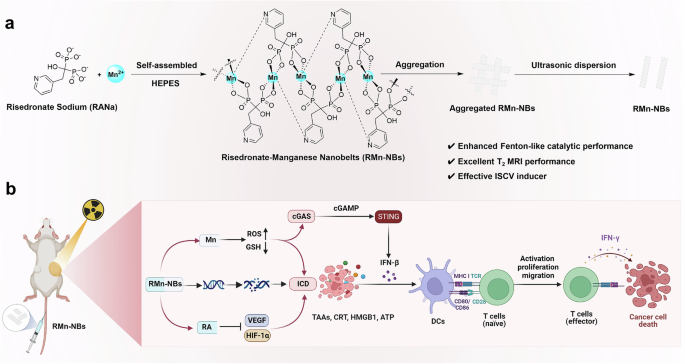
Two-dimensional coordination risedronate-manganese nanobelts as adjuvant for cancer radiotherapy and immunotherapy
- Select a language for the TTS:
- UK English Female
- UK English Male
- US English Female
- US English Male
- Australian Female
- Australian Male
- Language selected: (auto detect) - EN
Play all audios:

The irradiated tumor itself represents an opportunity to establish endogenous in situ vaccines. However, such in situ cancer vaccination (ISCV) triggered by radiation therapy (RT) alone is
very weak and hardly elicits systemic anticancer immunity. In this study, we develop two-dimensional risedronate-manganese nanobelts (RMn-NBs) as an adjuvant for RT to address this issue.
RMn-NBs exhibit good T2 magnetic resonance imaging performance and enhanced Fenton-like catalytic activity, which induces immunogenic cell death. RMn-NBs can inhibit the HIF-1α/VEGF axis to
empower RT and synchronously activate the cGAS/STING pathway for promoting the secretion of type I interferon, thereby boosting RT-triggered ISCV and immune checkpoint blockade therapy
against primary and metastatic tumors. RMn-NBs as a nano-adjuvant for RT show good biocompatibility and therapeutic efficacy, presenting a promising prospect for cancer radiotherapy and
immunotherapy.
Recent researches from preclinical and clinical trials suggest that radiation therapy (RT) of tumors can inherently generate in situ cancer vaccination (ISCV), potentially triggering
systemic anticancer adaptive immune response1,2,3. RT-triggered ISCV employs localized treatment at the lesion site to induce immunogenic cell death (ICD), striving to release
tumor-associated antigens (TAAs) and immune adjuvants to initiate systemic anticancer immunnity4,5,6. Unfavorably, RT often exacerbates intrinsic hypoxia with the overexpression of hydrogen
peroxide (H2O2) and glutathione (GSH) in tumor tissue, which results in a significantly reduced release of TAAs, thereby hindering systemic anticancer immune response7,8,9. Moreover, tumor
cells are inclined to upregulate hypoxia-inducible factor 1α (HIF-1α) for stimulating the expression of vascular endothelial growth factor A (VEGF-A) to promote tumor growth and metastasis
in the process of RT10,11. Targeting inhibition of HIF-1α/VEGF axis can relieve tumor hypoxia and suppress tumor angiogenesis, thus promoting the release of TAAs to improve the
immunogenicity of tumor cells and make them easy to be attacked by immune system12,13,14,15. However, this strategy can not effectively induce the secretion of type I interferon (IFN) that
is involved in ICD and indispensable for cross-presentation of TAAs to promote protective antitumor CD8+ T cell immunity, acting as a bridge molecule in linking innate and adaptive immune
responses to prime ISCV16,17,18,19. Cyclic GMP-AMP synthase (cGAS)-stimulator of interferon genes (STING) pathway can directly drive the secretion of type I IFN under the stimulation of
damaged DNA to promote the cross-presentation of TAAs and activate systemic anti-tumor immune responses20,21. Therefore, constructing a cancer nanoadjuvant with the versatility of both
inhibiting HIF-1α/VEGF axis and activating cGAS-STING pathway is expected for enhanced RT-triggered ISCV.
