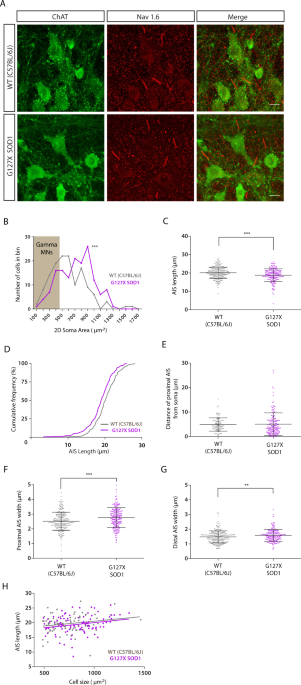
Shorter axon initial segments do not cause repetitive firing impairments in the adult presymptomatic g127x sod-1 amyotrophic lateral sclerosis mouse
- Select a language for the TTS:
- UK English Female
- UK English Male
- US English Female
- US English Male
- Australian Female
- Australian Male
- Language selected: (auto detect) - EN
Play all audios:

ABSTRACT Increases in axonal sodium currents in peripheral nerves are some of the earliest excitability changes observed in Amyotrophic Lateral Sclerosis (ALS) patients. Nothing is known,
however, about axonal sodium channels more proximally, particularly at the action potential initiating region - the axon initial segment (AIS). Immunohistochemistry for Nav1.6 sodium
channels was used to investigate parameters of AISs of spinal motoneurones in the G127X SOD1 mouse model of ALS in adult mice at presymptomatic time points (~190 days old). I_n vivo_
intracellular recordings from lumbar spinal motoneurones were used to determine the consequences of any AIS changes. AISs of both alpha and gamma motoneurones were found to be significantly
shorter (by 6.6% and 11.8% respectively) in G127X mice as well as being wider by 9.8% (alpha motoneurones). Measurements from 20–23 day old mice confirmed that this represented a change
during adulthood. Intracellular recordings from motoneurones in presymptomatic adult mice, however, revealed no differences in individual action potentials or the cells ability to initiate
repetitive action potentials. To conclude, despite changes in AIS geometry, no evidence was found for reduced excitability within the functional working range of firing frequencies of
motoneurones in this model of ALS. SIMILAR CONTENT BEING VIEWED BY OTHERS EXCESSIVE HOMEOSTATIC GAIN IN SPINAL MOTONEURONS IN A MOUSE MODEL OF AMYOTROPHIC LATERAL SCLEROSIS Article Open
access 03 June 2020 BIMODAL REGULATION OF AXONAL TRANSPORT BY THE GDNF-RET SIGNALLING AXIS IN HEALTHY AND DISEASED MOTOR NEURONS Article Open access 07 July 2022 HYPEREXCITABILITY IN YOUNG
IPSC-DERIVED _C9ORF72_ MUTANT MOTOR NEURONS IS ASSOCIATED WITH INCREASED INTRACELLULAR CALCIUM RELEASE Article Open access 05 May 2022 INTRODUCTION Amyotrophic Lateral Sclerosis (ALS) is a
fatal neurodegenerative disease which preferentially affects motoneurones in the brain and spinal cord. Although a number of different mutations have been found to account for a small
proportion of cases, the underlying pathophysiology of the disease is not fully understood and there is currently no cure. Riluzole, the only established treatment, has only modest effects
on survival time1,2,3,4,5 and blocks glutamatergic neurotransmission and Na+ and Ca2+ channels, indicating that excitability plays a role in the degenerative process in ALS. Threshold
tracking techniques have suggested a hyperexcitability of motoneurone axons in ALS patients consisting of an increase in sodium currents occurring with a decrease in potassium
currents6,7,8,9,10,11,12,13,14. The magnitude of the ion current changes appears to correlate with disease progression15, with excitability changes occurring before detectable axon loss7,15.
In humans, the cell body and proximal axons of motoneurones, however, are largely inaccessible, preventing direct investigations of sodium and potassium channel activity centrally in ALS
patients. This has therefore been investigated _in vitro_ in motoneurones cultured from induced pluripotent stem cells (iPSCs) from ALS patients with genetic forms of the disease including
superoxide dismutase 1 (SOD1), C9orf72 repeat expansions, TAR DNA binding proteins (TARDBP) and fused-in-sarcoma (FUS) mutations16,17,18,19. Increased action potential firing is observed at
early stages after plating16,19 due to reductions in delayed rectifier potassium currents19 and increases in peak sodium currents16. At later time points post-plating however, motoneurones
exhibit deficits in repetitive firing16,18 due to elevated potassium currents and attenuated sodium currents17 or attenuations in both16,18. Motoneurones derived from human iPSCs have been
shown, however, to be more similar to fetal spinal tissue than to adult spinal motoneurones20. Furthermore, experiments exposing wild type cultured rodent motoneurones to astrocytes
harbouring SOD1 or TDP43 mutations suggest that local astrocytes may be necessary to drive excitotoxic increases in persistent inward sodium currents21,22. _In vitro_ investigations of
cultured motoneurones from the transgenic G93A SOD1 mouse model of the disease have shown increases in persistent sodium currents23,24 with sodium channels displaying a faster recovery from
fast inactivation than controls25. Recordings from neonatal spinal slice preparations from the same mice have also confirmed an early increase in both Na+ and Ca2+ persistent inward currents
(PICs)26. ALS however, is an adult onset disorder, therefore it is crucial to establish whether the motoneurones exhibit abnormal excitability in adulthood. Furthermore, it is important to
determine this _in vivo_. Our previous _in vivo_ investigations in the adult G127X SOD1 mouse model of ALS have confirmed a presymptomatic increase in Na+ current in distal peripheral motor
axons27 and a disruption of axonal potassium channels related to a breakdown of nodal organisation in the ventral roots of these mice, assumed to be indicative of axonal degeneration28.
