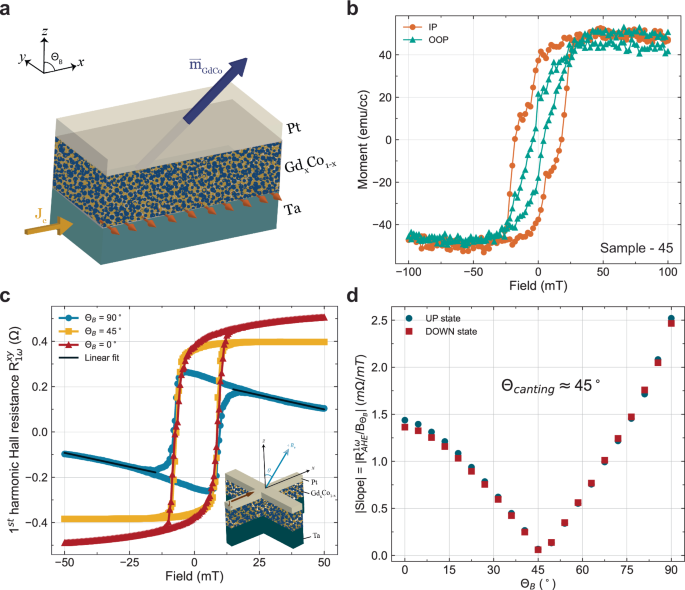
Tunable multistate field-free switching and ratchet effect by spin-orbit torque in canted ferrimagnetic alloy
- Select a language for the TTS:
- UK English Female
- UK English Male
- US English Female
- US English Male
- Australian Female
- Australian Male
- Language selected: (auto detect) - EN
Play all audios:

ABSTRACT Spin-orbit torque is not only a useful probe to study manipulation of magnetic textures and magnetic states at the nanoscale but also it carries great potential for next-generation
computing applications. Here we report the observation of rich spin-orbit torque switching phenomena such as field-free switching, multistate switching, memristor behavior and ratchet effect
in a single shot, co-sputtered, rare earth-transition metal Gd_x_Co100−_x_. Notably such effects have only been observed in antiferromagnet/ferromagnet bi-layer systems previously. We show
that these effects can be traced to a large anistropic canting, that can be engineered into the Gd_x_Co100−_x_ system. Further, we show that the magnitude of these switching phenomena can be
tuned by the canting angle and the in-plane external field. The complex spin-orbit torque switching observed in canted Gd_x_Co100−_x_ not only provides a platform for spintronics but also
serves as a model system to study the underlying physics of complex magnetic textures and interactions. SIMILAR CONTENT BEING VIEWED BY OTHERS ASYNCHRONOUS CURRENT-INDUCED SWITCHING OF
RARE-EARTH AND TRANSITION-METAL SUBLATTICES IN FERRIMAGNETIC ALLOYS Article 12 May 2022 FIELD-FREE SPIN-ORBIT TORQUE SWITCHING ASSISTED BY IN-PLANE UNCONVENTIONAL SPIN TORQUE IN ULTRATHIN
[PT/CO]N Article Open access 04 July 2023 AN ENERGY EFFICIENT WAY FOR QUANTITATIVE MAGNETIZATION SWITCHING Article Open access 17 July 2024 INTRODUCTION Spin-orbit torque (SOT) driven
magnetization switching has great potential for next-generation magnetic memory technology1,2. At the same time, it serves as a powerful probe to study spin-dependent phenomena such as
topological spin textures and quantum materials3,4. However, despite substantial effort over the last decade, challenges remain in terms of further lowering the critical switching current
density, exploring complimentary metal oxide semiconductor (CMOS) compatible materials with high spin-torque efficiency, engineering field-free switching designs2, etc. As a result, the
search for new materials and new spin/magnetic phenomena remains an ongoing effort5,6,7,8,9,10,11. In this regard, ferrimagnets have been an emerging material system that possesses abundant
magnetic properties for interesting phenomena and potential spintronics applications12. Ferrimagnets are composed of two different magnetic sublattices that are antiferromagnetically
coupled, which yields a non-zero magnetization13. As a result, ferrimagnets combine the unique properties of both ferromagnets (FM) and antiferromagnets (AFM), enabling magnetic properties
and phenomena such as bulk perpendicular magnetic anisotropy (PMA)14, bulk Dzyaloshinskii-Moriya Interaction (DMI)15, magnetic skyrmion host16, spin-orbit torque switching17, and all-optical
switching18. While there exist many different families of ferrimagnets, rare earth (RE) - transition metal (TM) ferrimagnetic alloy stands out for spintronics applications12,14,17,18 due to
its highly tunable magnetic, electronic, thermomagnetic, optical properties and relatively simple thin film deposition method12,14,19,20. Among many fascinating properties of RE-TM
ferrimagnetic alloys, the bulk perpendicular magnetic anisotropy (PMA), which even persists in thick films in the micro-meter regime14,20 is the driving force behind all the fascinating
magnetic phenomena. However, switching a PMA magnet with an in-plane polarized spin current requires an in-plane symmetry-breaking field to achieve deterministic switching21,22,23. Despite
many efforts in studying RE-TM ferrimagnetic alloys for spintronics applications12,14,15,16,17,18,19,20,24,25, it is not until recently that field-free spin-orbit torque switching can be
achieved in RE-TM ferrimagnetic systems26,27,28,29. It is not surprising that RE-TM ferrimagnets carry the capability of inducing a tilt in its anisotropy since it possess properties of both
FM and AFM. In fact, one of the most common systems designed to induce a tilt in its PMA for field-free SOT switching is the AFM/FM bi-layer, where an exchange bias is present23,30. In
AFM/FM bi-layer systems, not only field-free switching23,30,31 can be realized but also many interesting magnetic textures and switching behaviors such as electrical control of
antiferromagnetic order32,33, multistate switching23,34, and memristor behavior23,34 can be observed owing to its dynamic and intricate magnetic interaction at the AFM/FM interface35. Due to
the exchange coupling at the AFM/FM interface, AFM spin configuration at the interface can be controlled via SOT and exhibits an exchange spring effect that manifests in a ratchet behavior
of the magnetic states35 and antiferromagnetic states36,37. Since ferrimagnetism combines both FM and AFM properties, it is possible to observe not only tilting in its magnetic anisotropy
but also possibly all the switching behaviors found in AFM/FM systems, such as field-free switching, multistate switching, memristor behavior, exchange spring effect, and ratchet effect. In
RE-TM ferrimagnetic alloys, the parameter space for material design is also much wider with knobs on atomic concentration between RE and TM, thickness scaling (not limited to ultrathin ~ 1
nm thickness due to interfacial PMA), different RE and TM elements, growth condition, and operating temperature. With this motivation, we explore the possibility of achieving the interesting
switching behaviors and phenomena observed in AFM/FM systems in a single-layer ferrimagnetic GdCo alloy without any superlattice design that is deposited in a single shot. In this paper, we
report field-free spin-orbit torque switching of GdCo near its magnetic compensation with canted anisotropy and exchange spring behavior at room temperature via transport and magnetometry
measurements. Through angle-dependent anomalous Hall resistance field loop measurement, the angle of the canting can be characterized. In addition, multistate switching, memristor behavior,
and ratchet effect are observed where the multistate and ratchet effect is tunable with the in-plane symmetry-breaking field. This demonstrates that single-shot RE-TM ferrimagnetic alloys
