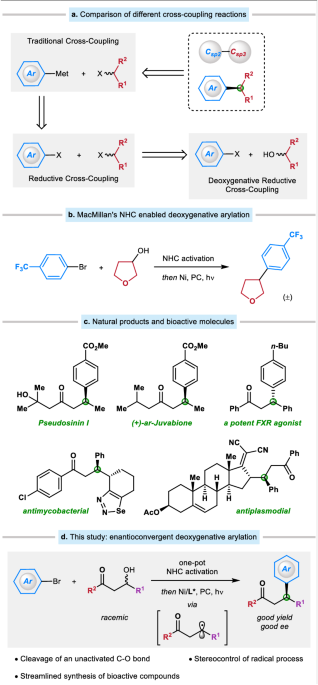
Ni-catalyzed enantioconvergent deoxygenative reductive cross-coupling of unactivated alkyl alcohols and aryl bromides
- Select a language for the TTS:
- UK English Female
- UK English Male
- US English Female
- US English Male
- Australian Female
- Australian Male
- Language selected: (auto detect) - EN
Play all audios:

ABSTRACT Transition metal-catalyzed enantioconvergent cross-coupling of an alkyl precursor presents a promising method for producing enantioenriched C(sp3) molecules. Because alkyl alcohol
is a ubiquitous and abundant family of feedstock in nature, the direct reductive coupling of alkyl alcohol and aryl halide enables efficient access to valuable compounds. Although several
strategies have been developed to overcome the high bond dissociation energy of the C − O bond, the asymmetric pattern remains unknown. In this report, we describe the realization of an
enantioconvergent deoxygenative reductive cross-coupling of unactivated alkyl alcohol (β-hydroxy ketone) and aryl bromide in the presence of an NHC activating agent. The approach can
accommodate substituents of various sizes and functional groups, and its synthetic potency is demonstrated through a gram scale reaction and derivatizations into other compound families.
Finally, we apply our convergent method to the efficient asymmetric synthesis of four β-aryl ketones that are natural products or bioactive compounds. SIMILAR CONTENT BEING VIEWED BY OTHERS
LIGAND-ENABLED NI-CATALYSED ENANTIOCONVERGENT INTERMOLECULAR ALKYL-ALKYL CROSS-COUPLING BETWEEN DISTINCT ALKYL HALIDES Article Open access 22 May 2023 NICKEL-CATALYZED C(SP2)−C(SP3)
CROSS-COUPLING TO ACCESS BENZYL BPINS OF ARYLBORONIC ACIDS WITH Α-HALO BORONIC ESTERS Article Open access 06 May 2025 ENANTIOCONVERGENT AND REGIOSELECTIVE REDUCTIVE COUPLING OF PROPARGYLIC
ESTERS WITH CHLOROGERMANES BY NICKEL CATALYSIS Article 30 October 2023 INTRODUCTION The saturation degree and the presence of chiral centers are two factors that correlate to the successful
transition of a compound from discovery, to clinical testing, and ultimately into a drug1. Transition metal-catalyzed enantioconvergent cross-coupling of an alkyl precursor presents a
promising method for producing these molecules2,3,4,5,6,7. This approach has been shown to be highly effective in forging C(sp3)−C(sp2) bond, particularly when using nickel as the
catalyst8,9,10,11,12,13,14,15,16,17,18. Traditional coupling utilizes alkyl halide and organometallic reagent to form a new C − C bond (Fig. 1a)19,20,21,22. The application of reductive
cross-coupling, led by Weix23,24,25,26,27,28,29, Reisman30,31,32,33,34,35,36,37,38,39,40, and others41,42,43,44,45,46,47,48,49,50,51,52,53,54,55,56,57,58,59,60,61,62,63,64, has proven
beneficial in circumventing the utilization of organometallic reagents that are vulnerable to air and moisture, and in shortening the synthesis with fewer steps. On the other hand, alkyl
halide can be produced through the Appel reaction using alkyl alcohol. Alkyl alcohol is an abundant alkyl source in nature and would be a desirable choice for C(sp3) coupling. However, the
direct cross-coupling of alkyl alcohols remains an underdeveloped field, especially when creating asymmetric patterns65,66,67,68,69. Due to the high bond dissociation energy of the C − O
bond and the low leaving ability of the OH− group70, the direct reductive cross-coupling of alkyl alcohols with aryl halides is elusive. In this vein, many types of alcohol derivatives, such
as alkyl acetates71,72, tosylates73,74,75, xanthate esters76, mesylates77, pivalates78,79, oxalates80,81,82, methyl ethers83, chloroformates84, and others85,86,87, have been extensively
explored in reductive cross-coupling reactions. However, most of these methods are limited to activated alkyl alcohol derivatives, and the pre-activation requires additional steps. In 2018,
Ukaji and co-workers offered an appealing solution by employing a Ti-mediated direct reductive cross-coupling, which only works with primary benzyl alcohols88,89,90,91,92,93,94,95,96,97,98.
An alternative strategy that may be used in certain cases is the one-pot process involving the in situ activation of alkyl alcohols and their subsequent reductive cross-coupling. In this
context, pioneering studies from Li99,100, Gong101, Weix102, Shu103,104,105,106, and others107,108,109,110,111,112,113 have demonstrated the power of this approach owing to the ubiquity of
the two building blocks: free alcohols and aryl bromides. A breakthrough was disclosed by the MacMillan group, who realized an NHC (N-heterocyclic carbene) enabled deoxygenative arylation in
2021 (Fig. 1b)114,115,116,117,118,119. This robust method features mild conditions and simple operations, and targets a broad spectrum of primary, secondary, and tertiary alcohols. Despite
these significant advances, an enantioconvergent deoxygenative reductive cross-coupling of an alkyl alcohol, especially an unactivated alkyl alcohol, still needs to be addressed. Managing
the stereoselective nature of free radical reactions remains a formidable task because of the high reactivity of radical species. β-Hydroxy ketones are a readily available building block
that can be obtained by one-step aldol condensation and contain an unactivated alkyl alcohol group. Furthermore, the carbonyl group is an essential functional group in organic chemistry due
to its versatility in forming a variety of structures and its prevalence in numerous biologically relevant compounds. We speculate that MacMillan’s robust NHC system would serve as an
excellent foundation for achieving the enantioconvergent deoxygenative reductive cross-coupling of β-hydroxy ketones, providing ready access to a wide variety of β-aryl ketones that are a
common subunit found in many natural products and bioactive molecules (Fig. 1c). Herein we describe the realization of this objective by using a chiral nickel/pyridyloxazoline catalyst (Fig.