Electrophysiological recordings have, however, provided no evidence for a decrease of potassium channels centrally, even at the symptomatic stage28. In the current experiments we now focus
on central sodium channels in the same transgenic mouse model at pre-symptomatic adult time points with a particular focus on the axon initial segment (AIS). We hypothesized the AIS to be
affected for a number of reasons. First, activity dependent plasticity of the AIS _in vitro_ has been shown to be driven by L-type calcium channel activity29,30 and we have previously shown
an increase in PICs mediated by these channels in this model in pre-symptomatic adults31. Second, reductions in AIS length have been shown to impair repetitive firing32 and recordings in the
adult G93A SOD1 mouse model of ALS have suggested impairments in repetitive firing in the motoneurones33. Finally, anatomical investigations of post-mortem ALS patients have also shown a
swelling of proximal motoneurone axons (including AISs) compared to controls34,35. In the present experiments immunohistochemistry was used to label Nav1.6 sodium channels at AISs of spinal
motoneurones in adult presymptomatic G127X SOD1 mice combined with _in vivo_ intracellular recording to identify the functional significance of any changes. RESULTS ALPHA MOTONEURONE AXON
INITIAL SEGMENTS ARE SHORTER AND WIDER IN ADULT G127X MICE Axon initial segments were immunohistochemically labelled using antibodies against Nav1.6 (the main sodium channel subtype found at
the AIS) and antibodies against choline acetyltransferase (ChAT) to label spinal motoneurones in the ventral horn of the lumbar spinal cord in adult (~193 day old) presymptomatic ALS mice
and aged-matched wild type (WT) mice (more details of all mice used can be found in Table 1). Examples of motoneurone AISs in WT and G127X mice are shown in Fig. 1A. Only AISs connected to
ChAT immunoreactive motoneurones were measured. For those motoneurones with the entire soma contained within the 50 µm thick tissue section, the 2D area was calculated using the maximum and
minimum diameters. AISs belonging to soma with areas smaller than 485 µm2 (gamma motoneurone) were taken out for separate analysis and all remaining cells were assumed to be alpha
motoneurones (Fig. 1B). The results are summarised in Table 2 and the spread of individual data points can be seen in the scatter plots shown in Fig. 1C,E–H. AISs of alpha motoneurones were
slightly (6.6%) but significantly shorter in the G127X mice (Fig. 1C). When this data is displayed as cumulative frequency fractions it can be seen that this difference is not due to a
selective loss at one end of the distribution but an overall shift of all values in the G127X mice towards shorter lengths (Fig. 1D). The mean distance of the AIS from the soma was not
statistically significant, (Fig. 1E) but AISs were significantly wider in G127X mice at both their proximal and distal ends (by 9.8% and 7.2% respectively, Fig. 1F,G). The soma of the alpha
motoneurones were significantly (10.7%) larger in the G127X mice than WT. To determine if this was, in anyway, related to the changes in AIS parameters, linear regressions were performed
between soma size and AIS length, distal width and distance from soma respectively. Surprisingly, there was no significant relationship between soma size and any of the AIS parameters for
both groups except one feature. In the G127X mice there was a significant relationship between soma size and AIS length with smaller motoneurones having shorter AISs (Fig. 1H). This suggests
that the difference in AIS length between WT and G127X mice was not simply due to the motoneurones being larger in the G127X group. Linear regression also showed that in the WT mice longer
AISs were usually further from the cell body (data not shown). This relationship was absent in the G127X mice. GAMMA MOTONEURONE AISS ARE SHORTER AND THINNER IN ADULT G127X MICE Differences
were also observed for AISs of gamma motoneurones (Table 3, Fig. 2A–D) although a smaller sample was obtained. AISs from G127X mice were on average 11.8% shorter than WT (Fig. 2B). Again,
when the length data is displayed as cumulative frequency fractions it can be seen that this difference is not due to a selective loss at one end of the distribution but an overall shift of
all values in the G127X mice towards shorter lengths (Fig. 2C). AISs from G127X mice were also 16.6% thinner proximally (Fig. 2D), but no significant differences were observed for either
distal width (Fig. 2E) or distance from soma (Fig. 2F). Given that size was used as an identifying feature for gamma motoneurones, size was not investigated further. MINIMAL MOTONEURONE LOSS
IS OBSERVED AT THIS AGE IN THE ADULT G127X MICE To determine whether changes in AISs of both alpha and gamma motoneurones were due to a selective cell loss we quantified cell loss at this
age in 5 presymptomatic G127X and 4 WT mice of a similar age using ChAT labelling (Fig. 3A). The number of motoneurones was counted in every 10th transverse section from the lumbar spinal
cord and aligned as described in the methods. The mean number of motoneurones at each level for both groups is shown in Fig. 3B. From this, no clear differences could be observed confirming
that there is not an obvious loss of motoneurones at this age in G127X mice. The mean number of motoneurones per section was 49.12 (WT) and 46.53 (G127X) which was not significantly
different (P = 0.2857, Mann Whitney, U = 5). SHORTER AISS OF ALPHA MOTONEURONES REPRESENT A DEVELOPMENTAL CHANGE IN ADULT G127X MICE, A FEATURE NOT OBSERVED IN WT MICE To investigate whether
the changes that we observed in the AISs of G127X mice represent plastic changes during adulthood or exist from birth we measured AISs in ~22-day old WT and G127X mice. Examples are shown
in Fig. 4A, AIS parameters are summarised in Table 4 and the spread of individual data points can be seen in the scatter plots shown in Fig. 4. AISs were actually slightly longer (by 3.9%)
