both upregulated expression and m5C levels were enriched in ten pathways (Fig. 3G). Interestingly, central carbon metabolism in cancer (HK2, MAPK3, MYC, PFKP, PKM2, SLC16A3),
galactose metabolism (B4GALT2, HK2, PFKP) and fructose and mannose metabolism (HK2, PFKFB4, PFKP) are associated with metabolism. Overall, m5C hypermethylation is associated with mRNA
overexpression and metabolism in HCC. PKM2 IS THE MAIN TARGET OF NSUN2-MEDIATED M5C MODIFICATION TO PROMOTE METABOLISM To identify the target mRNAs of NSUN2 in human HCC, we performed
mRNA-sequencing of Hep3B-NSUN2-sh2 cells and the corresponding negative control. After NSUN2 was knocked down, 236 genes were upregulated, and 376 genes were downregulated (Fig. 4A,
Supplementary Table 12). We then overlapped the mRNAs with upregulated expression in HCC, the mRNAs with upregulated m5C levels in HCC, and the mRNAs whose expression was downregulated after
NSUN2 was knocked down in Hep3B cells. A Venn diagram revealed that eleven mRNAs (B3GNT3, CD7, EML2, FOXC1, GDF15, LRP4, MAPT, MCTP1, PKM2, PODXL and SLC1A7) met these criteria (Fig. 4B,
C). We then detected the expression of these eleven mRNAs in 40-paired HCC and ANL tissues and found that seven of them were upregulated in HCC (Fig. 4C). Furthermore, we detected the
expression of these seven mRNAs in HCC cell lines and found that only PKM2 was upregulated after NSUN2 was overexpressed in both HepG2 and SNU387, cells but downregulated after NSUN2 was
silenced in both Hep3B and Huh7 cells (Fig. 4D, E). According to the m5C-RIP-seq results, the upregulated m5C peak of PKM2 mRNA is located at its 3′-UTR (chr15:72491753-72491855, hg19).
m5C-RIP-qPCR targeting this peak validated these results (Fig. 4F). Therefore, we assumed that PKM2 mRNA is the main target of NSUN2. NSUN2 STABILISES PKM2 MRNA BY INCREASING ITS M5C LEVEL
Next, we investigated whether NSUN2 could induce the expression of PKM2 by stabilising PKM2 mRNA through increasing its m5C level. We found that overexpressing NSUN2 slowed the degradation
of PKM2 mRNA, whereas silencing NSUN2 had the opposite effect (Fig. 5A). These findings confirmed that NSUN2 could stabilise PKM2 mRNA. Furthermore, m5C-RIP-qPCR revealed that the m5C level
of PKM2 mRNA was increased after NSUN2 was overexpressed in SNU387 cells but decreased upon NSUN2 knockdown in Hep3B cells (Fig. 5B). To detect the exact m5C site of PKM2, we performed
bisulfite-PCR [13] by using primers targeting the m5C peak of PKM2 and cDNA template reverse transcribed from bisulfite converted RNA. Representative Sanger sequencing chromatograms revealed
that the signal at chr15:72491773 (hg19) (named C773) was mixed with cytosine (‘C’, blue) and thymine (‘T’, red), indicating that m5C was methylated in both the HCC tissues and the cell
lines (Fig. 5C). Using a previously described method [26], we calculated the m5C level in each sample. For example, PCR products from SNU387-NC were subcloned and inserted into the T-easy
vector, and 50 clones were randomly selected and sequenced. Among them, 9 clones were detected as ‘C’, whereas 41 clones were detected as ‘T’. Therefore, the m5C level of C773 in SNU387-NC
was calculated as 18% (9/50). Each sample was detected three times. Using this method, the m5C level of C773 in other samples was also detected (Fig. 5D–F). The m5C level in HCC tissues was
greater than that in ANL tissues (Fig. 5D). Furthermore, the m5C level increased after NSUN2 was overexpressed in SNU387 cells but decreased after NSUN2 was knocked down in Hep3B cells (Fig.