1d). RESULTS REACTION OPTIMIZATION In an initial study, we examined the coupling of racemic 3-hydroxy-1-phenyl-1-heptanone with methyl 4-bromobenzoate (Table 1). We chose an N-aryl
benzoxazolium salt (NHC) as the activator to convert the alkyl alcohol to an NHC-alcohol adduct in situ. It’s worth noting that this benzoxazolium salt can be easily prepared in the lab on a
hundred-gram scale. After an extensive evaluation of all reaction parameters, we determined that NiBr2·DME and chiral pyridyloxazoline ligand L1 can accomplish the desired enantioconvergent
deoxygenative reductive cross-coupling in good yield and ee (83% yield, 92% ee; entry 1). In the absence of NiBr2·DME, photocatalyst, quinuclidine, light, or ligand L1, essentially no or
only a small amount of product is observed (racemic; entries 2 and 3). The presence of 4-methylpyridine is crucial in the deoxygenative reductive coupling process. The absence of this
additive results in a product with much lower efficiency and selectivity (entry 4). A variety of other chiral ligands are less effective than ligand L1 (entries 5–9). Moreover, the mixed
solvent proves superior to a single solvent (entries 10 and 11). Employing any other base or additive results in a subpar outcome (entries 12 and 13). If the coupling is conducted with less
catalyst, for a shorter time, or at an elevated temperature (r.t.), then a lower yield and/or ee are obtained (entries 14-16). The reaction proceeds relatively smoothly in the presence of a
small amount of air (entry 17), whereas a reaction run with water leads to a diminished yield and ee (entry 18). Under these conditions, the corresponding ester, amide, and phosphonate are
not suitable coupling partners (entry 19). Additionally, substrates like 2-butanol and others that have a functional group at the β-position (-Ph, -OBz, -NHCbz, -NHBz, -NPh2, etc.), were
found to provide much lower yield and/or ee (for a broad exploration of other potential substrates, see Supplementary Fig. 6 and Supplementary Fig. 7), highlighting the critical role of the
ketone moiety as a potential directing group in the cross-coupling reaction120. It is also noteworthy that the MacMillan group employed a similar pyridyloxazoline ligand to successfully
couple alkyl alcohols and alkyl bromides in an achiral/racemic manner, which offers further support to the notion that the ketone moiety used in this study plays a significant role in
enantiocontrol118. SUBSTRATE SCOPE With the optimized reaction conditions in hand, we sought to examine the generality of substrate scope for both coupling partners. This straightforward
method for the catalytic enantioconvergent synthesis of arylated products is compatible with an array of substituents at the β-position (R1; Fig. 2a) of the ketones, providing a range of
products with good yields and high ee. For example, the alkyl substituent at the β-position can vary in size from methyl to neopentyl to isopropyl, and consistently good yields and ee are
observed (products 1–7). A variety of functional groups can be present, including silyl ether, ether, ester, unactivated primary alkyl fluoride/chloride, terminal olefin, and Boc-protected
amine (products 8−18). In reactions involving alcohols with a stereocenter, the catalyst determines the stereochemistry outcome, rather than the substrate (products 19 and 20). Notably, the
presence of an aryl group at the β-position in place of an alkyl group results in a comparable outcome as well (products 21–23). With regard to the groups attached to the carbonyl of the
ketones (R2; Fig. 2b), many aryls prove to be appropriate, including several heteroaryls such as furan, thiophene, and benzothiophene (products 24–36). Furthermore, not only aryl ketones but
also alkyl ketones illustrate superior reactivity and selectivity, and the alkyl size can vary in size from methyl to _tert_-butyl (products 37–41). We next evaluated the scope of aryl
bromides (Ar; Fig. 2c). Under similar conditions, the chiral nickel catalyst couples 1.0 equivalent of racemic β-hydroxy ketone to provide the substitution product with good
enantioselectivity and yield (for example, product 44, 70% yield, 93% ee). The observed values of the enantiomeric excess and yield provide evidence that the coupling reaction works as an
enantioconvergent process. In this context, the catalyst efficiently converts both enantiomers of the racemic alkyl alcohol substrate into a specific stereoisomer of the desired product.
This protocol can efficiently incorporate aryl bromides containing either electron-rich or electron-deficient substituents, complementing previously established Ni-catalyzed reductive
cross-couplings that are typically limited to the electron-deficient aryl halides. Many functional groups, including ester, fluoride/chloride, trifluoromethyl, Bpin, nitrile, and ketone, are
well tolerated in the current system (products 3, 42–55). Unfortunately, the reaction cannot be carried out with _o_-substituted aryl bromides (_o_-F and _o_-Me) due to the increased steric
hindrance. Aryl rings can also be replaced by heteroaryls, including quinoline, benzofuran, and benzothiophene (products 56–58). In reactions involving aryl bromides with one or more
stereocenters, the catalyst determines the stereochemistry outcome instead of the substrate (products 59−62). A reaction on a gram scale (1.22 g of product) yields coupling product 46 with
similar yield and enantiomeric excess as observed in a reaction performed on a 0.50 mmol scale. The absolute configuration of products was unambiguously determined through X-ray diffraction
analysis of compounds 21, 28, and 39. APPLICATIONS AND MECHANISTIC OBSERVATIONS To illustrate the synthetic utility of this method, we have transformed the products into a variety of other
useful enantioenriched compounds (Fig. 3a). For example, β-aryl ketone can be directly transformed in good yields without racemization into terminal olefin, secondary alcohol, aromatic
compound, ester, and amide (products 63–67). Next, we applied our catalytic asymmetric synthesis of β-aryl ketone to a variety of target molecules, starting from commercially available
ketones (Fig. 3b). For example, compound 68, a potent FXR (farnesoid X receptor) agonist analog121, can be prepared in two steps from acetophenone, via an aldol condensation followed by
deoxygenative reductive cross-coupling. Pseudosinin I (69), a sesquiterpenoid obtained from Pseudotsuga sinensis122, can be produced in two steps from diacetone alcohol. (+)-_ar_-Juvabione
(70), generated earlier in four steps via an enantioselective Heck arylation, exhibits juvenile hormone properties123. Using our method, we can obtain β-aryl ketone 70 in two steps and 90%
ee from commercially available building blocks. Another natural product, (-)-dihydro-_ar_-Turmerone (71), which was previously generated in six steps via an asymmetric Michael addition and
Dauben oxidation process, can be synthesized in two steps via our approach124. We have conducted preliminary mechanistic studies of this deoxygenative reductive cross-coupling. In 2022, Zhou
and co-workers presented an elegant method for the enantioselective reductive arylation of α,β-unsaturated ketones using nickel catalyst, which provided a highly efficient approach to
β-aryl ketones125. Mechanistic studies revealed that arylnickel(I) species inserted into enones through 1,4-addition. On the other hand, MacMillan’s research have proposed the formation of
organic radicals derived from alkyl alcohols114. In light of these findings, we are curious about whether our reaction occurs through an enone intermediate or an organic radical species. The
reaction of (_E_)-1-phenylhept-2-en-1-one was examined as the substrate in combination with an aryl bromide, in the absence of both NHC and 2,6-di-_tert_-butylpyridine. Interestingly, the
desired product was successfully obtained, albeit with a slightly lower yield but maintaining the same ee (71% yield, 92% ee; Fig. 4a, entry 1). The introduction of a protic solvent (H2O)
was found to be crucial in Zhou’s reaction. When we switched the mixed solvent system to pure MTBE, the yield for the reaction of enone was much lower compared to that of alcohol (Fig. 4a,
entry 2). This observation indicates the involvement of distinct mechanisms for these two reactions. GC analysis of the model reaction (Table 1) showed that the enone species was generated
in 0.10 equivalent after the initial step of NHC-alcohol adduct preparation, and maintained ~0.10 equiv throughout the entire coupling process. Kinetic studies suggested that the reaction
starting from the alcohol substrate was approximately twice as fast as that starting from the enone substrate (Fig. 4a, right graph). Considering the additional time required for the
formation of enone from the NHC-alcohol adduct, the likelihood of the coupling reaction proceeding through the enone intermediate is diminished. An alternative way to differentiate between
the two pathways is by observing whether an organic radical is produced. Our findings provide evidence in support of the direct deoxygenative radical pathway. For example, if 1 equivalent of
TEMPO (2,2,6,6-tetramethylpiperidin-1-yl)oxyl is added to a coupling in progress, carbon–carbon bond formation essentially ceases. The addition of 2 equivalents of allylic sulfone or vinyl
phosphonate to the model reaction leads to a racemic adduct A1 or A2 without the formation of β-aryl ketone product (Fig. 4b). When the β-hydroxy ketone illustrated in eq 1 (Fig. 4c) was
subjected to the standard coupling conditions, the uncyclized product U was generated with 14% yield and 90% ee, along with the formation of cyclized product C in 65% yield and 1.6:1.0 dr
(both diastereomers are racemic). This dr value is essentially identical to that obtained in an _n_-Bu3SnH-mediated reductive cyclization of the corresponding β-bromo ketone (1.7:1.0; Fig.