in the 22-day old G127X mice compared with WT (Fig. 4C). Displayed as cumulative frequency fractions, the data suggests that in the G127X population there is a greater number of longer AISs
(Fig. 4D). AISs in the G127X mice were also significantly thinner proximally (by 6.4%, Fig. 4E) but not distally (Fig. 4F) and further from the soma (Fig. 4G) compared to WT. So, although
the AISs of the motoneurones are different from WT at a young age in the G127X mice, these differences are not in the same direction as observed in adults at ages close to but before symptom
onset. To evaluate how the changes in the AIS parameters between ~22 and ~193 days on G127X mice represent normal, abnormal or enhanced development we first compared the parameters between
WT mice at ~22 days and the WT adults. How the different parameters of the AIS in the adult WT and G127X mice have changed relative to the same parameters at ~22 day (zero on Y axis) is
illustrated in Fig. 4H. From this it can be seen that for most parameters the magnitude of the change is different between WT and G127X but in the same direction except length. In adult WT
mice, as the mice mature, a small increase (2.8%) in the length of the AIS is seen (P = 0.008) and AISs move closer to the soma (by 22.6%, P < 0.015) and are thinner at their distal ends
(by 11.9%, P = 0.0049) but with no changes to the width of the proximal AIS. In the adult G127X mice the AISs also showed a trend to become thinner distally (2.1%, P = 0.0904) and moved
significantly closer to the soma, in fact, this was much more pronounced (37.1% closer, P < 0.0001). This most likely explained why AISs become wider proximally (by 17.9%, P < 0.0001)
as they come closer to the axon hillock which is a wider structure. The main difference, however, was that, unlike in WT mice, the AISs in the G127X mice actually became shorter with age (by
7.6%, P = 0.0004) instead of longer. Therefore, this decrease in AIS length in the 190 day old G127X mice is in contrast to the normal changes seen during development. ELECTROPHYSIOLOGICAL
RESULTS To determine whether the changes in AIS parameters observed in the adult mice can have a functional consequence, we investigated excitability parameters related to sodium channels
and action potential generation in the adult WT and G127X mice using _in vivo_ intracellular recording with sharp microelectrodes. Intracellular recording techniques _in vivo_ are generally
biased towards recording from alpha motoneurones rather than gamma due to their bigger cell size. We therefore assume our recordings to represent alpha motoneurones. All motoneurones used
for analysis were antidromically identified by stimulation of the sciatic nerve. ACTION POTENTIAL HEIGHT AND RATE OF RISE IS NOT DIFFERENT IN THE G127X MICE Antidromic action potentials were
recorded from 89 motoneurones in WT mice and 71 in G127X mice, examples of which are shown in Fig. 5A. The spike height of the somato-dendritic (SD) spike was measured from baseline to
peak, however, the amplitude of the initial segment (IS) action potential cannot be measured directly as it is masked by the SD action potential. The average of the action potential was
therefore differentiated to obtain the maximum rate of rise of the IS component (Fig. 5B, lower trace). Linear regression confirmed that the amplitude of the SD action potential correlated
with baseline membrane potential, Vm (WT: R2 = 0.7788, P < 0.0001; G127X: R2 = 0.7014, P < 0.0001, Fig. 5C). No significant differences were found with respect to both the slopes (P =
0.9186) and intercepts (P = 0.2156) of the regression lines between WT and G127X mice. Linear regression also confirmed that the maximum rate of rise of the IS component of the antidromic
action potential correlated with Vm (WT: R2 = 0.4488, P < 0.0001; G127X: R2 = 0.4221, both P < 0.0001, Fig. 5D). Again, no significant differences were found between the slopes (P =
0.6294) and intercepts (and P = 0.6381) of the 2 regression lines for action potentials from WT and G127X mice. Finally, the IS-SD latency was calculated as the latency between the maximum
rate of rise of the IS and SD components of the antidromic action potential (Fig. 5B). The mean IS-SD latency for WT was 0.29 ms (SD 0.105) and for G127X it was 0.2812 ms (SD 0.131) which
was not significantly different between WT and G127X mice (P = 0.3959 Mann Whitney U = 3332, Fig. 5E). RECOVERY FROM INACTIVATION IS REDUCED AT THE SOMA BUT NOT AISS OF G127X MICE To test
how quickly the antidromic spike could recover from inactivation, a second stimulation was given in close succession and the interval between the two action potentials decreased until the
second action potential failed (Fig. 6A). The last measurement of both spikes, just before the second spike fails, is referred to as the minimum inter-spike interval for the two SD action
potentials (ISImin SD-SD), measured from peak to peak of the two action potentials. Often, when the SD component of the second spike failed, the IS spike persisted (Fig. 6A). The point just
before the second IS action potential fails is referred to as ISImin SD-IS. Recovery from inactivation was tested in a total of 103 motoneurones in WT and 76 motoneurones in G127X mice. The
ISImin SD-SD was significantly longer in G127X mice (means (+SD) WT: 1.724 ms (0.838), G127X. 1.876 ms (0.7466), Mann Whitney U = 3224, P = 0.0438, Fig. 6B), suggesting that action
potentials at the soma have a longer recovery time from inactivation. The ISImin SD-IS, however, was not significantly different between WT and G127X mice (means (+SD) WT: 1.267 ms (0.333),
G127X. 1.342 ms (0.4434), Mann Whitney U = 3689, P = 0.5119, Fig. 6C), suggesting that at the AIS, the recovery from inactivation is unchanged in G127X mice. MOTONEURONES IN THE G127X MOUSE
RETAIN THE ABILITY TO FIRE REPETITIVELY IN RESPONSE TO INTRACELLULAR CURRENT INJECTION To test the ability of the motoneurones to fire repetitively in response to central inputs,
intracellular current injection was used to mimic synaptic input. Triangular ramps of current were injected through the microelectrode and the cell’s ability to fire repetitively determined.