5E). To validate the role of C773 in regulating the expression of PKM2, we performed a luciferase reporter assay using a plasmid with the wild-type 3′-UTR of PKM2 mRNA (PKM2-WT) or with a
mutated C773 (PKM2-Mut). As expected, forced expression of NSUN2 increased the luciferase activity of PKM2-WT but not that of PKM2-Mut (Fig. 5F), while silencing NSUN2 inhibited it,
indicating that the regulatory effect of NSUN2 on PKM2 was dependent on the m5C site C773. In summary, NSUN2 could stabilise PKM2 mRNA by increasing the m5C level of the m5C site C773 in the
3′-UTR of PKM2 mRNA. NSUN2 PROMOTES HCC GLYCOLYSIS AND PROGRESSION BY UPREGULATING PKM2 The Warburg effect is characterised by increased glucose uptake and lactate production [27]. To test
whether NSUN2 could promote the progression of HCC by enhancing the PKM2-mediated Warburg effect, we detected glucose uptake and lactate production after NSUN2 was overexpressed and knocked
down. We found that overexpressing NSUN2 significantly increased glucose uptake and lactate production, while silencing NSUN2 decreased glucose uptake and lactate production in HCC cells
(Fig. 6A, B). Importantly, NSUN2 overexpression increased the ECAR (reflecting overall glycolytic flux), whereas NSUN2 silencing decreased the ECAR of HCC cells (Fig. 6C). GlycoPER
(subtracting CO2-dependent acidification related to respiratory activity) was also performed to examine the glycolytic capacities of the cells after NSUN2 was overexpressed and silenced. The
results showed that overexpressing NSUN2 resulted in a significant increase in glycoPER in HCC cells. Furthermore, silencing NSUN2 resulted in a reduction in the glycoPER in HCC cells (Fig.
6D). We next investigated whether the effect of NSUN2 on HCC was mediated by PKM2. First, we found that siRNA targeting PKM2 could downregulate the expression of the PKM2 tetramer, dimer,
and monomer (the expression ratios of tetramer, dimer and monomer did not change), while overexpressing NSUN2 simultaneously could retard this effect (Fig. 6E). Second, we found that
silencing PKM2 inhibited glucose uptake and lactate production, and this effect was blocked by overexpressing NSUN2 (Fig. 6F, G). Furthermore, silencing PKM2 decreased the ECAR and glycoPER,
whereas overexpressing NSUN2 simultaneously retarded this effect (Fig. 6H, I). In addition, knocking down PKM2 inhibited the growth and invasion of HCC cells, and this effect was blocked by
overexpressing NSUN2 (Fig. 6J–L). In summary, NSUN2 promotes HCC glycolysis and progression by upregulating PKM2. DISCUSSION HCC is the most common type of primary liver cancer, accounting
for 85–90% of all cases, and has a high mortality rate. Currently, progress has been made in the development of methods for the diagnosis and treatment of HCC, but the prevention of HCC
recurrence or metastasis remains a challenge because the understanding of its complex molecular pathogenesis is insufficient. Therefore, new effective molecular diagnostics and therapeutic
targets are urgently needed. In the present study, we investigated not only the effect of NSUN2-mediated m5C modification on HCC growth, but also the correlation of this m5C modification
with the clinical prognosis of patients with HCC, as well as the possible molecular mechanism involved. We found that the upregulation of NSUN2 was associated with elevated mRNA m5C levels
in HCC and was associated with poor prognosis in HCC patients after hepatectomy. We demonstrated for the first time that PKM2 is a target gene of NSUN2-mediated m5C modification and that
NSUN2 can promote HCC progression by enhancing PKM2-mediated glycolysis (Fig. 7). m5C is an RNA modification that has attracted increasing attention. It can dynamically regulate a variety of
biological functions through its regulators and plays an important role in a variety of cancers. Our recent study revealed that the m5C RNA methyltransferase NSUN5 promoted the development
of HCC [28]. NSUN2, also known as MYC-induced SUN-domain-containing protein, is a methyltransferase that catalyses 5-methylcytosine formation in the mRNAs of mammals. Accumulating evidence
has shown that NSUN2-mediated mRNA m5C modification plays a vital role in various cancers, such as gastric cancer [29], oesophageal squamous cell carcinoma [30], and bladder cancer [31]. In
the present study, obviously increased NSUN2 was verified in our 40-paired fresh HCC samples and their corresponding adjacent noncancerous liver tissues via qPCR and another independent
80-paired HCC samples and their corresponding adjacent noncancerous liver tissues via TMA IHC staining. Importantly, we found that higher expression of NSUN2 was strongly correlated with
lower OS and lower RFS in HCC patients after hepatectomy in both the TCGA database and our HCC verification cohort. NSUN2 expression, along with several clinicopathological indicators, such
as pathological satellites, larger tumour size and the presence of microvascular invasion, are important prognostic factors for predicting the OS rate and RFS rate of HCC patients.