4c, eq 2), which is consistent with organic free radical R serving as a common intermediate in both processes. It has been reported that 5-hexenyl radicals cyclize with a rate constant of
~105 s-1 126, while the rate constant for diffusion is typically greater than 108 s-1 127. Thus, the identification of cyclized product C in eq 1 implies that the organic radical persists
long enough to leave the solvent cage. An increase in the U/C ratio was observed with increasing nickel catalyst concentration (Fig. 4c, graph below eq 1), which suggests out-of-cage radical
coupling instead of in-cage radical coupling. In contrast, the corresponding α,β-unsaturated ketone exclusively furnishes uncyclized product U (64% yield, 90% ee; Fig. 4c, eq 3), which
supports a distinct 1,4-addition pathway in the coupling reaction of enone. Taken together, these observations suggest that the deoxygenative reductive cross-coupling reaction predominantly
proceeds via an organic radical intermediate rather than an enone intermediate (for a proposed mechanism, see Supplementary Fig. 12). DISCUSSION We have developed a nickel-catalyzed
enantioconvergent deoxygenative reductive cross-coupling of unactivated alkyl alcohol (β-hydroxy ketone) and aryl bromide in the presence of an NHC activating agent. This scalable method
tolerates substituents of varying sizes on the alcohol, and displays good functional-group tolerance. This approach features the utilization of two readily available coupling partners: alkyl
alcohols and aryl bromides, enabling efficient and modular access to enantioenriched β-aryl ketones including a variety of interesting target molecules. Additional efforts to apply
earth-abundant metals to useful asymmetric coupling reactions are underway in our lab. METHODS GENERAL PROCEDURE FOR ENANTIOCONVERGENT DEOXYGENATIVE REDUCTIVE CROSS-COUPLING OF ALKYL ALCOHOL
AND ARYL BROMIDE (ALKYL ALCOHOL: ARYL BROMIDE = 1.6: 1.0) In a nitrogen-filled glovebox, an oven-dried 4 mL vial that contained a stir bar was charged with NiBr2·DME (8.0 mg, 0.025 mmol,
5.0 mol%), (_S_)-L1 (6.5 mg, 0.030 mmol, 6.0 mol%), and Ir[dF(CF3)ppy]2(dtbbpy)PF6 (9.0 mg, 0.0075 mmol, 1.5 mol%). Anhydrous isopropanol (1.5 mL) was added, and the vial was capped with a
PTFE septum cap. The mixture was stirred at room temperature for 30 min, leading to a laurel-green solution. In a nitrogen-filled glovebox, a separate oven-dried 4 mL vial was charged with
the alkyl alcohol (0.80 mmol, 1.6 equiv), NHC (316.5 mg, 0.80 mmol, 1.6 equiv), and a stir bar. Methyl _tert_-butyl ether (3.5 mL) was added, and the mixture was stirred at room temperature
for 5 min. Next, 2,6-bis(_tert_-butyl) pyridine (179.5 μL, 0.80 mmol, 1.6 equiv) was added dropwise, and the resulting solution was stirred at room temperature for another 30 min (a white
solid precipitated during this time). The suspension was filtered to furnish a homogeneous solution. In a nitrogen-filled glovebox, an oven-dried 20 mL vial was charged with the aryl bromide
(0.50 mmol, 1.0 equiv), quinuclidine (67 mg, 0.60 mmol, 1.2 equiv), and a stir bar. The catalyst solution and NHC-alcohol adduct solution were transferred via syringe to the 20 mL reaction
vial, followed by the addition of 4-methylpyridine (75 μL, 0.75 mmol, 1.5 equiv). The vial was transferred out of the glovebox and placed in an EtOH cooling bath at 10 °C for 5 min. Then the
reaction was irradiated with blue LEDs (455 nm, 30 W) and was stirred at 10 oC for 18 h. The reaction mixture was passed through a plug of silica gel, and the vial, the cap, and the silica
gel were rinsed with EtOAc. The filtrate was concentrated, and the residue was purified by flash chromatography on silica gel. DATA AVAILABILITY The experimental data and the
characterization data for all the compounds generated in this study are provided in the Supplementary Information. Experimental details: general information, preparation of alkyl alcohols,
catalytic enantioconvergent cross-couplings, effect of reaction parameters, cross-couplings of other alkyl alcohols, comparison between the stability of alcohol and bromide, applications,
mechanistic experiments, assignments of absolute configuration, NMR spectra and determination of stereoselectivity (PDF). CCDC 2281455, 2281456, 2281457 contain the supplementary
crystallographic data for this paper. These data can be obtained free of charge via www.ccdc.cam.ac.uk/data_request/cif, or by emailing [email protected], or by contacting The
Cambridge Crystallographic Data Centre, 12 Union Road, Cam-bridge CB2 1EZ, UK; fax: +44 1223 336033. All other data are available from the corresponding author upon request. REFERENCES *
Lovering, F., Bikker, J. & Humblet, C. Escape from flatland: increasing saturation as an approach to Improving clinical success. _J. Med. Chem._ 52, 6752–6756 (2009). Article CAS
PubMed Google Scholar * Geist, E., Kirschning, A. & Schmidt, T. sp3-sp3 Coupling reactions in the synthesis of natural products and biologically active molecules. _Nat. Prod. Rep._ 31,
441–448 (2014). Article CAS PubMed Google Scholar * Cherney, A. H., Kadunce, N. T. & Reisman, S. E. Enantioselective and enantiospecific transition-metal-catalyzed cross-coupling
reactions of organometallic reagents to construct C−C bonds. _Chem. Rev._ 115, 9587–9652 (2015). Article CAS PubMed PubMed Central Google Scholar * Iwasaki, T. & Kambe, N.
Ni-catalyzed C−C couplings using alkyl electrophiles. _Top. Curr. Chem._ 374, 66 (2016). Article Google Scholar * Fu, G. C. Transition-metal catalysis of nucleophilic substitution
reactions: a radical alternative to SN1 and SN2 processes. _ACS Cent. Sci._ 3, 692–700 (2017). Article CAS PubMed PubMed Central Google Scholar * Choi, J. & Fu, G. C. Transition
metal–catalyzed alkyl-alkyl bond formation: another dimension in cross-coupling chemistry. _Science_ 356, eaaf7230 (2017). Article PubMed PubMed Central Google Scholar * Kaga, A. &
Chiba, S. Engaging radicals in transition metal-catalyzed cross-coupling with alkyl electrophiles: recent advances. _ACS Catal._ 7, 4697–4706 (2017). Article CAS Google Scholar * Cheng,
X., Lu, H. & Lu, Z. Enantioselective benzylic C-H arylation via photoredox and nickel dual catalysis. _Nat. Commun._ 10, 3549 (2019). Article ADS PubMed PubMed Central Google Scholar
* Gandolfo, E., Tang, X., Raha Roy, S. & Melchiorre, P. Photochemical asymmetric nickel-catalyzed acyl cross-coupling. _Angew. Chem. Int. Ed._ 58, 16854–16858 (2019). Article CAS
Google Scholar * Shu, X., Huan, L., Huang, Q. & Huo, H. Direct enantioselective C(sp3)–H acylation for the synthesis of α-amino ketones. _J. Am. Chem. Soc._ 142, 19058–19064 (2020).