Examples from both WT and G127X mice are shown in Fig. 7A. Before the cell was tested, it was first confirmed that electrode capacitance was correctly compensated (using the capacitance
compensation on the Axoclamp 2b amplifier) and that the electrode was passing the current, which is important given the high resistance electrodes used for these studies. The membrane
potential immediately prior to current injection was confirmed to be more hyperpolarised than −50 mV as, in our experience, more depolarized membrane potentials rarely exhibit repetitive
firing. Triangular current ramps evoked repetitive firing in 94.6% (53/56) motoneurones in WT mice and 94.7% (71/75) motoneurones in G127X mice (Fig. 7B). It is worth noting that the
motoneurones failing to fire repetitively had membrane potentials relatively close to −50 mV. Therefore, there was no significant impairment in repetitive firing in response to intracellular
current injection in G127X mice compared to WT. In 90 motoneurones (28 WT and 62 G127X) the current injection was taken to the level where repetitive firing of full spikes failed, in order
to determine the maximum firing frequencies in response to the ramp current injection (Fig. 7C). This was not significantly different between the two groups (P = 0.7550, t-test t = 0.313,
mean (+SD): WT 242.3 Hz (94.17), G127X 236.3 Hz (76.37), Fig. 7D). Consistent with our previous study31, an inflection in the current-frequency slopes corresponding to the onset of the
secondary range36, due to the onset of calcium PICs, was frequently observed, (Fig. 7C). When observed, this occurred at significantly lower firing frequencies in the G127X mice (P =
<0.0001, t-test t = 5.694, means (+SD), WT: 170 Hz (28.9), 29 cells, G127X: 129 Hz (22.2), 24 cells, Fig. 7E). In the majority of the cells in which repetitive firing was tested (49 WT
and 57 G127X), short hyperpolarizing current pulses (also in DCC mode) were used to estimate the input conductance to test if this may compensate for the increased PICs. This was found to be
significantly higher in G127X motoneurones (P = 0.0097, Mann-Whitney, U = 990, means (+SD), WT: 0.427 µS (0.222), G127X: 0.537 µS (0.2872), Fig. 7F). DISCUSSION Subtle differences were
found with respect to the geometric AIS parameters in the presymptomatic G127X SOD1 mouse model of ALS. Despite these changes, no significant differences were observed with respect to
features of individual action potentials between the two groups except a slight reduction in the recovery time for 2 successive somatic action potentials at very high firing frequencies.
None of the observed changes impaired the ability of the G127X motoneurones to fire repetitively or limit their maximum firing frequencies. PLASTICITY OF THE AIS The AISs of alpha
motoneurones in adult G127X mice were both significantly shorter and wider than in WT mice. Our measurements in ~22 day old mice confirmed that this was a plastic change in adulthood not
observed in WT mice. Despite shorter AISs, no functional changes were observed with respect to the rate of rise of the AIS action potential, suggesting no overall change in the number of Na+
channels. Using the mean distal AIS width to calculate the AIS diameter together with the mean AIS length we can calculate the mean surface area of the AIS for the 2 groups. These are
remarkably similar (WT: 96.99 μm2 and G127X 97.57 μm2). If we are to assume that the overall density of ion channels does not change (which is technically challenging to check with a highly
fixation-dependent antibody), then this would mean that there is a similar surface area of sodium channels in both groups which could explain why no differences were observed with respect to
the rate of rise of the AIS action potentials between the two groups. We have previously observed from a larger sample of rat motoneurones that AIS width correlates with soma size, with
larger motoneurones having wider AISs37. Furthermore, wider AISs were usually shorter and located more proximal to the somas. The changes in AIS parameters that we have observed in the
current experiments could therefore be explained by a compensatory response to the increase in soma size in the G127X mice. One concern is that the unequal distribution of males and females
in each group may have influenced the results, however, we have previously found in another study that the AIS parameters of mice motoneurones are not significantly different between adult
male and female C57BL mice37, although differences in cell size could be potentially explained in this way. In another, different SOD1 model, the G93A mouse, an increase in cell size has
also been observed of a similar magnitude (~8%)38 to what we observed in the G127X mouse (~12%). Gamma motoneurones also showed a reduction in the length of the AIS but with a reduction in
AIS width and no changes in the distance from the soma suggesting a decreased excitability of these neurones. Being much smaller than alpha motoneurones, gamma motoneurones possess a gamma
bias, producing a constant drive to the extrafusal muscle fibres. A decrease in gamma motoneurone excitability would therefore be expected to reduce the sensitivity of the muscle spindles to
changes in muscle length. Muscle stretch usually results in high frequency trains of action potentials in Ia afferents causing Excitatory Post Synaptic Potentials (EPSPs) in spinal
motoneurones which are normally reduced in amplitude at these high frequencies due to post activation depression. We have previously shown that post activation depression of the Ia EPSP is
reduced in the G127X mouse at a similar presymptomatic age to the current study39. Such a reduction in depression equates to a subtle increase in the amplitude of Ia EPSPs which may
compensate for reduction in the sensitivity of the muscle spindles potentially due to reduced gamma motoneurone excitability. Reductions in post activation depression is commonly observed in
disorders characterised by the feature of spasticity, it is therefore of interest to explore if the excitability of gamma motoneurones increases at symptom onset in this same model. CHANGES
IN AIS PARAMETERS OF ALPHA MOTONEURONES ARE CONSISTENT WITH CHANGES IN PROXIMAL MOTOR AXONS IN POST-MORTEM ALS PATIENTS Although the AISs of motoneurones of human ALS patients have not been
measured using precise molecular markers of the AIS, electron microscopy (EM) has been used to examine the proximal axons of ventral horn neurones from post-mortem patients with ALS. These
have shown a swelling of distal AISs (by around 21% in normal looking axons and 117% in swollen axons) and a decrease in the combined length of the AIS and axon hillock (measured as the
initial portion of the axon hillock to the point at which myelination started) by 4% in normal looking axons and 7.3% in swollen looking axons35. Our results confirm that similar changes
also occur in this animal model of the disease. Furthermore, the fact that we have observed this in pre-symptomatic mice suggests that this is an early event in the disease process. The EM
studies with the human tissue show the proximal axons to contain abnormal accumulations of neurofilaments, lysosomes, vesicles and mitochondria35,40 suggesting that deficits in axon
transport may underlie the swelling of the AISs. Similar accumulations of neurofilaments and mitochondria have also been observed in the proximal axons of motoneurones in the G93A SOD1 mouse
model41 suggesting that this could equivalently explain the changes in AIS diameter in adulthood in the G127X SOD1 model. REPETITIVE FIRING IS NOT IMPAIRED IN THE G127X MOUSE Our