Self-assembly strategy based on clinical drugs and functional trace metal ions provides an avenue to construct visual in situ cancer nanoadjuvant22,23,24. This strategy can not only avoid
the use of nanocarriers to potentially accelerate their clinical transformation, but also reduce toxicity of free clinical drugs and metal ions, improve their pharmacokinetic behaviors and
physiochemical properties, resulting in multifunctional theranostics22,23,24,25,26,27. Risedronate as the first-line treatment drug for osteoporosis has been recently proved to be capable of
alleviating hypoxia and inhibiting tumor angiogenesis by targeting inhibition of HIF-1α/VEGF signaling pathway28,29. Additionally, recent studies have shown that Mn2+ released from
membrane-enclosed organelles during viral infections can directly bind to cGAS for triggering cGAS-STING pathway to improve type I IFN secretion, which can induce maturation of DCs and
enhance TAAs presentation for promoting anticancer immune responses30,31. Mn2+ can also be used for T2 magnetic resonance imaging (MRI)32. However, free risedronate and Mn2+ exhibit severe
acute toxicity and poor tumor accumulation capabilities, which hinder their efficacy in inhibiting HIF-1α/VEGF and activating cGAS-STING signaling pathway, respectively28,29,30,31.
Furthermore, free Mn2+ in vivo always gives unsatisfactory MRI performance32. Self-assembly of risedronate and Mn2+ to form a self-delivering and tumor microenvironment (TME)-responsive
visual nanoadjuvant should be able to efficiently inhibit HIF-1α/VEGF axis and activate cGAS-STING pathway with the guide of its enhanced MRI for promoting RT-triggered ISCV.
In this work, risedronate-manganese nanobelts (RMn-NBs) are constructed through coordination-driven self-assembly of risedronate and Mn2+ as a self-delivery visual nanoadjuvant to enhance
RT-induced ISCV (Fig. 1a). RMn-NBs with negative surface charges and ultrathin nanobelt morphology exhibit excellent T2 MRI characteristics with a transverse relaxivity (r2) value of 11.25
mM−1 S−1 at 7.0 Tesla, slightly surpassing that of commercial Fe3O4 T2-MRI agents (r2 = 11.17 mM−1 S−1, 7.0 Tesla). The good T2-MRI performance of RMn-NBs grants them to be used for visually
studying their own in vivo pharmacokinetic behaviors for precise RT. Moreover, RMn-NBs present the TME-responsive release of risedronate, which specifically inhibits HIF−1α/VEGF axis to
substantially promote the release of TAAs triggered by RT. RMn-NBs also demonstrate excellent Fenton-like catalytic property, enabling them to amplify RT-mediated oxidative stress for
inducing potent ICD. The released Mn2+ from RMn-NBs can further activate the cGAS-STING pathway, promoting the secretion of type I IFN and boosting the effects of RT-triggered ISCV.
RMn-NB-sensitized RT as an ISCV approach can efficiently augment the efficacy of CD8+ T cell-dependent antitumor immune responses and immune checkpoint blockade (ICB) therapy against primary
and metastatic tumors (Fig. 1b).
a Schematically illustrating the preparation of RMn-NBs. b The mechanism of RMn-NBs as a visual nanoadjuvant for in situ vaccination of RT. Reactive oxygen species (ROS), glutathione (GSH),
calreticulin (CRT), high mobility group protein B1 (HMGB1), adenosine triphosphate (ATP), dendritic cells (DCs), interferon-beta (IFN-β), interferon-gama (IFN-γ), hypoxia-inducible factor-1α
(HIF-1α), vascular endothelial growth factor (VEGF), cyclic GMP-AMP synthase (cGAS), cyclic GMP-AMP (cGAMP), stimulator of interferon genes (STING). Created in BioRender. Luo, Z. (2024)
BioRender.com/u37f450.
Manganese chloride (MnCl2) and isomolar risedronate sodium (RANa) are reacted under room temperature to form nanosized risedronate-manganese coordination complex, referred as RMn-NBs.