possess similar properties to AFM/FM bi-layers, which expands the material design space for spintronics applications. RESULTS THIN FILM DEPOSITION AND CHARACTERIZATION OF MAGNETIC ANISOTROPY
IN GDCO Canted Gd_x_Co100−_x_ are deposited via co-sputtering of elemental Gd and Co targets with base pressure around 1e-8 Torr prior to deposition. The canted Gd_x_Co100−_x_ thin films
are deposited on the thermally oxidized silicon substrate with 8 nm Ta as the underlayer and capped with 2 nm Pt to prevent oxidation (see Fig. 1a). In this study, three samples (sample-45,
sample-56, and sample-13) with different anisotropy canting angles are deposited with the nominal Gd concentrations to be around 24 ~ 25% (see “Methods” Table 1), which is close to the
magnetic compensation point19,20,38. The thin film magnetometry conducted under room temperature on sample-45 reveals an exchange spring behavior and a large canting of magnetic anisotropy
(see Fig. 1b). The magnetization hysteresis loops taken along the OOP and IP directions show comparable remanent magnetization in both directions, indicating a large canting and the total
effective anisotropy is close to none. Not only the remanent magnetization is comparable, but also a two-phase switching characterized by a small coercive field and a large coercive field
can be observed in both IP and OOP directions. This two-phase switching indicates the Gd_x_Co100−_x_ layer is most likely composed of a soft magnetic layer and a hard magnetic layer, which
is a signature of exchange-spring behavior39. This phenomenon has also been observed in other RE-TM ferrimagnetic systems such as TbCo, especially when the thickness is ultrathin (< 10
nm)39. In TbCo, the first 2 nm layer is composed of a soft low-density magnetic layer. The sequential layers deposited are much denser and have harder magnetic layers. The coexistence of the
soft and hard magnetic layers in RE-TM ferrimagnetic alloy that are exchange coupled serve as a great exchange spring system to engineer a tunable anisotropy tilting through controlling the
concentration, growth condition, underlayer, and overlayer39,40. Here, in Gd_x_Co100−_x_, this effect can be amplified due to the symmetric filling of the 4_f_ shell in Gd compared to Tb
(details see Supplementary Note 1). In order to quantify the anisotropy canting angle of each sample, we present a new way of characterizing anisotropy canting angle through anomalous Hall
effect (AHE) measurements with the field direction to be a function of the angle between the _z_-axis and _x_-axis (see Fig. 1c inset). Most often, for thin-film magnets, the magnetic
easy-axis is either strictly in-plane (_θ_B = 90∘) due to the shape anisotropy or strictly out-of-plane due to strong interfacial or bulk PMA (_θ__B_ = 0∘). In our case, due to the large
canting of the magnetic anisotropy, the easy axis will be between 0∘ and 90∘. In a typical AHE measurement, when the field is applied in the magnetic easy-axis, the AHE hysteresis will
behave as constant resistance values above the two coercive fields (B_c_), and the switching between the two constant resistance values occurs upon crossing the coercive fields. In the case
of the field being applied in the magnetic hard axis, the AHE hysteresis curve is a sloped line with minimal hysteresis opening below the anisotropy field before the moments are saturated.
This is because there are magnetizations in which the easy-axis does not align with the field direction thus, as the field increases, these magnetizations are pulled into the field direction
and away from their magnetic easy-axis. However, when the external field direction is aligned with the magnetization easy-axis, the slope in the higher field region (above ∣B_c_∣) should be
close to zero since all moments are saturated. As a result, when the AHE measurements are done with the field applied in a direction between the easy axis and the hard axis, the
superposition of the AHE hysteresis from both cases (easy axis and hard axis) occurs, and the AHE resistance should be a sloped line in the region above the coercive fields. It is to be
noted that the canting angle obtained through this method represents the canting of the magnetic easy-axis in the net magnetization of the fabricated GdCo heterostructure device. This
canting angle is different from the tilting angle of the OOP magnetization often seen in field-free switching literature26,27 because there is a very minimal IP magnetization component in
these systems. We patterned the thin films into Hall bar devices (“methods”) and carried out the angle-dependent AHE measurement on sample-45. In Fig. 1c and Supplementary Fig. S1a, a series
of AHE hysteresis loops measured at different field directions _θ__B_ are shown. In the region above the coercive fields, the slopes of the curve decreases in magnitude as the field
direction approaches 45 degrees, and once it crosses 45 degrees, the slope magnitudes begin to increase again (see Fig. 1d). By fitting a line to the region above the coercive fields (see
Fig. 1c), we are able to find the angle at which the minimum slope is obtained. In sample-45, the minimum slope is found to be 45 degrees away from the z-axis (see Fig. 1d). This confirms
that the magnetic easy-axis in sample-45 is indeed largely canted from the typical IP or OOP directions. RECIPE FOR INDUCING LARGE CANTING IN GDCO FERRIMAGNET The canting in GdCo can be
induced through oxygen incorporation inside the GdCo layer. To study the effect of oxygen to induce canting, we fabricated Hall bar devices on Ta (8 nm) / Gd_x_Co100−_x_ (10 nm) / Pt (2.5
nm) thin film heterostructure that is deposited with composition near the magnetic compensation at room temperature (Fig. 2a). Without any oxidation, the device shows a strong in-plane
anisotropy characterized by the sloped straight line in the AHE field loop (Fig. 2b). As we treat the device to a mild oxygen plasma (50 W, 180 mTorr, 50 ∘C) with a set duration, the shape
of the AHE loop transformed from a straight line into a switching loop with improved squareness. After a set amount of oxidation, the squareness no longer improves and rather may degrade if
further oxidation is carried out. At this point, we can let the sample stay in an ambient environment for at least a day, the squareness then further improves (Fig. 2b). By conducting the
angle-dependent AHE field sweep measurement, we can characterize the canting angle of the easy-axis after 1 day and 58 days which turns out to be 22.5 and 13.5 degrees from OOP axis (Fig.