electrophysiological recordings confirmed that the changes in AIS parameters did not impair the neurones ability to fire repetitive action potentials. This is in contrast to data recently
published in adult G93A SOD1 mice33 where a larger number of cells were unable to fire repetitively compared with WT. There are a number of possible reasons for this difference. One major
difference between the two models is in the timing of symptom onset and disease progression. In the G93A mouse strain, mice start to develop paralysis by 90 days of age followed by a disease
course lasting approximately 30 days33. The G127X mouse, by contrast, does not develop clear symptoms until approximately 250 days of age, followed by a rapid disease progression of around
10 days27. It is therefore possible that the adult pre-symptomatic time points used in the two different studies (G93A: 38 to 82 days and G127X: 213 days) represent different time points in
the disease progression. As cells are beginning to degenerate much earlier in the G93A mice, it is possible that in the experiments of Delestrée _et al_. the increase in non-firing cells may
represent penetrations of sick or dying cells. It is also worth noting, however, that in the current experiments there is a much lower proportion of cells lacking repetitive firing
abilities in WT mice than reported by Delestrée _et al_. Our criteria for classification were subtly different, potentially resulting in a higher number of cells classified as firing
repetitively. From our own experience, we know that even in WT mice, cells sometimes fail to fire repetitively in response to current injection initially after being penetrated by the
microelectrode, despite still displaying single action potentials. Most often, however, if tested later on in the recording they will display repetitive firing. Therefore, if a cell did not
fire initially it was tested again later on in the experiment. When testing in this way and by confirming the resting membrane to be more hyperpolarized than −50 mV (using extracellular
control on exiting the cells) almost all cells were found to be able to fire repetitively. Another possible explanation for the discrepancies between studies could be due to the use of
different anaesthetics. Both for the current and previous studies in the G127X mice we used Hypnorm and Midazolam anaesthesia. In contrast, in the experiments of Delestrée _et al_. a
barbiturate anaesthesia was used, which is known to block PICs42,43. In our previous study31 we have shown that activation of PICs is enhanced in the G127X mouse, a finding replicated in the
current experiments. This is important as increased PICs have also been observed in neonatal and embryonic motoneurones from the G93A mouse suggesting they may be a common feature of SOD1
ALS models. Therefore, it is plausible to suggest that the increase in persistent inward currents may be important for repetitive firing in SOD1 mice. The main channels mediating the Ca2+
PICs in motoneurones are believed to be located mainly on proximal dendrites44,45,46,47 where they would be activated by subthreshold excitatory input before the threshold for action
potential initiation is reached at the AIS. By contrast, when using intracellular current injected directly to the soma to mimic synaptic input (as done in intracellular recording
experiments), the current activates the AIS much earlier than the dendrites resulting in a delay in the activation of the dendritic Ca2+ channels mediating the PICs. This is then seen as the
secondary range on the input-output (I-F) slope48. Thus, during normal physiological activation of motoneurones, the PIC activation occurs at much lower firing frequencies. For example,
during intracellular current ramp injections, the simultaneous activation of afferent input to motoneurones causes activation of the secondary range at significantly lower firings
frequencies than without49. In fact, when activated by synaptic input alone the PIC would actually be activated before repetitive firing starts and therefore the amplification of the input
by PICs that underlies the secondary range would occur much earlier and therefore contribute to a steeper I-f slope in the primary range. This means that any increase in Ca2+ PIC would have
very real consequences for repetitive firing. The exact location of the channels mediating the Na+ PIC is unknown but they are likely to be located at the axon initial segment as this is the
highest density of channels that can mediate PICs. These are also activated at sub-spike threshold and are important for kicking off repetitive firing in motoneurones49,50,51. Therefore,
both Na+ and Ca2+ PICs would have very real implications for repetitive firing in motoneurones. Given that there appears to be increases in both the Ca2+ and Na+ PIC in adult SOD1 mice, then
repetitive firing would likely be more affected by anaesthesia in these animals. Our observation of an increase in input conductance is consistent with previous findings in the G93A SOD 1
mouse and the hypothesis that the increased PIC may be a mechanism to compensate for the increase in input conductance33. FUNCTIONAL CONSEQUENCES OF SLOWER MAXIMAL DOUBLET FIRING The only
change with respect to multiple action potential firing was in the recovery time for action potentials initiated at the soma. The mean minimal inter-spike-interval of the 2 successive
antidromic action potentials corresponded to 580 Hz in WT mice compared to 533 Hz in the G127X mice. When considering the functional implication of such a change, it is important to note
that these firing frequencies are well beyond the general firing rates reported for hind limb motoneurones in awake freely moving C57BL/6 J mice52. During rhythmic activity, such as
respiration and locomotion, however, motoneurones often initiate their active phase with high frequency doublets53,54. Mice hind limb motoneurones are no exception and often display
doublets, or even triplets at the onset of their active phases during fictive locomotion55. Doublets lead to a rapid potentiation of force due to non –linear summation of the force from the
two successive twitches which function to accelerate the force development at the start of movements, particularly when larger forces are required (reviewed by56). During fictive locomotion
in mice we have observed inter-spike-intervals of between 2.6 and 11 ms, corresponding to 90–384 Hz55, therefore the differences in the minimum inter-spike-intervals that we observed in this
study may still be considered to be outside of the normal working range during locomotion, assuming the ranges for doublets are similar in awake freely moving mice. CONCLUSION Despite
shorter AISs there was no evidence for reduced excitability within the functional working range of firing frequencies. Therefore, the conclusion from the current studies, along with our
previous publications is that there is no evidence for impairments in repetitive firing in this particular ALS strain at adult presymptomatic time points. Deficits in repetitive firing
should therefore not be considered a general feature of motoneurones in adult presymptomatic ALS models. It is, of course, entirely possible that this may change with disease progression in
this model, therefore it will be crucial to also investigate this in the same mutant after symptom onset. MATERIALS AND METHODS ETHICAL APPROVAL The experimental procedures were approved by
the Danish Animal Experiments Inspectorate (Permission no. 2010/561-1825 and 2013-15-2934-00879) with local approval from the Department of Experimental Medicine at Copenhagen University.