Furthermore, the results of functional studies from in vitro CCK-8 and transwell assays and in vivo subcutaneous xenografts and pulmonary metastatic models in nude mice revealed that while
NSUN2 overexpression induced the growth and metastasis of HCC, NSUN2 silencing inhibited growth and metastasis. Taken together, these data indicate that the NSUN2 level is a potential marker
that is strongly correlated with the prognosis of HCC patients. Therefore, further study of the mechanism of NSUN2 in HCC is highly important. To further explore whether the oncogenic role
of NSUN2 is m5C-dependent in HCC, we examined the mRNA m5C level in human HCC and found that the m5C level in HCC tissues was greater than that in ANL tissues. To explore m5C modifications
in HCC, we subsequently performed RNA m5C-RIP-seq to identify candidate RNAs associated with NSUN2. RNA m5C-RIP-seq was performed to identify m5C peaks on mRNAs [9], lncRNAs [32] and
circular RNAs (circRNAs) [33] in human HCC and adjacent tissues. However, the sequencing results were not validated experimentally. Sun et al. reported that NSUN2 could promote the
proliferation and invasion of HepG2 cells via m5C modification of the long lncRNA-H19 [13]. However, the clinical significance of NSUN2 expression in HCC and whether NSUN2 affects the
progression of HCC through m5C-modified mRNAs remain largely unknown. In this study, we found that mRNA m5C is frequently hypermethylated in HCC. As m5C has been reported to stabilise mRNAs
in bladder cancer [31], we sought to analyse whether this approach was applied to HCC. To test this hypothesis, we analysed m5C methylation and transcriptome data and found that the
expression of mRNAs in HCC was slightly positively correlated with m5C levels. Interestingly, KEGG pathway analyses revealed that the mRNAs with both upregulated expression and m5C levels
were correlated with metabolism. The liver is the largest metabolic organ in the human body, and metabolic abnormalities are closely related to the occurrence and development of HCC.
Therefore, we hypothesised that NSUN2 may be involved in the occurrence and development of HCC by regulating the m5C modification of metabolism-related genes. To identify the target mRNAs of
NSUN2 in human HCC, we overlapped the mRNAs with upregulated expression in HCC, the mRNAs with upregulated m5C levels in HCC and the mRNAs whose expression was downregulated after NSUN2 was
knocked down in Hep3B cells. The results revealed that eleven mRNAs met this criterion, and the expression of PKM2, a key enzyme in glucose metabolism, was found to be the most consistent
with this criterion via PCR verification. PKM2, a terminal enzyme in the glycolytic pathway [34], is upregulated and associated with poor prognosis in HCC patients [35]. Several studies have
reported how PKM2 is regulated in HCC. For example, the circRNA circMAT2B can upregulate PKM2 mRNA expression by sponging miR-338-3p [36]. The lncRNA LNCAROD can bind to SRSF3 to induce PKM
switching towards PKM2 and maintain PKM2 level by sponging miR-145-5p [37]. ZFP91 can inhibit PKM splicing, resulting in lower levels of the PKM2 isoform and higher levels of the PKM1
isoform [27]. However, whether PKM2 can be regulated by RNA methylation remains unknown. In this study, we identified an m5C RNA methylation site (chr15:72491773 (hg19)) (named C773) at the
3′-UTR of PKM2 mRNA via m5C-RIP-seq, and m5C-RIP-qPCR targeting this peak validated the result. We tested whether NSUN2 influences the expression of PKM2 by stabilising PKM2 mRNA through