2c, d). We believe the step for the sample to stay in an ambient environment is for oxygen to slowly diffuse into the bulk of the GdCo layer and create an OOP anisotropy (Supplementary Note
2). By controlling the duration of the oxygen plasma step and the duration of the ambient exposure (or acceleration through elevated temperature annealing), a canting angle anywhere between
IP and OOP should be obtainable with reasonable optimization. FIELD-FREE SPIN-ORBIT TORQUE SWITCHING IN CANTED GDCO HETEROSTRUCTURES With the magnetic anisotropy canting angle quantified, we
first performed the spin-orbit torque switching experiments to realize the possibility of SOT switching in the absence of a symmetry-breaking field on the canted GdCo samples. We performed
pulsed current measurements (200 μs) on Hall bar devices (see Fig. 3a) with anomalous Hall effect as the voltage readout mechanism to probe the magnetic state of the canted GdCo moments
(“Methods”). Before we applied the current pulses, a large set field in the z-direction (B_se__t_,_z_ = ± 100 mT) was applied to initialize the magnetic moments to a known state. After the
moments are initialized, the large set field is removed. The current pulse amplitude sequence for studying the SOT switching behavior in Fig. 3 starts from a large negative current value (−
27 mA) above the critical switching threshold and traverses to the positive critical switching current value (+ 27 mA) and back to the negative critical switching current value with a
hysteresis profile. SOT switching curves from sample-45 without symmetry-breaking fields are shown in Fig. 3b. Switching between two magnetic states is observable, which confirms the
field-free switching behavior in largely canted GdCo moments. In addition to the field-free switching, the _Δ__R__A__H__E_ appears to be dependent on the initialization direction with the
case of B_set,z_ = 100 mT having a larger _Δ__R__A__H__E,_ and the switching chirality is independent of the initialization direction. This indicates that the symmetry breaking caused by the
canting is fixed in a particular direction, but the strength of the canting is dependent on the initialization direction. The difference in _Δ__R__A__H__E_ is amplified under a certain
combination of B_s__e__t_,_z,_ and B_x_. In Fig. 3c and d, the switching curves were obtained with ± 2.5 mT symmetry-breaking fields during the switching experiments, and the magnet was
initialized with B_se__t_,_z_ = ± 100 mT. From these four switching curves, we can confirm that the effective canting field in Gd_x_Co100−_x_ is in the direction of \(-\hat{x}\) since the
switching chirality is the same between the case of B_x_ = 0 and the case of B_x_ = − 2.5 mT. By comparing the four curves, the switching chirality is consistent with the symmetry-breaking
field sign. However, for the case of [B_z_,_s__e__t_, B_x_] = [100 mT, 2.5 mT] (see Fig. 3c), the _Δ__R__A__H__E_ is highly suppressed compared to the rest of the three switching curves.
While it is expected that a positive B_x_ may partially cancel out the canting since the effective canting field is in the \(-\hat{x}\) direction, it is not immediately clear on the
B_z_,_s__e__t_ dependence of _Δ__R__A__H__E_ suppression. In order to better understand how the symmetry breaking field (B_x_) and the initialization conditions affect the canting of the
anisotropy during SOT switching, we repeated the switching experiment over a series of B_x_ for both initialization cases B_se__t_,_z_ = ± 100 mT (see Fig. 4). In Fig. 4, all _R__A__H__E_
loops are plotted as they are without any centering or normalization since the DC offsets of the _R__A__H__E_ are dependent on B_x_. For moments initialized by 100 mT, clear switching due to
SOT can be clearly seen in all symmetry-breaking fields (see Fig. 4a). However, reduced _Δ__R__A__H__E_ are present for B_x_ = 1 mT and 2.5 mT (See Figs. 4a and 3c), indicating partial
switching. This can be due to the canting effective field being canceled by the externally applied in-plane field (B_x_). The effective canting field from Fig. 4a is between 1 and 2.5 mT in
the direction of \(-\hat{x}\). This is further supported by the fact that the switching chirality changed between 1 mT and 2.5 mT. In a usual SOT switching framework without any canting in
the anisotropy21,22, switching chirality reverses when the sign of the symmetry-breaking field reverses. However, in Fig. 4a, the switching chirality did not reverse upon crossing the zero
symmetry breaking field, instead, the chirality reversed between 1 and 2.5 mT. As for the moments initialized by − 100 mT (see Figs. 4b and 3d), similar switching behavior is observed in the
case of B_se__t_,_z_ = 100 mT. However, the field where the switching chirality reverses is reduced to be between 0 and 1 mT, with the _Δ__R__A__H__E_ to be heavily diminished at B_x_ = 1
mT. From the B_x_ dependent switching experiment (see Fig. 4), we found the effective symmetry-breaking field due to canting is between 0 and 2.5 mT in the \(-\hat{x}\) direction, and it is
also dependent on the initialization direction (\(\pm \hat{z}\)) with B_se__t_,_z_ = 100 mT yielding a larger canting effective field. This can be self-consistently observed in the amplitude
of the _Δ__R__A__H__E_ at zero symmetry breaking field. Since the canting is weaker when the magnetic state is set with a − B_z_ compared to + B_z_, this means it is more difficult to
achieve deterministic switching in the cases of − B_z_. As a result, the _Δ__R__A__H__E_ at B_x_ = 0 will be smaller for the case of B_se__t_,_z_ = −100 mT. This also explains the
diminished _Δ__R__A__H__E_ in Fig. 3c for the case of [B_x_ = 2.5 mT, B_set_,_z_ = 100 mT]. Another observation from this experiment is the flatness of the up and down magnetic states in the
switching curves as a function of the symmetry-breaking field. Regardless of the initialization field direction, the magnetic state that is set by a positive current in both switching
chirality is always sloped in the current sweep direction that goes from positive to negative. This is visible in the upstate for B_x_ ≤ − 2.5 mT and the downstate for B_x_ ≥ 2.5 mT in
Figs. 4a, 3c. This has been observed in the AFM / FM bilayer system with an in-plane exchange bias field acting on the FM with PMA in past studies23. The fact that this behavior only shows
up in one magnetic state rather than both can be due to the exchange spring effect and the ratchet effect in SOT, which is an asymmetry in the magnetic state stability35. In addition to the