All procedures were in accordance with the EU Directive 2010/63/EU for the protection of animals used for scientific purposes. MICE Mice transgenically expressing G127insTGGG (G127X) mutant
human SOD1(Jonsson _et al_. 2004) were backcrossed on C57BL/6 J mice for more than 25 generations in Umea, Sweden57. Homozygote mice from the original line 716, over-expressing 19 copies of
the human SOD1 G127X gene, were then bred as homozygotes at our own institution. This model was used, as the mutant SOD1 itself lacks enzyme activity and is rapidly degraded resulting in low
levels of the protein in the spinal cord57. This reduces the risk of over-expression artefacts associated with more commonly used mutants such as the G93A SOD1 model. The G127X strain has a
relatively late clinical onset of around 250 days which is followed by a rapid progression of approximately one week. Experiments were therefore performed at ~190 days, a time point well
into adulthood but prior to obvious symptom onset. Any mice starting to show symptoms were excluded. Given that the mutant SOD1 G127X has no enzymatic function and that it was necessary to
breed the G127X mice as homozygotes, the best available controls were aged-matched C57BL/6 J mice, which we will refer to as wild type (WT). The numbers of mice used for each investigation
are detailed in Table 1. Due to the slow breeding of our colony of the G127X, mice of both genders were used. Our previous controls have shown no effect of sex on AIS parameters in adult
C57BL/6 J mice37. To investigate whether differences in AIS parameter represent a strain difference existing from birth or changes that occur during adulthood in the G127X mice, we also
labelled AISs in an additional 14 mice at ~22 (20–23) days of age (7 WT and 7 G127X). ELECTROPHYSIOLOGY EXPERIMENTS In vivo electrophysiological experiments were performed on 11 G127X mice
(~195 days old) and 6 age-matched C57BL/6 J mice. Experiments were performed as previously described31,58. Anaesthesia was induced and maintained with an intraperitoneal injection of a
mixture of Hypnorm (0.315 mg/mL fentanyl-citrate and 10 mg/mL fluanisone), Midazolam (5 mg/mL), and sterile water (mixed 1:1:2) with an induction dose of this mixture of 0.15 mL/25 g body
weight. Maintenance dosages of the same mixture were administered through an intraperitoneal cannula (0.05 mL every 20 minutes). All mice received a single dose of atropine (0.02 mg I.P.) at
the start of the surgery. A tracheal cannula was then inserted to allow for artificial ventilation. The sciatic nerve was dissected and a hemi-laminectomy performed at vertebral levels
T12-L1 allowing access to spinal levels L3-L4. Mice were then placed in a modified Narashige frame with the head secured in a head holder. The temperature was monitored using a rectal probe
and maintained at 37 °C using a heat pad underneath and a heat lamp above the mouse, controlled by the output from the temperature probe. Mice were connected to a ventilator (SAR-83 CWE) and
artificially ventilated at 70 breaths per minute (and a tidal volume of approximately 0.2 ml). Mice were then paralysed using the neuromuscular blocking agent Pavulon (diluted 1:10 with
saline then 0.1 ml dose initially followed by 0.05 ml doses every hour). Expired carbon dioxide levels were measured with a Capstar CO2 analyser (IITC Life Science) and clips placed on the
ear and rear foot measured the electrocardiogram (ECG) which was monitored throughout the experiment. This was used to monitor adequacy of the anaesthesia under neuromuscular blockage which
was maintained by administration of the same anaesthetic (dosage as necessary for the surgical procedures). Vertebral clamps were attached on the vertebrae above and below the laminectomy to
secure the spinal column in this region. A silver ball electrode was placed on the dorso-lateral surface of the spinal cord (and another down the side of the rib cage) to record the cord
dorsum potentials for timing of the arrival of signals at the spinal cord (for later classification of antidromic responses). For intracellular recording, using an electronic micro-drive, a
glass microelectrode (filled with 2 M Potassium acetate, resistances ~27 mΩ) was inserted into the spinal cord and antidromic field potentials from stimulation of the peripheral nerves were
used as guides to locate the motoneurones. Recordings were amplified and filtered (10 kHz) using custom-made amplifiers (Copenhagen University). Finally, the intracellular signals were
digitised (40 kHz) using the 1401 analogue to digital converter (Cambridge Electronic Design, UK) and recorded using the Signal software (Cambridge Electronic Design, UK). Identification of
motoneurones was made by the presence of all-or-none antidromic action potentials following stimulation of the sciatic nerve. Antidromic spikes were distinguished from synaptically driven
action potentials by latency (relative to the cord dorsum potential) and arriving before any synaptic activity evoked by the stimulation was observed in the neurone. Measurements of
antidromic action potentials were made from averages of successive trials (~10, averaged using Spike 2 software). Membrane potentials were measured at the time point at which averages were
made and these were confirmed using extracellular potentials on exit of the cell. Analysis of spike height was performed with respect to membrane potential. Antidromic action potentials had
the characteristic shape as previous defined59, consisting of an initial inflection in the depolarising phase caused by the presence of the initial segment (IS) spike arriving before the
full somato-dendritic (SD) action potential. Averages were exported to Excel (Microsoft, US) where the first derivative was obtained. From this maximum rate of rise could be obtained for
both the IS and SD components and the difference between the timing of these two points taken as the IS-SD latency59. To test the recovery from inactivation, two stimuli were given in close
succession and the timing between the two gradually decreased until the second antidromic action potential failed. The minimum interspike interval was then recorded. Finally, to test the