increasing its m5C level. Through actinomycin D and m5C-RIP-qPCR assays, we found that overexpressing NSUN2 could retard the degradation of PKM2 mRNA and increase the m5C level of PKM2 mRNA,
whereas silencing NSUN2 had the opposite effect. Moreover, a luciferase reporter assay indicated that the regulatory effect of NSUN2 on PKM2 was dependent on the m5C site C773. Taken
together, these data, for the first time, show that NSUN2 can stabilise PKM2 mRNA by increasing the m5C level of the m5C site C773 in the 3′-UTR of PKM2 mRNA. Glucose metabolic
reprogramming, characterised by increased glycolysis despite the presence of oxygen (Warburg effect), provides essential metabolic intermediates for biosynthesis, thus promoting the
progression of cancers, including HCC [38, 39]. PKM2 can enhance the Warburg effect by catalysing the conversion of phosphoenolpyruvate and ADP to pyruvate and ATP, thus promoting the
occurrence and metastasis of HCC [40, 41]. These findings prompted us to investigate whether NSUN2 could promote the progression of HCC by enhancing the PKM2-mediated Warburg effect. By
analysing the metabolic phenotype of HCC cells, we found that NSUN2 overexpression increased glucose uptake, lactate production, the ECAR and glycoPER. These findings revealed the underlying
mechanism by which NSUN2-mediated PKM2 regulates HCC glycolysis. By performing further rescue assays, we subsequently confirmed that NSUN2 could promote HCC glycolysis and progression by
upregulating PKM2. CONCLUSIONS In summary, our study revealed the critical role of NSUN2 in HCC progression and confirmed that this role is dependent on its m5C modification. The influence
of NSUN2 on promoting HCC growth and metastasis was further demonstrated in vitro and in vivo. Mechanistically, NSUN2-mediated m5C modification promotes glycolysis and the progression of HCC
by stabilising PKM2 mRNA. These findings suggest that NSUN2-mediated m5C modification plays an important role in HCC and provide potential prognostic factors and therapeutic targets for HCC
patients. DATA AVAILABILITY m5C-RIP-Seq data that support the findings of this study have been deposited in the Gene Expression Omnibus database under accession number GSE278763. RNA-seq
data are available from the NCBI Sequence Read Archive (SRA) database (BioProject Accession: PRJNA1181112, SUB14831975). All other data that support the findings of this study are available
from the corresponding authors upon reasonable request. REFERENCES * Sung H, Ferlay J, Siegel RL, Laversanne M, Soerjomataram I, Jemal A, et al. Global Cancer Statistics 2020: GLOBOCAN
estimates of incidence and mortality worldwide for 36 cancers in 185 countries. CA Cancer J Clin. 2021;71:209–49. Article PubMed Google Scholar * Boccaletto P, Machnicka MA, Purta E,
Piatkowski P, Baginski B, Wirecki TK, et al. MODOMICS: a database of RNA modification pathways. 2017 update. Nucleic Acids Res. 2018;46:D303–7. Article CAS PubMed Google Scholar * You Y,
Wen D, Zeng L, Lu J, Xiao X, Chen Y, et al. ALKBH5/MAP3K8 axis regulates PD-L1+ macrophage infiltration and promotes hepatocellular carcinoma progression. Int J Biol Sci. 2022;18:5001–18.
Article CAS PubMed PubMed Central Google Scholar * Du A, Li S, Zhou Y, Disoma C, Liao Y, Zhang Y, et al. M6A-mediated upregulation of circMDK promotes tumorigenesis and acts as a
nanotherapeutic target in hepatocellular carcinoma. Mol Cancer. 2022;21:109. Article CAS PubMed PubMed Central Google Scholar * Yang Y, Cai J, Yang X, Wang K, Sun K, Yang Z, et al.