Article CAS PubMed Google Scholar * Guo, L. et al. General method for enantioselective three-component carboarylation of alkenes enabled by visible-light dual photoredox/nickel
catalysis. _J. Am. Chem. Soc._ 142, 20390–20399 (2020). Article CAS Google Scholar * Cheng, X., Li, T., Liu, Y. & Lu, Z. Stereo- and enantioselective benzylic C–H alkenylation via
photoredox/nickel dual catalysis. _ACS Catal._ 11, 11059–11065 (2021). Article CAS Google Scholar * Chen, J. & Zhu, S. Nickel-catalyzed multicomponent coupling: synthesis of α-chiral
ketones by reductive hydrocarbonylation of alkenes. _J. Am. Chem. Soc._ 143, 14089–14096 (2021). Article CAS PubMed Google Scholar * Shu, X., Zhong, D., Lin, Y., Qin, X. & Huo, H.
Modular access to chiral α-(hetero)aryl amines via Ni/photoredox-catalyzed enantioselective cross-coupling. _J. Am. Chem. Soc._ 144, 8797–8806 (2022). Article CAS PubMed Google Scholar *
Jiang, X., Sheng, F.-T., Zhang, Y., Deng, G. & Zhu, S. Ligand relay catalysis enables asymmetric migratory reductive acylation of olefins or alkyl halides. _J. Am. Chem. Soc._ 144,
21448–21456 (2022). Article CAS PubMed Google Scholar * Li, X. et al. Three-component enantioselective alkenylation of organophosphonates via nickel metallaphotoredox catalysis. _Chem_
9, 154–169 (2023). Article CAS Google Scholar * Xu, S. et al. Enantioselective C(sp3)–H functionalization of oxacycles via photo-HAT/nickel dual catalysis. _J. Am. Chem. Soc._ 145,
5231–5241 (2023). Article CAS PubMed Google Scholar * Du, X., Cheng-Sánchez, I. & Nevado, C. Dual nickel/photoredox-catalyzed asymmetric carbosulfonylation of alkenes. _J. Am. Chem.
Soc._ 145, 12532–12540 (2023). Article CAS PubMed PubMed Central Google Scholar * Choi, J., Martín-Gago, P. & Fu, G. C. Stereoconvergent arylations and alkenylations of unactivated
alkyl electrophiles: catalytic enantioselective synthesis of secondary sulfonamides and sulfones. _J. Am. Chem. Soc._ 136, 12161–12165 (2014). Article CAS PubMed PubMed Central Google
Scholar * Liang, Y. & Fu, G. C. Stereoconvergent negishi arylations of racemic secondary alkyl electrophiles: differentiating between a CF3 and an alkyl group. _J. Am. Chem. Soc._ 137,
9523–9526 (2015). Article CAS PubMed PubMed Central Google Scholar * Huang, W., Wan, X. & Shen, Q. Enantioselective construction of trifluoro methoxylated stereogenic centers by a
nickel-catalyzed asymmetric Suzuki–Miyaura coupling of secondary benzyl bromides. _Angew. Chem. Int. Ed._ 56, 11986–11989 (2017). Article CAS Google Scholar * Varenikov, A. &
Gandelman, M. Organotitanium nucleophiles in asymmetric cross-coupling reaction: stereoconvergent synthesis of chiral α‑CF3 thioethers. _J. Am. Chem. Soc._ 141, 10994–10999 (2019). Article
CAS PubMed Google Scholar * Everson, D. A., Shrestha, R. & Weix, D. J. Nickel-catalyzed reductive cross-coupling of aryl halides with alkyl halides. _J. Am. Chem. Soc._ 132, 920–921
(2010). Article CAS PubMed Google Scholar * Zhao, Y. & Weix, D. J. Nickel-catalyzed regiodivergent opening of epoxides with aryl halides: co-catalysis controls regioselectivity. _J.
Am. Chem. Soc._ 136, 48–51 (2014). Article CAS PubMed Google Scholar * Zhao, Y. & Weix, D. J. Enantioselective cross-coupling of _meso_-epoxides with aryl halides. _J. Am. Chem.
Soc._ 137, 3237–3240 (2015). Article CAS PubMed PubMed Central Google Scholar * Huihui, K. M. M. et al. Decarboxylative cross-electrophile coupling of _N_-hydroxyphthalimide esters with
aryl iodides. _J. Am. Chem. Soc._ 138, 5016–5019 (2016). Article CAS PubMed PubMed Central Google Scholar * Kim, S., Goldfogel, M. J., Gilbert, M. M. & Weix, D. J. Nickel-catalyzed
cross-electrophile coupling of aryl chlorides with primary alkyl chlorides. _J. Am. Chem. Soc._ 142, 9902–9907 (2020). Article ADS CAS PubMed PubMed Central Google Scholar * Franke,
M. C. et al. Zinc-free, scalable reductive cross-electrophile coupling driven by electrochemistry in an undivided cell. _ACS Catal._ 12, 12617–12626 (2022). Article CAS PubMed PubMed
Central Google Scholar * Mouat, J. M. et al. CdS quantum dots for metallaphotoredox-enabled cross-electrophile coupling of aryl halides with alkyl halides. _ACS Catal._ 13, 9018–9024
(2023). Article CAS PubMed Google Scholar * Cherney, A. H., Kadunce, N. T. & Reisman, S. E. Catalytic asymmetric reductive acyl cross-coupling: synthesis of enantioenriched acyclic
α,α-disubstituted ketones. _J. Am. Chem. Soc._ 135, 7442–7445 (2013). Article CAS PubMed Google Scholar * Cherney, A. H. & Reisman, S. E. Nickel-catalyzed asymmetric reductive
cross-coupling between vinyl and benzyl electrophiles. _J. Am. Chem. Soc._ 136, 14365–14368 (2014). Article CAS PubMed PubMed Central Google Scholar * Kadunce, N. T. & Reisman, S.
E. Nickel-catalyzed asymmetric reductive cross-coupling between heteroaryl iodides and α‑chloronitriles. _J. Am. Chem. Soc._ 137, 10480–10483 (2015). Article CAS PubMed PubMed Central
Google Scholar * Suzuki, N., Hofstra, J. L., Poremba, K. E. & Reisman, S. E. Nickel-catalyzed enantioselective cross-coupling of _N_-hydroxyphthalimide esters with vinyl bromides. _Org.