ability of the motoneurones to fire repetitively to central inputs, a ramp of increasing current was injected directly into the cell body through the microelectrode (at speeds of 5
nA/second, using DCC mode, 3 kHz on the Axoclamp amplifier) to evoke repetitive firing. Occasionally, motoneurones can fail to fire repetitively if tested immediately after penetration with
the glass microelectrode. In those cases the motoneurone was tested again later in the recording. Not all features were tested in all cells in all mice and so the number of cells and mice
tested are provided in the results section. ANATOMICAL EXPERIMENTS At the end of the electrophysiological experiments mice were perfused with saline via the left ventricle, followed by a
brief perfusion with 2% paraformaldehyde (in phosphate buffer, pH7.4). The spinal cords were then removed and post fixed for 1 hour in 2% paraformaldehyde. The lumbar enlargement was then
cut into 50 µm thick horizontal sections on a freezing microtome and ventral sections containing motoneurones processed. Axon initial segments were labelled with a primary antibody against
the voltage-gated sodium channel Nav 1.6 (Alomone 1:500) and a donkey anti-rabbit secondary antibody conjugated to Alexa Fluor 594 (Millipore 1:1000). We used Nav 1.6 as this is the main
sodium channel found at the AIS responsible for action potential initiation. The Nav 1.6 antibody labelled axon initial segments (confirmed by co-labelling with Ankyrin G or Pan Nav channel
antibodies) and nodes of Ranvier (confirmed by labelling of Caspr in the flanking paranodes) and pre-incubation of the antibody with its immunising peptide eliminated labelling (data not
shown). Secondary-only, auto-fluorescence and cross reactivity controls produced no labelling (data not shown). Motoneurones were labelled with an antibody directed against Choline
acetyletransferase (ChAT, Millipore, 1:100) and a donkey anti-goat secondary antibody conjugated to Alexa Fluor 488 (Millipore 1:1000). This labelled large ventral horn neurones
(motoneurones), sympathetic preganglionic neurones in the intermediolateral column and small neurones around the central canal and intercalated nucleus, all regions known to contain neurones
using acetylcholine as a neurotransmitter. By taking only horizontal sections through the ventral horn ChAT immunoreactive motoneurones could be isolated. AIS MEASUREMENTS Confocal images
of the lumbar spinal cord segments were obtained using a Zeiss LSM700 Axio Imager 2 microscope. Cells imaged were from regions of the lumbar enlargement corresponding to spinal levels L3-4
(regions containing hind limb motoneurones with axons in the sciatic nerve). Measurements of the AISs and the associated motoneurones were performed in 3 dimensions using confocal software
ZEN Black (Zeiss, Germany). The following parameters of the AIS were measured in 3D: length of the AIS, distal and proximal width, distance of the proximal AIS from the cell body. Not all
measurements were possible for each AIS, for example, only the AISs where both the distal and proximal ends were clearly contained within the Z-stacks were measured for length. Surface area
of the neuronal cell body was measured in the x-y plane and was calculated as the area of an ellipse obtained from the minimum and maximum diameters. Identification of gamma motoneurones was
based on size criterion (gamma motoneurones being less than 485 µm260) and lack of large cholinergic boutons surrounding the gamma motoneurone soma. Due to the large size of the
motoneurones and the thickness of the sections being only 50 µm, many of the motoneurone cell bodies were not entirely contained within the Z-stack, however their AISs were still included in
the study if they could be identified as originating from a motoneurone (hence some gamma motoneurones may still be included in the larger sample). An extra two WT mice (not included in the
table) underwent immunohistochemical labelling for Nav.1.6 but the labelling did not adequately label AISs (presumably due to too high a level of tissue fixation and the Nav1.6 antibody
being very sensitive to fixation) so these mice were excluded. CELL COUNTING A further 9 mice (4 WT and 5G127X) were perfused with 4% paraformaldehyde (in phosphate buffer pH7.4) and
post-fixed in the same fixative for approximately 2 hours. The lower thoracic, lumbar enlargement and upper sacral part of the spinal cord was cut into transverse 40 µm thick sections and
stored in serial order. These sections were processed immunohistochemically for ChAT (same primary antibody as above) using a donkey anti-goat secondary antibody conjugated to Alexa Fluor
594. For cell counting only every 10th section was used. Counting of motoneurones was carried out using an epifluorescent microscope (Axioplan 2, Zeiss, Germany). Only motoneurones with
visible nuclei in their somas were counted. The rostral-caudal extent of the lumbar region of the spinal cord used for counting was defined by the end of the intermediolateral (IML) nucleus
(rostral border) and the sacral parasympathetic (SPSy) nucleus (caudal border), both of which contain ChAT-immunoreactive neurones and mark the first lumbar segment and the beginning of the
sacral segments, respectively. The results were plotted in serial order and aligned with respect to the last section containing IML neurones, the first section containing SPSy neurones and
the central peak. Illustrative photographs were captured with a laser scanning confocal microscope (Leica LSM 700). Brightness and contrast for all illustrations was adjusted using Image J
(NIH) or Photoshop (Adobe) and was applied uniformly to the entire image for illustration purposes only. All analyses, however, were performed with the raw data. STATISTICAL ANALYSES All
statistical analyses were performed using the GraphPad Prism software. D’Agostino & Pearson omnibus normality tests were used to confirm normality. For data passing this, parametric
statistics (t-tests) were used. For data not passing normality tests (or analyses with a low sample number per group) non-parametric statistics (Mann-Whitney) were used. Statistical
significance was accepted at the P < 0.05 level. On all graphs asterisks are used to indicate the following levels of significance: *(P < 0.05), **(P < 0.01), ***(P < 0.001).