Dysregulated m6A modification promotes lipogenesis and development of non-alcoholic fatty liver disease and hepatocellular carcinoma. Mol Ther. 2022;30:2342–53. Article CAS PubMed PubMed
Central Google Scholar * Chen YH, Zhao YC, Chen JR, Peng CH, Zhang YP, Tong RL, et al. ALKBH5 suppresses malignancy of hepatocellular carcinoma via m6A-guided epigenetic inhibition of
LYPD1. Mol Cancer. 2020;19:123. Article CAS PubMed PubMed Central Google Scholar * Bohnsack KE, Höbartner C, Bohnsack MT. Eukaryotic 5-methylcytosine (m5C) RNA methyltransferases:
mechanisms, cellular functions, and links to disease. Genes. 2019;10:102. Article CAS PubMed PubMed Central Google Scholar * Squires JE, Patel HR, Nousch M, Sibbritt T, Humphreys DT,
Parker BJ, et al. Widespread occurrence of 5-methylcytosine in human coding and non-coding RNA. Nucleic Acids Res. 2012;40:5023–33. Article CAS PubMed PubMed Central Google Scholar *
Zhang QY, Zheng QY, Yu X, He Y, Guo W. Overview of distinct 5-methylcytosine profiles of messenger RNA in human hepatocellular carcinoma and paired adjacent non-tumor tissues. J Transl Med.
2020;18:245. Article CAS PubMed PubMed Central Google Scholar * Fang L, Wang W, Li GP, Zhang L, Li J, Gan DW, et al. CIGAR-seq, a CRISPR/Cas-based method for unbiased screening of novel
mRNA modification regulators. Mol Syst Biol. 2020;16:e10025. Article CAS PubMed PubMed Central Google Scholar * Liu JH, Huang T, Chen WY, Ding CH, Zhao TX, Zhao XN, et al.
Developmental mRNA m5C landscape and regulatory innovations of massive m5C modification of maternal mRNAs in animals. Nat Commun. 2022;13:2484. Article CAS PubMed PubMed Central Google
Scholar * Chellamuthu A, Gray SG. The RNA methyltransferase NSUN2 and its potential roles in cancer. Cells. 2020;9:1758. Article CAS PubMed PubMed Central Google Scholar * Sun Z, Xue
SL, Zhang MY, Xu H, Hu XM, Chen SH, et al. Aberrant NSUN2-mediated m5C modification of H19 lncRNA is associated with poor differentiation of hepatocellular carcinoma. Oncogene.
2020;39:6906–19. Article CAS PubMed PubMed Central Google Scholar * Chen LX, Ding J, Wang BX, Chen XW, Ying XJ, Yu ZW, et al. RNA methyltransferase NSUN2 promotes hypopharyngeal
squamous cell carcinoma proliferation and migration by enhancing TEAD1 expression in an m5C-dependent manner. Exp Cell Res. 2021;404:112664. Article CAS PubMed Google Scholar * Cheng JX,
Chen L, Li Y, Cloe A, Yue M, Wei JB, et al. RNA cytosine methylation and methyltransferases mediate chromatin organization and 5-azacytidine response and resistance in leukaemia. Nat
Commun. 2018;9:1163. Article PubMed PubMed Central Google Scholar * Shen L, Shao NY, Liu X, Maze I, Feng J, Nestler EJ. diffReps: detecting differential chromatin modification sites from
ChIP-seq data with biological replicates. PLoS ONE. 2013;8:e65598. Article CAS PubMed PubMed Central Google Scholar * de Hoon MJ, Imoto S, Nolan J, Miyano S. Open source clustering
software. Bioinformatics. 2004;20:1453–4. Article PubMed Google Scholar * Huang T, Chen WY, Liu JH, Gu NN, Zhang R. Genome-wide identification of mRNA 5-methylcytosine in mammals. Nat
Struct Mol Biol. 2019;26:380–8. Article CAS PubMed Google Scholar * Hu L, Lau SH, Tzang CH, Wen JM, Wang W, Xie D, et al. Association of Vimentin overexpression and hepatocellular
carcinoma metastasis. Oncogene. 2004;23:298–302. Article CAS PubMed Google Scholar * Tao QF, Yuan SX, Yang F, Yang S, Yang Y, Yuan JH, et al. Aldolase B inhibits metastasis through
Ten-Eleven Translocation 1 and serves as a prognostic biomarker in hepatocellular carcinoma. Mol Cancer. 2015;14:170. Article PubMed PubMed Central Google Scholar * Budwit-Novotny DA,
McCarty KS, Cox EB, Soper JT, Mutch DG, Creasman WT, et al. Immunohistochemical analyses of estrogen receptor in endometrial adenocarcinoma using a monoclonal antibody. Cancer Res.