RMn-NBs aqueous suspension exhibits a zeta potential of −13.86 ± 0.88 mV (Supplementary Fig. 1a). A clear Tyndall effect can be observed for RMn-NBs aqueous suspension (Fig. 2a), suggesting
that RMn-NBs exist as a nanoscale form in aqueous solution. Transmission electron microscope (TEM) imaging in Fig. 2b shows that RMn-NBs have an obvious nanobelt morphology with the width of
~5 nm and the length of ~180 nm. The average particle size of RMn-NBs is evaluated to be 177.1 ± 16.2 nm by dynamic light scattering (DLS) (Supplementary Fig. 1b), aligning with the length
observed from TEM. The changes of their average particle sizes with different time indicate the good stability of RMn-NBs in deionized water (25 °C), phosphate buffered saline (PBS, 25 °C),
and culture medium containing 10% fetal bovine serum (FBS, 37 °C) (Supplementary Fig. 1c). The ultraviolet-visible (UV-Vis) spectra in Supplementary Fig. 1d shows that RANa has a
characteristic absorption peak at ~260 nm, which red-shifts to ~270 nm after coordination with Mn2+. Fourier transform infrared (FTIR) spectra of RMn-NBs in Supplementary Fig. 1e shows the
characteristic absorption peaks of MnCl2 (~1130 cm−1) and RANa (~993, ~1053, and ~1635 cm−1)33,34, confirming the coordination interaction of Mn2+ and RANa to form RMn-NBs. The Powder X-ray
diffraction (PXRD) analysis of RMn-NBs reveals distinct diffraction peaks, suggesting potential polymorphism in these supramolecular nanobelts (Fig. 2c). This result indicates that the
assembly process of RMn-NBs is systematic and orderly. Energy dispersive spectroscopy (EDS) mapping (Fig. 2d) confirms the presence of N, O, P, and Mn elements within RMn-NBs.
High-resolution Mn 2p X-ray photoelectron spectroscopy (XPS) spectrum shows two binding energies at 639.97 and 651.45 eV attributed to Mn2+, and two binding energies at 638.56 and 643.39 eV
can be attributed to the formation of lower reduction-state Mn species due to self-assembly of Mn2+ with RANa (Fig. 2e and Supplementary Fig. 1f)35. Two binding energies at 529.95 and 531.38
eV in the high-resolution O 1 s XPS spectrum of RANa (Supplementary Fig. 1g) respectively redshift to 530.48 and 531.92 eV (Fig. 2f) after self-assembly of RANa with Mn2+ to form RMn-NBs,
suggesting the formation of Mn-O bonds36. Similarly, two binding energies at 398.62 and 400.68 eV in the high-resolution N 1 s XPS spectrum of RANa respectively blueshift to 397.18 and
399.23 eV after self-assembly of RANa with Mn2+ to form RMn-NBs (Fig. 2g and Supplementary Fig. 1h), providing strong evidence for the formation of Mn-N bonds37. The self-assembly molar
ratio of Mn2+ and RANa in RMn-NBs is about 1:1 by XPS elemental analysis of lyophilized RMn-NBs powder (Supplementary Table 1).
a Tyndall effect of RMn-NBs aqueous suspension. b TEM imaging of RMn-NBs, scale bar = 50 nm. This experiment is repeated twice independently with similar results. c PXRD of MnCl2, RANa,
RMn-NBs. This experiment is repeated twice independently with similar results. d EDS mapping of RMn-NBs, scale bar = 50 nm. This experiment is repeated twice independently with similar
results. High-resolution XPS spectra of (e) Mn 2p, (f) O 1 s, and (g) N 1 s of RMn-NBs (n = 3 independent samples). Arbitrary units (a. u.). h Electron spin resonance (ESR) spectra of ROS
detection for different treatments, arbitrary units (a. u.). 2,2,6,6-tetramethyl-4-piperidinone (TEMP) is used as a trap for ROS (n = 3 independent samples). i Time-dependent GSH consumption
after treating with RMn-NBs in a simulated tumor microenvironment (n = 3 independent samples). j Release profiles of RANa from RMn-NBs in different
N-2-hydroxyethylpiperazine-N-ethane-sulphonicacid (HEPES) buffer solutions (pH = 7.4, 6.5, 5.5) containing 10% serum in vitro, respectively (n = 3 independent samples). All data are shown as
mean ± SD. Statistical analysis is performed using two-tailed Student’s t-test for comparing two groups. p > 0.05 represent nonsignificance, while p 0.05 represent nonsignificance, while p
0.05 represent nonsignificance, while p 0.05 represent nonsignificance, while p 0.05 represent nonsignificance, while p 0.05 represent nonsignificance, while p 0.05 represent
nonsignificance, while p 7.25. As the pH value gradually decreases, the phosphate partially becomes mono-protonated (6.12 0.05 represent nonsignificance and p