Lett._ 19, 2150–2153 (2017). Article CAS PubMed PubMed Central Google Scholar * Poremba, K. E., Kadunce, N. T., Suzuki, N., Cherney, A. H. & Reisman, S. E. Nickel-catalyzed
asymmetric reductive cross-coupling to access 1,1-diarylalkanes. _J. Am. Chem. Soc._ 139, 5684–5687 (2017). Article CAS PubMed PubMed Central Google Scholar * Hofstra, J. L., Cherney,
A. H., Ordner, C. M. & Reisman, S. E. Synthesis of enantioenriched allylic silanes via nickel-catalyzed reductive cross-coupling. _J. Am. Chem. Soc._ 140, 139–142 (2018). Article CAS
PubMed Google Scholar * DeLano, T. J. & Reisman, S. E. Enantioselective electroreductive coupling of alkenyl and benzyl halides via nickel catalysis. _ACS Catal._ 9, 6751–6754 (2019).
Article CAS PubMed PubMed Central Google Scholar * DeLano, T. J. et al. Nickel-catalyzed asymmetric reductive cross-coupling of α-chloroesters with (hetero)aryl iodides. _Chem. Sci._
12, 7758–7762 (2021). Article CAS PubMed PubMed Central Google Scholar * Turro, R. F., Brandstätter, M. & Reisman, S. E. Nickel-catalyzed reductive alkylation of heteroaryl imines.
_Angew. Chem. Int. Ed._ 61, e202207597 (2022). Article ADS CAS Google Scholar * Lacker, C. R. et al. Enantioselective synthesis of _N_-benzylic heterocycles by Ni/photoredox dual
catalysis. _J. Am. Chem. Soc._ 144, 20190–20195 (2022). Article CAS PubMed PubMed Central Google Scholar * Turro, R. F. et al. Mechanistic investigation of Ni-catalyzed reductive
cross-coupling of alkenyl and benzyl electrophiles. _J. Am. Chem. Soc._ 145, 14705–14715 (2023). Article CAS PubMed PubMed Central Google Scholar * Woods, B. P., Orlandi, M., Huang,
C.-Y., Sigman, M. S. & Doyle, A. G. Nickel-catalyzed enantioselective reductive cross-coupling of styrenyl aziridines. _J. Am. Chem. Soc._ 139, 5688–5691 (2017). Article CAS PubMed
Google Scholar * Banerjee, A. & Yamamoto, H. Nickel catalyzed regio-, diastereo-, and enantioselective cross-coupling of 3,4-epoxyalcohol with aryl Iodides. _Org. Lett._ 19, 4363–4366
(2017). Article CAS PubMed Google Scholar * Guan, H., Zhang, Q., Walsh, P. J. & Mao, J. Nickel/photoredox-catalyzed asymmetric reductive cross-coupling of racemic α-chloro esters
with aryl iodides. _Angew. Chem. Int. Ed._ 59, 5172–5177 (2020). Article CAS Google Scholar * Zheng, P. et al. Dual Ni/photoredox-catalyzed asymmetric cross-coupling to access chiral
benzylic boronic esters. _Nat. Commun._ 12, 1646 (2021). Article ADS CAS PubMed PubMed Central Google Scholar * Sun, D., Ma, G., Zhao, X., Lei, C. & Gong, H. Nickel-catalyzed
asymmetric reductive arylation of α-chlorosulfones with aryl halides. _Chem. Sci._ 12, 5253–5258 (2021). Article CAS PubMed PubMed Central Google Scholar * Min, Y. et al. Diverse
synthesis of chiral trifluoromethylated alkanes via nickel-catalyzed asymmetric reductive cross-coupling fluoroalkylation. _Angew. Chem. Int. Ed._ 60, 9947–9952 (2021). Article CAS Google
Scholar * Lau, S. H. et al. Ni/photoredox-catalyzed enantioselective cross-electrophile coupling of styrene oxides with aryl iodides. _J. Am. Chem. Soc._ 143, 15873–15881 (2021). Article
CAS PubMed PubMed Central Google Scholar * Wang, D. & Xu, T. A pivotal role of chloride ion on nickel-catalyzed enantioselective reductive cross-coupling to perfluoroalkylated
boronate esters. _ACS Catal._ 11, 12469–12475 (2021). Article CAS Google Scholar * Wang, H., Zheng, P., Wu, X., Li, Y. & Xu, T. Modular and facile access to chiral α-aryl phosphates
via dual nickel- and photoredox-catalyzed reductive cross-coupling. _J. Am. Chem. Soc._ 144, 3989–3997 (2022). Article CAS PubMed Google Scholar * Wu, B.-B., Xu, J., Bian, K.-J., Gao, Q.
& Wang, X.-S. Enantioselective synthesis of secondary β-trifluoromethyl alcohols via catalytic asymmetric reductive trifluoroalkylation and diastereoselective reduction. _J. Am. Chem.
Soc._ 144, 6543–6550 (2022). Article CAS PubMed Google Scholar * Jin, R.-X. et al. Asymmetric construction of allylic stereogenic carbon center featuring a trifluoromethyl group via
enantioselective reductive fluoroalkylation. _Nat. Commun._ 13, 7035 (2022). Article ADS CAS PubMed PubMed Central Google Scholar * Li, T. et al. Enantioselective reductive
cross-coupling of aryl/alkenyl bromides with benzylic chlorides via photoredox/biimidazoline nickel dual catalysis. _Chin. J. Chem._ 40, 1033–1038 (2022). Article ADS CAS Google Scholar
* Zhou, J., Wang, D., Xu, W., Hu, Z. & Xu, T. Enantioselective C(sp3)-C(sp3) reductive cross-electrophile coupling of unactivated alkyl halides with α-chloroboronates via dual
nickel/photoredox catalysis. _J. Am. Chem. Soc._ 145, 2081–2087 (2023). Article CAS PubMed Google Scholar * Hu, X., Cheng-Sánchez, I., Cuesta-Galisteo, S. & Nevado, C.
Nickel-catalyzed enantioselective electrochemical reductive cross-coupling of aryl aziridines with alkenyl bromides. _J. Am. Chem. Soc._ 145, 6270–6279 (2023). Article CAS PubMed PubMed
Central Google Scholar * Wang, H., Wu, X. & Xu, T. Enantioconvergent reductive C(sp)-C(sp3) cross-coupling to access chiral α-alkynyl phosphonates under dual nickel/photoredox
catalysis. _Angew. Chem. Int. Ed._ 62, e202218299 (2023). Article CAS Google Scholar * Wang, K., Ding, Z., Zhou, Z. & Kong, W. Ni-catalyzed enantioselective reductive diarylation of
activated alkenes by domino cyclization/cross-coupling. _J. Am. Chem. Soc._ 140, 12364–12368 (2018). Article CAS PubMed Google Scholar * Anthony, D., Lin, Q., Baudet, J. & Diao, T.