1986;46:5419–25. CAS PubMed Google Scholar * Ju A, Shen Y, Yue A. Circ_0011232 contributes to hepatocellular carcinoma progression through miR-503-5p/AKT3 axis. Hepatol Res.
2022;52:532–45. Article CAS PubMed Google Scholar * Ma ZL, Li S, Wang YQ, Zhang JX, Zeng XF. Upregulation of a novel LncRNA AC104958.2 stabilized by PCBP2 promotes proliferation and
microvascular invasion in hepatocellular carcinoma. Exp Cell Res. 2021;407:112791. Article CAS PubMed Google Scholar * Zheng QP, Bao CY, Guo WJ, Li SY, Chen J, Chen B, et al. Circular
RNA profiling reveals an abundant circHIPK3 that regulates cell growth by sponging multiple miRNAs. Nat Commun. 2016;7:11215. Article CAS PubMed PubMed Central Google Scholar * Tang ZF,
Kang BX, Li CW, Chen TX, Zhang ZM. GEPIA2: an enhanced web server for large-scale expression profiling and interactive analysis. Nucleic Acids Res. 2019;47:W556–60. Article CAS PubMed
PubMed Central Google Scholar * Chan TH, Lin CH, Qi L, Fei J, Li Y, Yong KJ, et al. A disrupted RNA editing balance mediated by ADARs (Adenosine DeAminases that act on RNA) in human
hepatocellular carcinoma. Gut. 2014;63:832–43. Article CAS PubMed Google Scholar * Chen D, Wang YJ, Lu RX, Jiang XF, Chen XH, Meng N, et al. E3 ligase ZFP91 inhibits Hepatocellular
Carcinoma Metabolism Reprogramming by regulating PKM splicing. Theranostics. 2020;10:8558–72. Article PubMed PubMed Central Google Scholar * Zhang XW, Wu LY, Liu HR, Huang Y, Qi Q, Zhong
R, et al. NSUN5 promotes progression and predicts poor prognosis in hepatocellular carcinoma. Oncol Lett. 2022;24:439. Article CAS PubMed PubMed Central Google Scholar * Hu YB, Chen
CB, Tong XY, Chen SA, Hu XJ, Pan BJ, et al. NSUN2 modified by SUMO-2/3 promotes gastric cancer progression and regulates mRNA m5C methylation. Cell Death Dis. 2021;12:842. Article CAS
PubMed PubMed Central Google Scholar * Su JC, Wu GD, Ye Y, Zhang JL, Zeng LX, Huang XD, et al. NSUN2-mediated RNA 5-methylcytosine promotes esophageal squamous cell carcinoma progression
via LIN28B-dependent GRB2 mRNA stabilization. Oncogene. 2021;40:5814–28. Article CAS PubMed PubMed Central Google Scholar * Chen X, Li A, Sun BF, Yang Y, Han YN, Yuan X, et al.