observations in Fig. 4, we repeated the B_x_ dependent switching experiment with 27 mA instead of − 27 mA in both initialization cases ± 100 mT (Supplementary Fig. S2) and found even more
distinct features. By starting the current pulse sequence from 27 mA, we found that for B_set_,_z_ = 100 mT case, a gap in _R__A__H__E_ exists when the SOT tries to switch the canted moments
back to the initial state for B_x_ ≥ 5 mT. Similar behavior can be observed in the case of B_se__t_,_z_ = − 100 mT when B_x_ ≤ − 2.5 mT while the rest of the switching curves resemble the
case in Fig. 4 correspondingly. To try to understand such a gap in _R__A__H__E_, we carried out more in-depth switching experiments. TUNABLE MULTI-STATE AND RATCHET EFFECT IN CANTED GDCO
HETEROSTRUCTURES VIA SPIN-ORBIT TORQUE The ratchet effect in SOT is manifested in the asymmetry of SOT switching via a variety of transport signatures. It is mentioned in the previous
section that only one magnetic state is sloped which is always set by the positive current in Fig. 4a, b. Another signature of the SOT ratchet effect is the sharpness of the switching
between the two magnetic states. This is usually more prominent in systems with higher saturation magnetization, such as IrMn/CoFeB systems35, nevertheless, it is still observable here in
Gd_x_Co100−_x_. In Fig. 4a and b, for switching curves with decent switching amplitude (∣B_x_∣ > 1 mT), the amount of _Δ__R__A__H__E_ per unit current density increase is larger when
switching with positive current regardless of the chirality (down to up state for − B_x_ and up to the down state for + B_x_). In canted Gd_x_Co100−_x_, the ratchet effect in SOT can be
more complex, where the exchange spring effect shows up in both in-plane and out-of-plane magnetic components. Since the initialization field direction affects the SOT switching behavior,
including the effective canting field amplitude and the difference in _Δ__R__A__H__E_ from the B_x_ dependent switching experiment, it is important to understand what the magnetic state
(_R__A__H__E_) has been set to by the initialization field (B_s__e__t_,_z_) before applying a large current pulse to switch the magnetic moments. As a result, we performed the B_x_ dependent
switching experiment with a starting current that is small, so the SOT is negligible, and the initial magnetic state can be probed. Interestingly, the large initial set field B_s__e__t_,_z_
sets the magnetic moments into a state that is not reachable by SOT (see Fig. 5), and this initial state is successfully initialized to the same state regardless of the symmetry-breaking
field applied during the switching experiment. This consistent initial state is quantitatively characterized by the constant _R__A__H__E_ value (_R__m__i__n_ for B_se__t_,_z_ = 100 mT and
_R__m__a__x_ for B_se__t_,_z_ = − 100 mT) in Fig. 5c, d. Thus, an additional state is visible, and this irreversible behavior manifests a similar SOT ratchet effect in a single-layer RE-TM
ferrimagnetic alloy as in AFM/FM systems35,37,41. The multistate and SOT ratchet effect can be observed in certain combinations of B_s__e__t_,_z_, and B_x_ in our study. In Fig. 5a, the
initial magnetic states are consistently set to the lowest _R__A__H__E_ value around − 0.25 Ω by B_se__t_,_z_ = 100 mT across all the different B_x_-dependent scans, and in Fig. 5b, the
initial magnetic states are set to the highest _R__A__H__E_ value consistently as well. Here, there are two observations we would like to point out, and they are differentiated by the
switching chirality. In the first observation, we focus on the switching curves set by B_x_ ≥ 2.5 mT with B_se__t_,_z_ = 100 mT, and B_x_ ≤ 0 mT with B_se__t_,_z_ = − 100 mT (Fig. 5a, b). In
these cases, the current pulse amplitude increases to the first critical current value where SOT is strong, and the magnetic state is switched to the opposite direction away from the
initial state. The reverse switching occurred upon reaching the second critical current with the opposite sign. However, the canted moments did not switch back to the original _R__A__H__E_
state set by the large initial field for cases of B_x_ ≥ 2.5 mT in Fig. 5a and B_x_ ≤ 0 mT in Fig. 5b. Instead, the _R__A__H__E_ state set by the SOT is near the mid-point of the full
_R__A__H__E_ curve, exhibiting a ratchet behavior. More interestingly, this ratchet effect exists for both switching chiralities. For the first observation, ratchet switching is defined in
the fashion that the first critical current switched the _R__A__H__E_ state away from the initial state to the opposite state, and when the current amplitude reaches the second critical
current with the opposite sign, the _R__A__H__E_ state that is set by the SOT does not return to the initial _R__A__H__E_ state set by the large magnetic field. For the second observation,
we focus on the switching curves of B_x_ = − 2.5 ~ 1 mT with B_se__t_,_z_ = 100 mT, and B_x_ = 1 ~ 5 mT with B_se__t_,_z_ = − 100 mT. In this case, we still observe the multistate even
though the first critical current sign already favors the initial magnetization state direction. Upon reaching the first critical current, the _R__A__H__E_ state is set to a different value
from the initial _R__A__H__E_ and once the current reaches the second critical current with the opposite sign, the _R__A__H__E_ state is switched to the opposite magnetic state. In fact, as
the symmetry-breaking field becomes stronger, the middle _R__A__H__E_ state that is set by the first critical current shifts into the _R__A__H__E_ state that is set by the second critical
current value, and the multistate is absent. This shows that the middle _R__A__H__E_ state can be tuned by the external symmetry-breaking field. If we disregard the initial magnetic state in
Fig. 5, the middle _R__A__H__E_ is essentially the bottom (top) state for the case of B_se__t_,_z_ = 100 mT (− 100 mT) in Fig. 4. To facilitate the comparison, the superposition of the
switching curves obtained with different starting current values but same symmetry breaking field and initialization field is plotted in Supplementary Fig. S3. The two states achieved by the
SOT with a large starting current is exactly overlapping on the part where the middle state and the final state are achieved by the SOT in the curve with the multistate (see Supplementary