Nickel-catalyzed asymmetric reductive diarylation of vinylarenes. _Angew. Chem. Int. Ed._ 58, 3198–3202 (2019). Article CAS Google Scholar * Jin, Y. & Wang, C. Nickel-catalyzed
asymmetric reductive arylalkylation of unactivated alkenes. _Angew. Chem. Int. Ed._ 58, 6722–6726 (2019). Article CAS Google Scholar * Tian, Z.-X. et al. Highly Enantioselective
cross-electrophile aryl-alkenylation of unactivated alkenes. _J. Am. Chem. Soc._ 141, 7637–7643 (2019). Article CAS PubMed Google Scholar * He, J. et al. Nickel-catalyzed asymmetric
reductive 1,2-carboamination of unactivated alkenes. _Angew. Chem. Int. Ed._ 59, 2328–2332 (2020). Article CAS Google Scholar * Tu, H.-Y. et al. Enantioselective three-component
fluoroalkylarylation of unactivated olefins through nickel-catalyzed cross-electrophile coupling. _J. Am. Chem. Soc._ 142, 9604–9611 (2020). Article CAS PubMed Google Scholar * Wei, X.,
Shu, W., García-Domínguez, A., Merino, E. & Nevado, C. Asymmetric Ni-catalyzed radical relayed reductive coupling. _J. Am. Chem. Soc._ 142, 13515–13522 (2020). Article CAS PubMed
Google Scholar * Wu, X., Qu, J. & Chen, Y. Quinim: a new ligand scaffold enables nickel-catalyzed enantioselective synthesis of α‑alkylated γ‑lactam. _J. Am. Chem. Soc._ 142,
15654–15660 (2020). Article CAS PubMed Google Scholar * Wu, X. et al. Catalytic desymmetric dicarbofunctionalization of unactivated alkenes. _Angew. Chem. Int. Ed._ 61, e202111598
(2022). Article ADS CAS Google Scholar * Cheng, L., Lin, Q., Chen, Y. & Gong, H. Recent progress on transition-metal-mediated reductive C(sp3)-O bond radical addition and coupling
reactions. _Synthesis_ 54, 4426–4446 (2022). Article CAS Google Scholar * Pang, X., Su, P.-F. & Shu, X.-Z. Reductive cross-coupling of unreactive electrophiles. _Acc. Chem. Res._ 55,
2491–2509 (2022). Article CAS PubMed Google Scholar * Pang, X. & Shu, X.-Z. Reductive deoxygenative functionalization of alcohols by first-row transition metal catalysis. _Chin. J.
Chem._ 41, 1637–1652 (2023). Article CAS Google Scholar * Do, H.-Q., Chandrashekar, E. R. R. & Fu, G. C. Nickel/bis(oxazoline)-catalyzed asymmetric Negishi arylations of racemic
secondary benzylic electrophiles to generate enantioenriched 1,1-diarylalkanes. _J. Am. Chem. Soc._ 135, 16288–16291 (2013). Article CAS PubMed Google Scholar * Chen, H.-W. et al.
Asymmetric deoxygenative cyanation of benzyl alcohols enabled by synergistic photoredox and copper catalysis. _Chin. J. Chem._ 38, 1671–1675 (2020). Article ADS CAS Google Scholar * Luo,
Y.-R. _Handbook of Bond Dissociation Energies in Organic Compounds_ 1st edn, Vol. 392, 205–208 (CRC Press: Boca Raton, FL, 2002). * Anka-Lufford, L. L., Prinsell, M. R. & Weix, D. J.
Selective cross-coupling of organic halides with allylic acetates. _J. Org. Chem._ 77, 9989–10000 (2012). Article CAS PubMed PubMed Central Google Scholar * Cui, X. et al.
Nickel-catalyzed reductive allylation of aryl bromides with allylic acetate. _Org. Biomol. Chem._ 11, 3094–3097 (2013). Article CAS PubMed Google Scholar * Molander, G. A., Traister, K.
M. & O’Neill, B. T. Engaging nonaromatic, heterocyclic tosylates in reductive cross-coupling with aryl and heteroaryl bromides. _J. Org. Chem._ 80, 2907–2911 (2015). Article CAS PubMed
Google Scholar * Wang, J., Zhao, J. & Gong, H. Nickel-catalyzed methylation of aryl halides/tosylates with methyl tosylate. _Chem. Commun._ 53, 10180–10183 (2017). Article CAS
Google Scholar * Komeyama, K., Ohata, R., Kiguchi, S. & Osaka, I. Highly nucleophilic vitamin B12-assisted nickel-catalysed reductive coupling of aryl halides and non-activated alkyl
tosylates. _Chem. Commun._ 53, 6401–6404 (2017). Article CAS Google Scholar * Vara, B. A., Patel, N. R. & Molander, G. A. _O_-benzyl xanthate esters under Ni/photoredox dual
catalysis: selective radical generation and Csp3−Csp2 cross-coupling. _ACS Catal._ 7, 3955–3959 (2017). Article CAS PubMed PubMed Central Google Scholar * Ackerman, L. K. G.,
Anka-Lufford, L. L., Naodovic, M. & Weix, D. J. Cobalt co-catalysis for cross-electrophile coupling: diarylmethanes from benzyl mesylates and aryl halides. _Chem. Sci._ 6, 1115–1119
(2015). Article CAS PubMed Google Scholar * Konev, M. O., Hanna, L. E. & Jarvo, E. R. Intra- and intermolecular nickel-catalyzed reductive cross-electrophile coupling reactions of
benzylic esters with aryl halides. _Angew. Chem. Int. Ed._ 55, 6730–6733 (2016). Article CAS Google Scholar * Tao, X., Chen, Y., Guo, J., Wang, X. & Gong, H. Preparation of α-amino
acids via Ni-catalyzed reductive vinylation and arylation of α-pivaloyloxy glycine. _Chem. Sci._ 12, 220–226 (2021). Article CAS Google Scholar * Zhang, X. & MacMillan, D. W. C.
Alcohols as latent coupling fragments for metallaphotoredox catalysis: sp3−sp2 cross-coupling of cxalates with aryl halides. _J. Am. Chem. Soc._ 138, 13862–13865 (2016). Article CAS PubMed
PubMed Central Google Scholar * Yan, X.-B., Li, C.-L., Jin, W.-J., Guo, P. & Shu, X.-Z. Reductive coupling of benzyl oxalates with highly functionalized alkyl bromides by nickel
catalysis. _Chem. Sci._ 9, 4529–4534 (2018). Article CAS PubMed PubMed Central Google Scholar * Gao, M., Sun, D. & Gong, H. Ni-catalyzed reductive C−O bond arylation of oxalates
derived from α-hydroxy esters with aryl halides. _Org. Lett._ 21, 1645–1648 (2019). Article CAS PubMed Google Scholar * Arendt, K. M. & Doyle, A. G. Dialkyl ether formation by
nickel-catalyzed cross-coupling of acetals and aryl iodides. _Angew. Chem. Int. Ed._ 54, 9876–9880 (2015). Article CAS Google Scholar * Pan, Y., Gong, Y., Song, Y., Tong, W. & Gong,
H. Deoxygenative cross-electrophile coupling of benzyl chloroformates with aryl iodides. _Org. Biomol. Chem._ 17, 4230–4233 (2019). Article CAS PubMed Google Scholar * Kariofillis, S.
K., Shields, B. J., Tekle-Smith, M. A., Zacuto, M. J. & Doyle, A. G. Nickel/photoredox-catalyzed methylation of (hetero)aryl chlorides using trimethyl orthoformate as a methyl radical
source. _J. Am. Chem. Soc._ 142, 7683–7689 (2020). Article CAS PubMed PubMed Central Google Scholar * Parasram, M., Shields, B. J., Ahmad, O., Knauber, T. & Doyle, A. G.
Regioselective cross-electrophile coupling of epoxides and (hetero)aryl iodides via Ni/Ti/photoredox catalysis. _ACS Catal._ 10, 5821–5827 (2020). Article CAS PubMed PubMed Central
Google Scholar * Kariofillis, S. K. et al. Using data science to guide aryl bromide substrate scope analysis in a Ni/photoredox-catalyzed cross-coupling with acetals as alcohol-derived
radical sources. _J. Am. Chem. Soc._ 144, 1045–1055 (2022). Article CAS PubMed PubMed Central Google Scholar * Suga, T. & Ukaji, Y. Nickel-catalyzed cross-electrophile coupling
between benzyl alcohols and aryl halides assisted by titanium co-reductant. _Org. Lett._ 20, 7846–7850 (2018). Article CAS PubMed Google Scholar * Suga, T., Takahashi, Y. & Ukaji, Y.