5-methylcytosine promotes pathogenesis of bladder cancer through stabilizing mRNAs. Nat Cell Biol. 2019;21:978–90. Article CAS PubMed Google Scholar * He YT, Shi QM, Zhang YZ, Yuan X, Yu
ZJ. Transcriptome-wide 5-methylcytosine functional profiling of long non-coding RNA in hepatocellular carcinoma. Cancer Manag Res. 2020;12:6877–85. Article CAS PubMed PubMed Central
Google Scholar * He YT, Zhang QY, Zheng QY, Yu X, Guo WZ. Distinct 5-methylcytosine profiles of circular RNA in human hepatocellular carcinoma. Am J Transl Res. 2020;12:5719–29. CAS PubMed
PubMed Central Google Scholar * Chen X, Chen SW, Yu DS. Protein kinase function of pyruvate kinase M2 and cancer. Cancer Cell Int. 2020;20:523. Article CAS PubMed PubMed Central
Google Scholar * Zhao R, Li L, Yang JB, Niu QF, Wang H, Qin XD, et al. Overexpression of pyruvate kinase M2 in tumor tissues is associated with poor prognosis in patients with
hepatocellular carcinoma. Pathol Oncol Res. 2020;26:853–60. Article CAS PubMed Google Scholar * Li Q, Pan XX, Zhu DM, Deng ZM, Jiang RQ, Wang XH. Circular RNA MAT2B promotes glycolysis
and malignancy of hepatocellular carcinoma through the miR-338-3p/PKM2 axis under hypoxic stress. Hepatology. 2019;70:1298–316. Article CAS PubMed Google Scholar * Jia GZ, Wang Y, Lin
CJ, Lai SH, Dai HL, Wang ZQ, et al. LNCAROD enhances hepatocellular carcinoma malignancy by activating glycolysis through induction of pyruvate kinase isoform PKM2. J Exp Clin Cancer Res.
2021;40:299. Article CAS PubMed PubMed Central Google Scholar * Liang BB, Jiang Y, Song SH, Jing W, Yang H, Zhao L, et al. ASPP2 suppresses tumour growth and stemness characteristics in
HCC by inhibiting Warburg effect via WNT/β-catenin/HK2 axis. J Cell Mol Med. 2023;27:659–71. Article CAS PubMed PubMed Central Google Scholar * Mossenta M, Busato D, Dal Bo M, Toffoli
G. Glucose metabolism and oxidative stress in hepatocellular carcinoma: role and possible implications in novel therapeutic strategies. Cancers. 2020;12:1668. Article CAS PubMed PubMed
Central Google Scholar * Liu WR, Tian MX, Yang LX, Lin YL, Jin L, Ding ZB, et al. PKM2 promotes metastasis by recruiting myeloid-derived suppressor cells and indicates poor prognosis for
hepatocellular carcinoma. Oncotarget. 2015;6:846–61. Article PubMed Google Scholar * Wong TL, Ng KY, Tan KV, Chan LH, Zhou L, Che N, et al. CRAF methylation by PRMT6 regulates aerobic
glycolysis-driven hepatocarcinogenesis via ERK-dependent PKM2 nuclear relocalization and activation. Hepatology. 2020;71:1279–96. Article CAS PubMed Google Scholar Download references
ACKNOWLEDGEMENTS We would like to thank Doctor Wen Li (Eastern Hepatobiliary Surgery Hospital, Naval Medical University) for her kind help in m5C dot blot assay. The image material for Fig.