Fig. S3). By calculating the _Δ__R__A__H__E_ for [B_x_, B_s__e__t_,_z_] = [7.5 mT, 100 mT] and [ − 7.5 mT, − 100 mT] in Fig. 5, we obtain _Δ__R__AHE_ = 1.26 and 1.25 Ω respectively (see
Fig. 5c, and d). If we further compare these values to the _Δ__R__A__H__E_ at zero field (1.064 Ω), obtained by multiplying the AHE field sweep hysteresis loop in the z-direction by
\(\sqrt{2}\) since it is detected by a lock-in amplifier (See Fig. 3b and methods), we find very similar values. This indicates that SOT either fully switched or very close to fully switched
the canted moments from its initial state set by the large B_s__e__t_,_z_ to the opposite state resulting in a _Δ__R__A__H__E_ close to the _Δ__R__A__H__E_ obtained by field sweep where the
moments are fully saturated in the _z_-direction. However, only partial switching can be obtained when the moments are switched back, resulting in the presence of a middle state in Fig. 5,
thus the ratchet effect. This phenomenon suggests the presence of fixed effective fields due to canting in both the in-plane and the out-of-plane direction. The change of the _R__A__H__E_
under different [B_x_, B_s__e__t_,_z_] conditions in Fig. 5 is the result of both the in-plane and the out-of-plane components of the canted GdCo moments are being manipulated by the SOT and
the switching dynamics of both components affecting each other, potentially due to the exchange spring effect. For completeness and repeatability, the symmetry-breaking field dependence
switching curves of conditions [I_st__a__rt_ = 1 mA, B_se__t_,_z_ = 100 mT] and [I_st__a__rt_ = 1 mA, B_se__t_,_z_ = − 100 mT] are shown in Supplementary Fig. S4 and the results are
consistent and symmetric to the cases in Fig. 5. EFFECT OF DIFFERENT CANTING ANGLES ON SPIN-ORBIT TORQUE SWITCHING IN GDCO HETEROSTRUCTURES From the SOT switching results on sample-45, it is
shown that strong magnetic anisotropy canting can lead to different switching phenomena, such as the ratchet effect, field-free switching, and multistate switching. Here we further explore
the effect of canting angle on the various switching phenomena. From our sample preparations, single-shot co-sputtering deposition of GdCo can lead to different canting angles even with the
same sputtering parameters but near the compensation point. Two other samples we found to possess different canting angles are shown in Fig. 6 (56.25∘) and Fig. 7 (13.5∘). Sample-56 has a
much larger saturation magnetization (Fig. 6a), and the canting angle (56.25∘) is closer to in-plane (Fig. 6c). For sample-56, the two-phase switching in the magnetometry data is weaker but
still observable, especially in the OOP direction (Fig. 6a). Although the anisotropy is closer to being in-plane thus the smaller squareness (_M__r_ / _M__s_), the field-sweep AHE curve
still exhibits a clear hysteresis (Fig. 6b). However, the SOT switching curve does not exhibit a strong two-state switching, instead, a very gradual switching curve as a function of current
amplitude is observed (Fig. 6d and e). This is mostly due to the weak PMA of sample-56 since the readout of the magnetic state is through the anomalous Hall effect. Interestingly, among the
two different switching conditions [B_z_,_s__et_ = − 100 mT, B_x_ = ± 2.5 mT], the _Δ__R__A__H__E_ of the case [B_x_ = + 2.5 mT] is highly suppressed but largely enhanced for the case [B_x_
= − 2.5 mT] (Fig. 6d). This is similar to sample-45 (Fig. 3c) where _Δ__R__A__H__E_ is suppressed for the case of [B_z_,_s__et_ = 100 mT, B_x_ = 2.5 mT] but the discrepancy between the
opposite sign of B_x_ is enhanced with a larger canting angle (sample-56). For the cases of [B_z_,_s__et_ = 100 mT, B_x_ = ± 2.5 mT] in sample-56, the _Δ__R__A__H__E_ is comparable
regardless of the symmetry breaking field, and also the chirality switches accordingly, which is the same as the case [B_z_,_s__et_ = − 100 mT, B_x_ = ± 2.5 mT] (Fig. 3d) in sample-45. While
it is expected that very minimal magnetic switching can be observed in sample-56 without a symmetry-breaking field due to the weak PMA, a non-zero hysteresis is still observed at zero
symmetry-breaking field (Fig. 6f). In addition, the switching chirality is fixed regardless of the initialization field (Fig. 6f), which is also similar to the zero-field switching result in
sample-45 (Fig. 3b). The opposite sign of the curvature in the two switching curves (Fig. 6f) is most likely due to the IP moments that are initialized by the opposite perpendicular field.
Here, we also observed a minimal hysteresis opening at B_x_ = 1 mT, which indicates that the canting effective field is close to 1 mT and also the switching chirality changes upon crossing
B_x_ = 1 mT. Next, we investigate how the canting angle affects the multi-state switching and ratchet effect. Clearly, the ratchet effect persists in all the switching curves with different
B_x_ (Fig. 6g), and the main difference from sample-45 is that the initial magnetic state (_R__A__H__E_) is no longer constant (Fig. 5) across the different symmetry-breaking fields in
sample-56. In fact, the initial state _R__m__i__n_ (Fig. 6h) is symmetric against B_x_ = 0. As for the ratchet effect, it is clear that once the SOT switches the moments to the other
direction, the opposite SOT sign does not switch the moments back to their initial state _R__m__i__n_ (Fig. 6g). Full B_x_-dependent switching curves with different current loop traverse
directions and initialization directions are shown in Supplementary Fig. S5. Due to the weak PMA in sample-56, the effective canting field is smaller (between 0 ~ 1 mT) than sample-45
across all the sets ([B_z_,_s__e__t_, B_x_]) of switching curves. Next, we investigate the effect of a much weaker canting on the switching phenomena. A strong PMA can be obtained with clear
exchange spring behavior from the magnetometry measurement in the OOP direction for single-shot co-sputtered GdCo with its composition close to the magnetic compensation - sample-13 (Fig.
7a). From the angle-dependent AHE field sweep measurement (Fig. 7b, c), the canting angle is close to 13∘. The discrepancy of _Δ__R__A__H__E_ between the two B_x_ = ± 2.5 mT switching
curves, when the moments are initialized by B_z_,_s__et_ = − 100 mT, is observable but much smaller (Fig. 7d) compared to sample-45 (Fig. 3b) and sample-56 (Fig. 6d). As for the other
initialization case (B_z_,_s__et_ = 100 mT), the _Δ__R__A__H__E_ are the same between the two B_x_ = ± 2.5 mT switching curves and their chiralities behave as expected correspondingly (Fig.