One-shot radical cross coupling between benzyl alcohols and alkenyl halides using Ni/Ti/Mn system. _Adv. Synth. Catal._ 362, 5622–5626 (2020). Article CAS Google Scholar * Diéguez, H. R.
et al. Weakening C-O bonds: Ti(III), a new reagent for alcohol deoxygenation and carbonyl coupling olefination. _J. Am. Chem. Soc._ 132, 254–259 (2010). Article PubMed Google Scholar *
Zheng, X. et al. Umpolung of hemiaminals: titanocene-catalyzed dehydroxylative radical coupling reactions with activated alkenes. _Angew. Chem. Int. Ed._ 52, 3494–3498 (2013). Article ADS
CAS Google Scholar * Suga, T., Shimazu, S. & Ukaji, Y. Low-valent titanium-mediated radical conjugate addition using benzyl alcohols as benzyl radical sources. _Org. Lett._ 20,
5389–5392 (2018). Article CAS PubMed Google Scholar * Xie, H. et al. Radical dehydroxylative alkylation of tertiary alcohols by Ti catalysis. _J. Am. Chem. Soc._ 142, 16787–16794 (2020).
Article CAS PubMed Google Scholar * Sumiyama, K. et al. Use of isopropyl alcohol as a reductant for catalytic dehydoxylative dimerization of benzylic alcohols utilizing Ti–O bond
photohomolysis. _Eur. J. Org. Chem_. 2021, 2474–2478 (2021). * Xie, H., Wang, S., Wang, Y., Guo, P. & Shu, X.-Z. Ti-catalyzed reductive dehydroxylative vinylation of tertiary alcohols.
_ACS Catal._ 12, 1018–1023 (2022). Article CAS Google Scholar * Suga, T., Takahashi, Y., Miki, C. & Ukaji, Y. Direct and unified access to carbon radicals from aliphatic alcohols by
cost-efficient titanium-mediated homolytic C–OH bond cleavage. _Angew. Chem. Int. Ed._ 61, e202112533 (2022). Article CAS Google Scholar * Suga, T., Takada, R., Shimazu, S., Sakata, M.
& Ukaji, Y. Highly (_E_)-selective trisubstituted alkene synthesis by low-valent titanium-mediated homolytic cleavage of alcohol C–O bond. _J. Org. Chem._ 87, 7487–7493 (2022). Article
CAS PubMed Google Scholar * Lin, Q., Tong, W., Shu, X.-Z. & Chen, Y. Ti-catalyzed dehydroxylation of tertiary alcohols. _Org. Lett._ 24, 8459–8464 (2022). Article CAS PubMed Google
Scholar * Li, Z. et al. Electrochemically enabled, nickel-catalyzed dehydroxylative cross-coupling of alcohols with aryl halides. _J. Am. Chem. Soc._ 143, 3536–3543 (2021). Article CAS
PubMed Google Scholar * Li, Y. et al. Chemoselective and diastereoselective synthesis of _C_-aryl nucleoside analogues by nickel-catalyzed cross-coupling of furanosyl acetates with aryl
iodides. _Angew. Chem. Int. Ed._ 61, e202110391 (2022). Article CAS Google Scholar * Lin, Q., Ma, G. & Gong, H. Ni-catalyzed formal cross-electrophile coupling of alcohols with aryl
halides. _ACS Catal._ 11, 14102–14109 (2021). Article CAS Google Scholar * Chi, B. K. et al. In-situ bromination enables formal cross-electrophile coupling of alcohols with aryl and
alkenyl halides. _ACS Catal._ 12, 580–586 (2022). Article CAS PubMed Google Scholar * Jia, X.-G., Guo, P., Duan, J. & Shu, X.-Z. Dual nickel and lewis acid catalysis for
cross-electrophile coupling: the allylation of aryl halides with allylic alcohols. _Chem. Sci._ 9, 640–645 (2018). Article CAS PubMed Google Scholar * Guo, P. et al. Dynamic kinetic
cross-electrophile arylation of benzyl alcohols by nickel catalysis. _J. Am. Chem. Soc._ 143, 513–523 (2021). Article CAS PubMed Google Scholar * Ma, W.-Y. et al. Cobalt-catalyzed
enantiospecific dynamic kinetic cross-electrophile vinylation of allylic alcohols with vinyl triflates. _J. Am. Chem. Soc._ 143, 15930–15935 (2021). Article CAS PubMed Google Scholar *
Pan, F.-F., Guo, P., Huang, X. & Shu, X.-Z. Synthesis of dibenzyls by nickel-catalyzed homocoupling of benzyl alcohols. _Synthesis_ 53, 3094–3100 (2021). Article CAS Google Scholar *
Chen, Y.-G. et al. Regioselective Ni-catalyzed carboxylation of allylic and propargylic alcohols with carbon dioxide. _Org. Lett._ 19, 2969–2972 (2017). Article CAS PubMed Google Scholar
* van Gemmeren, M. et al. Switchable site-selective catalytic carboxylation of allylic alcohols with CO2. _Angew. Chem. Int. Ed._ 56, 6558–6562 (2017). Article Google Scholar *
Chenniappan, V. K., Peck, D. & Rahaim, R. Nickel catalyzed deoxygenative cross-coupling of benzyl alcohols with aryl-bromides. _Tetrahedron Lett._ 61, 151729 (2020). Article Google
Scholar * Tercenio, Q. D. & Alexanian, E. J. Stereospecific nickel-catalyzed reductive cross-coupling of alkyl tosylate and allyl alcohol alectrophiles. _Org. Lett._ 23, 7215–7219
(2021). Article CAS PubMed PubMed Central Google Scholar * Wang, H. et al. Nickel-catalyzed reductive Csp2−Csp3 cross coupling using phosphonium salts. _Org. Lett._ 23, 8183–8188
(2021). Article CAS PubMed Google Scholar * Yu, H. & Wang, Z.-X. Nickel-catalyzed cross-electrophile coupling of aryl chlorides with allylic alcohols. _Org. Biomol. Chem._ 19,
9723–9731 (2021). Article CAS PubMed Google Scholar * Fan, Z., Chen, S., Zou, S. & Xi, C. Direct C–C bond formation of allylic alcohols with CO2 toward carboxylic acids by
ahotoredox/nickel dual catalysis. _ACS Catal._ 12, 2781–2787 (2022). Article CAS Google Scholar * Dong, Z. & MacMillan, D. W. C. Metallaphotoredox-enabled deoxygenative arylation of
alcohols. _Nature_ 598, 451–456 (2021). Article ADS CAS PubMed PubMed Central Google Scholar * Sakai, H. A. & MacMillan, D. W. C. Nontraditional fragment couplings of alcohols and
carboxylic acids: C(_sp__3_)−C(_sp__3_) cross-coupling via radical sorting. _J. Am. Chem. Soc._ 144, 6185–6192 (2022). Article CAS PubMed PubMed Central Google Scholar * Intermaggio, N.