7 was obtained from biorender.com (Agreement number: RF27A9MWON). FUNDING This work was supported by the National Natural Science Foundation of China (No. 82002458 and 82102482). AUTHOR
INFORMATION Author notes * These authors contributed equally: Qin Qi, Rui Zhong, Yan Huang, Yong Tang. AUTHORS AND AFFILIATIONS * Yueyang Hospital of Integrated Traditional Chinese and
Western Medicine, Shanghai University of Traditional Chinese Medicine, Shanghai, China Qin Qi, Rui Zhong, Yan Huang, Xiao-wen Zhang, Chun-fang Gao & Lu-yi Wu * International Joint
Research Centre on Purinergic Signalling, Chengdu University of Traditional Chinese Medicine, Chengdu, China Yong Tang * Department of Laboratory Medicine, Changzheng Hospital, Naval Medical
University, Shanghai, China Chang Liu & Lin Zhou * Department of Laboratory Medicine, Eastern Hepatobiliary Surgery Hospital, Naval Medical University, Shanghai, China Chun-fang Gao *
The Third Department of Hepatic Surgery, Eastern Hepatobiliary Surgery Hospital, Naval Medical University, Shanghai, China Jian Yu Authors * Qin Qi View author publications You can also
search for this author inPubMed Google Scholar * Rui Zhong View author publications You can also search for this author inPubMed Google Scholar * Yan Huang View author publications You can
also search for this author inPubMed Google Scholar * Yong Tang View author publications You can also search for this author inPubMed Google Scholar * Xiao-wen Zhang View author publications
You can also search for this author inPubMed Google Scholar * Chang Liu View author publications You can also search for this author inPubMed Google Scholar * Chun-fang Gao View author
publications You can also search for this author inPubMed Google Scholar * Lin Zhou View author publications You can also search for this author inPubMed Google Scholar * Jian Yu View author
publications You can also search for this author inPubMed Google Scholar * Lu-yi Wu View author publications You can also search for this author inPubMed Google Scholar CONTRIBUTIONS Luyi
Wu, Jian Yu and Lin Zhou conceived and designed the study. Qin Qi and Rui Zhong performed the experiments. Yong Tang and Chang Liu prepared the figures and tables. Qin Qi and Xiaowen Zhang
acquired, analysed and interpreted the data. Yan Huang and Jian Yu wrote the manuscript. Chunfang Gao, Jian Yu, Luyi Wu and Lin Zhou revised and improved the manuscript. All authors read and
approved the final manuscript. CORRESPONDING AUTHORS Correspondence to Lin Zhou, Jian Yu or Lu-yi Wu. ETHICS DECLARATIONS COMPETING INTERESTS The authors declare no competing interests.
ETHICS APPROVAL AND CONSENT TO PARTICIPATE Human specimen collection was approved by the Ethics Committee of Eastern Hepatobiliary Surgery Hospital (approval number: EHBHKY2018-02-014).
Written informed consent was obtained from each patient according to the policies of the committee. The animal experiments in this study conformed to the Animal Research: Reporting of In
Vivo Experiments (ARRIVE) guidelines (http://www.nc3rs.org.uk/arrive-guidelines) and were approved by the Institutional Animal Care and Use Committee of Shanghai University of Traditional
Chinese Medicine (Shanghai, China). ADDITIONAL INFORMATION PUBLISHER’S NOTE Springer Nature remains neutral with regard to jurisdictional claims in published maps and institutional
affiliations. Edited by Gennaro Ciliberto SUPPLEMENTARY INFORMATION FULL AND UNCROPPED WESTERN BLOTS SUPPLEMENTARY FIGURES 1 AND 2 AND SUPPLEMENTARY TABLES 1–3 SUPPLEMENTARY TABLES 4–12
RIGHTS AND PERMISSIONS OPEN ACCESS This article is licensed under a Creative Commons Attribution 4.0 International License, which permits use, sharing, adaptation, distribution and
reproduction in any medium or format, as long as you give appropriate credit to the original author(s) and the source, provide a link to the Creative Commons licence, and indicate if changes
were made. The images or other third party material in this article are included in the article’s Creative Commons licence, unless indicated otherwise in a credit line to the material. If
material is not included in the article’s Creative Commons licence and your intended use is not permitted by statutory regulation or exceeds the permitted use, you will need to obtain
permission directly from the copyright holder. To view a copy of this licence, visit http://creativecommons.org/licenses/by/4.0/. Reprints and permissions ABOUT THIS ARTICLE CITE THIS
ARTICLE Qi, Q., Zhong, R., Huang, Y. _et al._ The RNA M5C methyltransferase NSUN2 promotes progression of hepatocellular carcinoma by enhancing PKM2-mediated glycolysis. _Cell Death Dis_ 16,
82 (2025). https://doi.org/10.1038/s41419-025-07414-5 Download citation * Received: 14 December 2023 * Revised: 22 January 2025 * Accepted: 30 January 2025 * Published: 09 February 2025 *
DOI: https://doi.org/10.1038/s41419-025-07414-5 SHARE THIS ARTICLE Anyone you share the following link with will be able to read this content: Get shareable link Sorry, a shareable link is
not currently available for this article. Copy to clipboard Provided by the Springer Nature SharedIt content-sharing initiative