7e). Due to the weaker canting and strong PMA, minimal switching is observed at zero symmetry breaking field (Fig. 7f) and the switching chirality is again fixed. Although the canting is
weak, a clear SOT ratchet effect is observable across the different symmetry-breaking field switching loops (Fig. 7g). However, due to the strong PMA and weak canting, the switching curves
and multistate behavior are less tunable across the different in-plane symmetry breaking fields. For example, in sample-45, the switching curves as a function of in-plane field (B_x_)
resided in the _R__A__H__E_ range of 0 to 0.5 _Ω_ for B_x_ = − 10 mT, and as B_x_ increases, the switching curve moved up in _R__A__H__E_ values and end up in the range of 0.5 to 1.2 _Ω_ for
B_x_ = 10 mT. This can be characterized by the _R__m__i__n_ (Fig. 4d) and _R__m__a__x_ (Fig. 4d) in Fig. 4b. This is not the case for sample-13 where the switching curves do not move up by
more than 0.125 _Ω_ from B_x_ = − 10 mT to 10 mT in Fig. 7g and characterized by _R__m__i__n_ in Fig. 6h. For completeness, B_x_-dependent switching curves of different B_z_,_s__e__t_ and
different current start values are shown in Supplementary Fig. S6. A clear ratchet effect can be observed in all of the switching curves. Since the IP magnetization is crucial to
observations such as field-free switching, multistate and ratchet effect, we performed a simple B_y_ IP field dependent SOT switching experiment to determine whether a significant portion of
the IP magnetization lies along the _y_-direction, which is transverse to the current direction. According to Kong et al.42, if the IP magnetization lies in the direction transverse to the
current direction, strong SOT switching can be observed regardless of the applied transverse (B_y_) IP field. However, this is not the case for our system as minimal albeit non-zero SOT
switching can be observed as B_y_ increases (Supplementary Fig. S8). This indicates that the majority of the IP magnetization lies in the direction of the current (_x_) with a small tilt
toward the y-direction. We also studied the possibility of the memristor behavior23,34 in sample-13 since the strong PMA can lead to much easily observable SOT switching loops and
_R__A__H__E_ value changes. Due to the strong exchange spring effect in the OOP direction and the small canting, GdCo in sample-13 serves as an equivalent system as AFM/FM, which possesses a
small canting from the exchange bias and the exchange spring behavior from the coupling between the pinned interface AFM moments and FM moments. Indeed, the memristor behavior can be
observed in sample-13 with a small symmetry-breaking field to assist full switching (Supplementary Fig. S7). In addition, the spacing between the _R__A__H__E_ states achieved by the
different I_m__a__x_ values can be tuned by the symmetry-breaking field strength. DISCUSSION Our results have demonstrated that unconventional SOT switching behaviors, including multistate,
memristor, field-free, and ratchet SOT switching, can be achieved by engineering appropriate anisotropy-induced canting in ferrimagnetic Gd_x_Co100−_x_. In addition to the canting, the
presence of both a hard and a soft magnetic phase in each of the magnetic anisotropy directions (IP and OOP) exhibits characteristics of an exchange-spring system. At the same time, the
ratchet effect in SOT switching provides further confirmation of an exchange-spring system. The tunability of such multi-state and ratchet effects is highly dependent on the canting of the
magnetic anisotropy. A canting angle of ~ 45∘ is the ideal system to have tunable multistate (_R__A__H__E_) and large _Δ__R__A__H__E_, as it was demonstrated in this work. The ratchet SOT
effect is observed across all canting angles, and it exists regardless of the symmetry-breaking field or initialization direction. These diverse SOT switching effects can be useful for
applications such as neuromorphic computing43,44, multistate magnetic memory, and efficient magnetic memory. METHODS SAMPLE PREPARATION AND DEVICE FABRICATION Thin film Ta/GdCo/Pt
heterostructure is deposited by magnetron sputtering at room temperature. Specifically, the GdCo layer is deposited by co-sputtering Gd and Co elemental targets in one single shot with fixed
calibrated powers. Co power is fixed at 60 W, while Gd power is varied between 20 and 30 W for different concentrations. No pause or change of the Gd and Co sputtering powers during the
growth is implemented. The heterostructure is deposited on thermally oxidized silicon of 100 nm formed on a silicon substrate. All three samples have the stack design as Si substrate / SiO2
(100 nm) / Ta (8 nm) / Gd_x_Co100−_x_ (10 nm) / Pt (2 nm). The concentration of each Gd_x_Co100−_x_ sample is shown in Table 1. Hall bar devices are patterned with standard photolithography
and ion milling. Metal contacts to the device are fabricated through the lift-off process with electron beam evaporation of Ti (5 nm) / Au (80 nm). OXYGEN PLASMA TREATMENT AND DIFFUSION FOR
INDUCING MAGNETIC CANTING Oxygen plasma treatment is carried out with parameters including 50 W RF power, 180 mTorr oxygen, and 50 ∘C chuck in a Technics C Plasma Etching System in the
Berkeley Nanofabrication facility. Diffusion of oxygen is done under ambient conditions of room temperature and atmospheric pressure. More explanation can be found in Supplementary Note 2.
MAGNETOMETRY AND ANOMALOUS HALL MEASUREMENTS Thin film magnetometry measurements are done at room temperature with a Lakeshore 7400 series vibrating sample magnetometer in both in-plane and
out-of-plane directions. Analysis of the magnetometry result is done by subtracting out the diamagnetic signal from the silicon substrate and shifting the DC offset of the curve to zero
moments. The diamagnetic signal from the silicon is obtained by fitting a line in the saturated regime. Magnetization is calculated by dividing the measured moments by the volume of the thin
film GdCo. Angle-dependent anomalous Hall field sweep measurements are done under room temperature with a home-built setup that consists of an Amtek 7270 lock-in amplifier, a Keithey 6221
ac current source, a Lakeshore 475 Gaussmeter, and a GMW 5403 electromagnet driven by a kepco power supply that can produce a bi-polar magnetic field up to 330 mT. Magnetic fields are
applied with a hysteresis profile, and the AC current is fixed at a small value (500 μA), so minimal spin-orbit torque affects the magnetization. The current direction is fixed at the
_x_-direction and the magnetic field direction starts from the _z_-direction as 0∘ and traverses to the _x_-direction as 90∘, which is the in-plane direction. Analysis of the angle-dependent
anomalous Hall field sweep measurement involves the fitting a linear function to the data in the region above the coercive field for both up and down magnetic states. By extracting the
slope of such linear function, we can compare the angle of the easy-axis in our Gd_x_Co100−_x_ magnetic devices. PULSE-IV SWITCHING MEASUREMENTS Pulsed I–V switching measurements are carried
out with a home-built setup including a Keithley 6221 ac current source, a Keithley 2182a nanovoltmeter, a GMW 5403 electromagnet, a Lakeshore 475 Gaussmeter, and a custom-built rotating
dip stick chip carrier with electrical contacts. Keithley 6221 and 2182a are connected together in the pulsed-delta mode for simultaneous current sourcing and voltage measurement. All the
switching measurements are conducted with 200 μs pulse width and 60 μs source delay. Every pulse switching curve is obtained with a reset magnetic field applied in the ±
\(\hat{z}\)-direction at 100 mT before the current pulse sequence is applied. After the large set field is applied, the field is removed, and the sample is rotated back to in-plane
configuration with respect to the magnetic field application direction (\(\hat{x}\)-direction). The in-plane symmetry breaking field is set and kept on throughout the entire pulsed I–V
sequence. Voltage is measured in the transverse direction utilizing the anomalous Hall effect to read out the magnetic state. The SOT pulsed I–V measurements are done on Hall bar devices
with 6 μm width and 40 μm length. The Hall voltage arms of the Hall bar devices are 2.5 μm in width and 14 μm in length. DATA AVAILABILITY The authors declare that all data supporting the
claims of this work including every data point collected are included in the main text, methods and the supplementary information. All raw data in table format are available at
https://doi.org/10.6084/m9.figshare.27020875. Additional physical materials can be requested from S.S. ([email protected]) or C.-H.H. ([email protected]). REFERENCES * Salahuddin, S., Ni,
K. & Datta, S. The era of hyper-scaling in electronics. _Nature Electronics_ 1, 442–450 (2018). Article Google Scholar * Dieny, B. et al. Opportunities and challenges for spintronics
in the microelectronics industry. _Nature Electronics_ 3, 446–459 (2020). Article Google Scholar * Han, W., Maekawa, S. & Xie, X.-C. Spin current as a probe of quantum materials.