E., Millet, A., Davis, D. L. & MacMillan, D. W. C. Deoxytrifluoromethylation of alcohols. _J. Am. Chem. Soc._ 144, 11961–11968 (2022). Article CAS PubMed PubMed Central Google
Scholar * Wang, J. Z., Sakai, H. A. & MacMillan, D. W. C. Alcohols as alkylating agents: photoredox-catalyzed conjugate alkylation via in situ deoxygenation. _Angew. Chem. Int. Ed._ 61,
e202207150 (2022). Article CAS Google Scholar * Lyon, W. L. & MacMillan, D. W. C. Expedient access to underexplored chemical space: deoxygenative C(sp3)−C(sp3) cross-coupling. _J.
Am. Chem. Soc._ 145, 7736–7742 (2023). Article CAS PubMed PubMed Central Google Scholar * Gould, C. A., Pace, A. L. & MacMillan, D. W. C. Rapid and modular access to quaternary
carbons from tertiary alcohols via bimolecular homolytic substitution. _J. Am. Chem. Soc._ 145, 16330–16336 (2023). Article CAS PubMed PubMed Central Google Scholar * Zhao, W.-T. &
Shu, W. Enantioselective Csp3-Csp3 formation by nickel-catalyzed enantioconvergent cross-electrophile alkyl-alkyl coupling of unactivated alkyl halides. _Sci. Adv._ 9, eadg9898 (2023).
Article CAS PubMed PubMed Central Google Scholar * Schuster, D. et al. Pharmacophore-based discovery of FXR agonists. Part I: model development and experimental validation. _Bioorg.
Med. Chem._ 19, 7168–7180 (2011). Article CAS PubMed PubMed Central Google Scholar * Huang, T. et al. Phytochemical and biological studies on rare and endangered plants endemic to
China. Part XV. structurally diverse diterpenoids and sesquiterpenoids from the vulnerable conifer _Pseudotsuga sinensis_. _Phytochemistry_ 169, 112184 (2020). Article CAS PubMed Google
Scholar * Werner, E. W., Mei, T.-S., Burckle, A. J. & Sigman, M. S. Enantioselective heck arylations of acyclic alkenyl alcohols using a redox-relay strategy. _Science_ 338, 1455–1458
(2012). Article ADS CAS PubMed PubMed Central Google Scholar * Khatua, A., Pal, S., Das, M. K. & Bisai, V. Asymmetric total syntheses of (–)-_ar_-turmerone,
(–)-dihydro-_ar_-turmerone, (–)-_ar_-dehydrocurcumene, and (–)-_ar_-himachalene via a key allylic oxidative rearrangement. _Tetrahedron Lett._ 73, 153105 (2021). Article CAS Google Scholar
* Zhang, L. et al. Nickel-catalyzed enantioselective reductive conjugate arylation and heteroarylation via an elementary mechanism of 1,4-addition. _J. Am. Chem. Soc._ 144, 20249–20257
(2022). Article CAS PubMed Google Scholar * Beckwith, A. L. J. & Schiesser, C. H. Regio- and stereo-selectivity of alkenyl radical ring closure: a theoretical study. _Tetrahedron_
41, 3925–3941 (1985). Article CAS Google Scholar * Anslyn, E. V. & Dougherty, D. A. _Modern Physical Organic Chemistry_. 155–157 (University Science Books, 2006). Download references
ACKNOWLEDGEMENTS We thank Prof. Gregory C. Fu (Caltech), Prof. Shu-Li You (SIOC), and Prof. Chao Zheng (SIOC) for helpful suggestions and proofreading. Support has been provided by the
National Natural Science Foundation of China (Grant No. 22201216), the National Key Research & Development Program of China (Grant No. 2023YFA1508600), and the Fundamental Research Funds
for the Central Universities (Grant No. 22120230252, 2023-3-YB-10). AUTHOR INFORMATION Author notes * These authors contributed equally: Li-Li Zhang, Yu-Zhong Gao. AUTHORS AND AFFILIATIONS
* School of Chemical Science and Engineering, Tongji University, Shanghai, 200092, People’s Republic of China Li-Li Zhang, Sheng-Han Cai, Hui Yu & Ze-Peng Yang * Key Laboratory of
Magnetic Molecules, Magnetic Information Materials Ministry of Education, The School of Chemical and Material Science, Shanxi Normal University, Taiyuan, 030031, People’s Republic of China
Yu-Zhong Gao & Shou-Jie Shen * State Key Laboratory of Pollution Control and Resource Reuse, College of Environmental Science and Engineering, Tongji University, Shanghai, 200092,
People’s Republic of China Qian Ping Authors * Li-Li Zhang View author publications You can also search for this author inPubMed Google Scholar * Yu-Zhong Gao View author publications You
can also search for this author inPubMed Google Scholar * Sheng-Han Cai View author publications You can also search for this author inPubMed Google Scholar * Hui Yu View author publications
You can also search for this author inPubMed Google Scholar * Shou-Jie Shen View author publications You can also search for this author inPubMed Google Scholar * Qian Ping View author
publications You can also search for this author inPubMed Google Scholar * Ze-Peng Yang View author publications You can also search for this author inPubMed Google Scholar CONTRIBUTIONS
Z.-P.Y. conceived and directed the project. L.-L.Z. and Y.-Z.G. discovered and developed the reaction. L.-L.Z., Y.-Z.G. and S.-H.C. performed the experiments and collected the data. H.Y.,
S.-J.S. and Q.P. discussed the project with Z.-P.Y. Z.-P.Y. wrote the paper with contributions from all authors. L.-L.Z. and Y.-Z.G. contributed equally. CORRESPONDING AUTHOR Correspondence
to Ze-Peng Yang. ETHICS DECLARATIONS COMPETING INTERESTS The authors declare no competing interests. PEER REVIEW PEER REVIEW INFORMATION _Nature Communications_ thanks Takuya Suga and the
other anonymous reviewer(s) for their contribution to the peer review of this work. A peer review file is available. ADDITIONAL INFORMATION PUBLISHER’S NOTE Springer Nature remains neutral
with regard to jurisdictional claims in published maps and institutional affiliations. SUPPLEMENTARY INFORMATION SUPPLEMENTARY INFORMATION PEER REVIEW FILE RIGHTS AND PERMISSIONS OPEN ACCESS
This article is licensed under a Creative Commons Attribution 4.0 International License, which permits use, sharing, adaptation, distribution and reproduction in any medium or format, as
long as you give appropriate credit to the original author(s) and the source, provide a link to the Creative Commons licence, and indicate if changes were made. The images or other third
party material in this article are included in the article’s Creative Commons licence, unless indicated otherwise in a credit line to the material. If material is not included in the
article’s Creative Commons licence and your intended use is not permitted by statutory regulation or exceeds the permitted use, you will need to obtain permission directly from the copyright
holder. To view a copy of this licence, visit http://creativecommons.org/licenses/by/4.0/. Reprints and permissions ABOUT THIS ARTICLE CITE THIS ARTICLE Zhang, LL., Gao, YZ., Cai, SH. _et
al._ Ni-catalyzed enantioconvergent deoxygenative reductive cross-coupling of unactivated alkyl alcohols and aryl bromides. _Nat Commun_ 15, 2733 (2024).
https://doi.org/10.1038/s41467-024-46713-x Download citation * Received: 14 September 2023 * Accepted: 07 March 2024 * Published: 28 March 2024 * DOI:
https://doi.org/10.1038/s41467-024-46713-x SHARE THIS ARTICLE Anyone you share the following link with will be able to read this content: Get shareable link Sorry, a shareable link is not
currently available for this article. Copy to clipboard Provided by the Springer Nature SharedIt content-sharing initiative