_Nature Materials_ 19, 139–152 (2020). Article ADS PubMed CAS Google Scholar * Trier, F. et al. Oxide spin-orbitronics: spin-charge interconversion and topological spin textures.
_Nature Reviews Materials_ 7, 258–274 (2022). Article ADS Google Scholar * Tsai, H. et al. Electrical manipulation of a topological antiferromagnetic state. _Nature_ 580, 608–613 (2020).
Article ADS PubMed CAS Google Scholar * Noël, P. et al. Non-volatile electric control of spin-charge conversion in a SrTiO3 Rashba system. _Nature_ 580, 483–486 (2020). Article ADS
PubMed Google Scholar * Luo, Z. et al. Current-driven magnetic domain-wall logic. _Nature_ 579, 214–218 (2020). Article ADS PubMed CAS Google Scholar * Gao, S. et al. Fractional
antiferromagnetic skyrmion lattice induced by anisotropic couplings. _Nature_ 586, 37–41 (2020). Article ADS PubMed CAS Google Scholar * Jani, H. et al. Antiferromagnetic half-skyrmions
and bimerons at room temperature. _Nature_ 590, 74–79 (2021). Article PubMed CAS Google Scholar * Caretta, L. et al. Relativistic kinematics of a magnetic soliton. _Science_ 370,
1438–1442 (2020). Article ADS MathSciNet PubMed CAS Google Scholar * Wang, Y. et al. Magnetization switching by magnon-mediated spin torque through an antiferromagnetic insulator.
_Science_ 366, 1125–1128 (2019). Article ADS PubMed CAS Google Scholar * Kim, S. K. et al. Ferrimagnetic spintronics. _Nat. Mater._ 21, 24–34 (2022). Article ADS PubMed CAS Google
Scholar * Néel, M. L. Propriétés magnétiques des ferrites ; ferrimagnétisme et antiferromagnétisme. _Ann. De Phys._ 12, 137–198 (1948). Article ADS Google Scholar * Chaudhari, P., Cuomo,
J. J. & Gambino, R. J. Amorphous metallic films for bubble domain applications. _IBM J. Res. Dev._ 17, 66–68 (1973). Article CAS Google Scholar * Kim, D.-H. et al. Bulk
Dzyaloshinskii-Moriya interaction in amorphous ferrimagnetic alloys. _Nat. Mater._ 18, 685–690 (2019). Article ADS PubMed CAS Google Scholar * Caretta, L. et al. Fast current-driven
domain walls and small skyrmions in a compensated ferrimagnet. _Nat. Nanotechnol._ 13, 1154–1160 (2018). Article ADS PubMed CAS Google Scholar * Roschewsky, N., Lambert, C.-H. &
Salahuddin, S. Spin-orbit torque switching of ultralarge-thickness ferrimagnetic GdFeCo. _Phys. Rev. B_ 96, 064406 (2017). Article ADS Google Scholar * Stanciu, C. D. et al. All-optical
magnetic recording with circularly polarized light. _Phys. Rev. Lett._ 99, 047601 (2007). Article ADS PubMed CAS Google Scholar * Brunsch, A. & Schneider, J. Perpendicular magnetic
anisotropy in evaporated amorphous GdCo films. _J. Appl. Phys._ 48, 2641–2643 (1977). Article ADS CAS Google Scholar * Taylor, R. C. & Gangulee, A. Magnetization and magnetic
anisotropy in evaporated GdCo amorphous films. _J. Appl. Phys._ 47, 4666–4668 (1976). Article ADS CAS Google Scholar * Yu, G. et al. Switching of perpendicular magnetization by
spin-orbit torques in the absence of external magnetic fields. _Nat. Nanotechnol._ 9, 548–554 (2014). Article ADS PubMed CAS Google Scholar * You, L. et al. Switching of perpendicularly
polarized nanomagnets with spin orbit torque without an external magnetic field by engineering a tilted anisotropy. _Proc. Nat Acad. Sci. USA_ 112, 10310–10315 (2015). Article ADS PubMed
PubMed Central CAS Google Scholar * Fukami, S., Zhang, C., Duttagupta, S., Kurenkov, A. & Ohno, H. Magnetization switching by spin-orbit torque in an antiferromagnet-ferromagnet
bilayer system. _Nat. Mater._ 15, 535–541 (2016). Article ADS PubMed CAS Google Scholar * Hellman, F. & Gyorgy, E. M. Growth-induced magnetic anisotropy in amorphous Tb-Fe. _Phys.
Rev. Lett._ 68, 1391–1394 (1992). Article ADS PubMed CAS Google Scholar * Harris, V. G., Aylesworth, K. D., Das, B. N., Elam, W. T. & Koon, N. C. Structural origins of magnetic
anisotropy in sputtered amorphous Tb-Fe films. _Phys. Rev. Lett._ 69, 1939–1942 (1992). Article ADS PubMed CAS Google Scholar * Kim, H. et al. Field-free switching of magnetization by
tilting the perpendicular magnetic anisotropy of gd/co multilayers. _Adv. Funct. Mater._ 32, 2112561 (2022). Article CAS Google Scholar * Ren, X. et al. Field-free switching of
magnetization induced by spin-orbit torque in pt/cogd/pt thin film. _Appl. Phys. Lett._ 120, 252403 (2022). Article ADS CAS Google Scholar * Wu, H. et al. Chiral symmetry breaking for
deterministic switching of perpendicular magnetization by spin-orbit torque. _Nano Lett._ 21, 515–521 (2021). Article ADS PubMed CAS Google Scholar * Zheng, Z. et al. Field-free
spin-orbit torque-induced switching of perpendicular magnetization in a ferrimagnetic layer with a vertical composition gradient. _Nat. Commun._ 12, 4555 (2021). Article ADS PubMed PubMed
Central CAS Google Scholar * Oh, Y. W. et al. Field-free switching of perpendicular magnetization through spin-orbit torque in antiferromagnet/ferromagnet/oxide structures. _Nat.
Nanotechnol._ 11, 878–884 (2016). Article ADS PubMed PubMed Central CAS Google Scholar * van den Brink, A. et al. Field-free magnetization reversal by spin-hall effect and exchange
bias. _Nat. Commun._ 7, 10854 (2016). Article PubMed PubMed Central Google Scholar * Lin, P.-H. et al. Manipulating exchange bias by spin-orbit torque. _Nat. Mater._ 18, 335–341 (2019).
Article ADS PubMed CAS Google Scholar * Peng, S. et al. Exchange bias switching in an antiferromagnet/ferromagnet bilayer driven by spin-orbit torque. _Nat. Electron._ 3, 757–764
(2020). Article CAS Google Scholar * Yun, J. et al. Tailoring multilevel-stable remanence states in exchange-biased system through spin-orbit torque. _Adv. Funct. Mater._ 30, 1909092
(2020). Article CAS Google Scholar * Huang, Y. H. et al. A spin-orbit torque ratchet at ferromagnet/antiferromagnet interface via exchange spring. _Adv. Funct. Mater._ 32, 1–8 (2022).
Google Scholar * Chen, X. et al. Electric field control of néel spin-orbit torque in an antiferromagnet. _Nat. Mater._ 18, 931–935 (2019). Article ADS PubMed CAS Google Scholar *
Gomonay, O., Kläui, M. & Sinova, J. Manipulating antiferromagnets with magnetic fields: Ratchet motion of multiple domain walls induced by asymmetric field pulses. _Appl. Phys. Lett._
109, 142404 (2016). Article ADS Google Scholar * Joo, S., Alemayehu, R. S., Choi, J.-G., Park, B.-G. & Choi, G.-M. Magnetic anisotropy and damping constant of ferrimagnetic GdCo alloy
near compensation point. _Materials_ 14, 2604 (2021). Article ADS PubMed PubMed Central CAS Google Scholar * Ceballos, A., Charilaou, M., Molina-Ruiz, M. & Hellman, F. Coexistence
of soft and hard magnetic phases in single layer amorphous Tb-Co thin films. _J. Appl. Phys._ 131, 33901 (2022). Article CAS Google Scholar * Song, M. et al. Spin-orbit torque-driven
magnetic switching of Co/Pt-CoFeB exchange spring ferromagnets. _IEEE Trans. Magn._ 55, 1–4 (2019). Google Scholar * Vélez, S. et al. Current-driven dynamics and ratchet effect of skyrmion
bubbles in a ferrimagnetic insulator. _Nat. Nanotechnol._ 17, 834–841 (2022). Article ADS PubMed Google Scholar * Kong, W. J. et al. Spin-orbit torque switching in a T-type magnetic
configuration with current orthogonal to easy axes. _Nat. Commun._ 10, 1–7 (2019). Article ADS Google Scholar * Liu, S. et al. Controllable reset behavior in domain wall-magnetic tunnel
junction artificial neurons for task-adaptable computation. _IEEE Magn. Lett._ 12, 1–5 (2021). Article Google Scholar * Zhou, J. et al. Spin-orbit torque-induced domain nucleation for
neuromorphic computing. _Adv. Mater._ 33, 1–8 (2021). Article Google Scholar Download references ACKNOWLEDGEMENTS This work was primarily supported by the U.S. Department of Energy, Office
of Science, Office of Basic Energy Sciences, Materials Sciences and Engineering Division, under Contract No. DE-AC02-05-CH11231 within the Non-Equilibrium Magnetism program (MSMAG). This
work was also supported in part by the ASCENT center, one of the six centers within the JUMP initiative jointly supported by DARPA and SRC. In addition, support from NSF E3S center is
gratefully acknowledged. This work was performed in part at the Berkeley Marvell Nanofabrication Laboratory at the University of California, Berkeley, and their staff research support is
greatly appreciated. AUTHOR INFORMATION AUTHORS AND AFFILIATIONS * Department of Electrical Engineering and Computer Science, University of California, Berkeley, California, USA Cheng-Hsiang
Hsu, Hannah Calzi Kleidermacher, Shehrin Sayed & Sayeef Salahuddin * Materials Science Division, Lawrence Berkeley National Laboratory, Berkeley, California, USA Cheng-Hsiang Hsu,
Shehrin Sayed & Sayeef Salahuddin * Department of Physics, University of California, Berkeley, California, USA Miela J. Gross Authors * Cheng-Hsiang Hsu View author publications You can
also search for this author inPubMed Google Scholar * Miela J. Gross View author publications You can also search for this author inPubMed Google Scholar * Hannah Calzi Kleidermacher View
author publications You can also search for this author inPubMed Google Scholar * Shehrin Sayed View author publications You can also search for this author inPubMed Google Scholar * Sayeef
Salahuddin View author publications You can also search for this author inPubMed Google Scholar CONTRIBUTIONS The original idea was conceived by C.-H.H. and the research project is
supervised by S.Salahuddin. Film preparation was performed by C.-H.H. and M.J.G.; device fabrication was performed by C.-H.H.; magnetic characterization was performed by C.-H.H. and H.C.K.;
transport and switching measurement was performed by C.-H.H.; data analysis was done by C-H.H. under the supervision of S.Salahuddin; C.-H.H. and S.Salahuddin co-wrote the manuscript. All
authors contributed to discussions and commented on the manuscript. CORRESPONDING AUTHORS Correspondence to Cheng-Hsiang Hsu or Sayeef Salahuddin. ETHICS DECLARATIONS COMPETING INTERESTS The
authors declare no competing interests. PEER REVIEW PEER REVIEW INFORMATION _Nature Communications_ thanks Shouzhong Peng, and the other, anonymous, reviewer(s) for their contribution to
the peer review of this work. A peer review file is available. ADDITIONAL INFORMATION PUBLISHER’S NOTE Springer Nature remains neutral with regard to jurisdictional claims in published maps
and institutional affiliations. SUPPLEMENTARY INFORMATION SUPPLEMENTARY INFORMATION PEER REVIEW FILE RIGHTS AND PERMISSIONS OPEN ACCESS This article is licensed under a Creative Commons
Attribution 4.0 International License, which permits use, sharing, adaptation, distribution and reproduction in any medium or format, as long as you give appropriate credit to the original
author(s) and the source, provide a link to the Creative Commons licence, and indicate if changes were made. The images or other third party material in this article are included in the
article’s Creative Commons licence, unless indicated otherwise in a credit line to the material. If material is not included in the article’s Creative Commons licence and your intended use
is not permitted by statutory regulation or exceeds the permitted use, you will need to obtain permission directly from the copyright holder. To view a copy of this licence, visit
http://creativecommons.org/licenses/by/4.0/. Reprints and permissions ABOUT THIS ARTICLE CITE THIS ARTICLE Hsu, CH., Gross, M.J., Kleidermacher, H.C. _et al._ Tunable multistate field-free
switching and ratchet effect by spin-orbit torque in canted ferrimagnetic alloy. _Nat Commun_ 15, 8713 (2024). https://doi.org/10.1038/s41467-024-52834-0 Download citation * Received: 19
December 2022 * Accepted: 20 September 2024 * Published: 08 October 2024 * DOI: https://doi.org/10.1038/s41467-024-52834-0 SHARE THIS ARTICLE Anyone you share the following link with will be
able to read this content: Get shareable link Sorry, a shareable link is not currently available for this article. Copy to clipboard Provided by the Springer Nature SharedIt content-sharing
initiative