Unless indicated on the graphs no significant difference was found. DATA AVAILABILITY The data that support the findings of this study are available on request from the corresponding author.
REFERENCES * Bensimon, G., Lacomblez, L. & Meininger, V. A controlled trial of riluzole in amyotrophic lateral sclerosis. ALS/Riluzole Study Group. _N. Engl. J. Med._ 330, 585–591
(1994). Article CAS PubMed Google Scholar * Lacomblez, L., Bensimon, G., Leigh, P. N., Guillet, P. & Meininger, V. Dose-ranging study of riluzole in amyotrophic lateral sclerosis.
Amyotrophic Lateral Sclerosis/Riluzole Study Group II. _Lancet_ 347, 1425–1431 (1996). Article CAS PubMed Google Scholar * Lacomblez, L. _et al_. A confirmatory dose-ranging study of
riluzole in ALS. ALS/Riluzole Study Group-II. _Neurology_ 47, S242–S250 (1996). Article CAS PubMed Google Scholar * Miller, R. G. _et al_. Clinical trials of riluzole in patients with
ALS. ALS/Riluzole Study Group-II. _Neurology_ 47, S86–S90 (1996). Article CAS PubMed Google Scholar * Miller, R. G., Mitchell, J. D., Lyon, M. & Moore, D. H. Riluzole for amyotrophic
lateral sclerosis (ALS)/motor neuron disease (MND). _Cochrane. Database. Syst. Rev_. CD001447 (2007). * Bostock, H., Sharief, M. K., Reid, G. & Murray, N. M. Axonal ion channel
dysfunction in amyotrophic lateral sclerosis. _Brain_ 118(Pt 1), 217–225 (1995). Article PubMed Google Scholar * Cheah, B. C. _et al_. Progressive axonal dysfunction and clinical
impairment in amyotrophic lateral sclerosis. _Clin. Neurophysiol._ 123, 2460–2467 (2012). Article CAS PubMed Google Scholar * Kanai, K. _et al_. Altered axonal excitability properties in
amyotrophic lateral sclerosis: impaired potassium channel function related to disease stage. _Brain_ 129, 953–962 (2006). Article PubMed Google Scholar * Nakata, M. _et al_. Distal
excitability changes in motor axons in amyotrophic lateral sclerosis. _Clin. Neurophysiol._ 117, 1444–1448 (2006). Article CAS PubMed Google Scholar * Shibuta, Y. _et al_. Utility of
recovery cycle with two conditioning pulses for detection of impaired axonal slow potassium current in ALS. _Clin. Neurophysiol._ 121, 2117–2120 (2010). Article PubMed Google Scholar *
Shibuya, K. _et al_. Split hand syndrome in amyotrophic lateral sclerosis: different excitability changes in the thenar and hypothenar motor axons. _J. Neurol. Neurosurg. Psychiatry_ 84,
969–972 (2013). Article PubMed Google Scholar * Stephanova, D. I., Krustev, S. M. & Negrev, N. Mechanisms defining the electrotonic potential abnormalities in simulated amyotrophic
lateral sclerosis. _J. Integr. Neurosci._ 11, 155–167 (2012). Article CAS PubMed Google Scholar * Vucic, S. & Kiernan, M. C. Axonal excitability properties in amyotrophic lateral
sclerosis. _Clin. Neurophysiol._ 117, 1458–1466 (2006). Article PubMed Google Scholar * Vucic, S. & Kiernan, M. C. Upregulation of persistent sodium conductances in familial ALS. _J.
Neurol. Neurosurg. Psychiatry_ 81, 222–227 (2010). Article PubMed Google Scholar * Iwai, Y. _et al_. Axonal Dysfunction Precedes Motor Neuronal Death in Amyotrophic Lateral Sclerosis.
_PLoS. One._ 11, e0158596 (2016). Article PubMed PubMed Central CAS Google Scholar * Devlin, A. C. _et al_. Human iPSC-derived motoneurons harbouring TARDBP or C9ORF72 ALS mutations are
dysfunctional despite maintaining viability. _Nat. Commun._ 6, 5999 (2015). Article ADS CAS PubMed Google Scholar * Naujock, M. _et al_. 4-Aminopyridine Induced Activity Rescues
Hypoexcitable Motor Neurons from Amyotrophic Lateral Sclerosis Patient-Derived Induced Pluripotent. _Stem Cells. Stem Cells_ 34, 1563–1575 (2016). Article CAS PubMed Google Scholar *
Sareen, D. _et al_. Targeting RNA foci in iPSC-derived motor neurons from ALS patients with a C9ORF72 repeat expansion. _Sci. Transl. Med._ 5, 208ra149 (2013). Article PubMed PubMed
Central CAS Google Scholar * Wainger, B. J. _et al_. Intrinsic membrane hyperexcitability of amyotrophic lateral sclerosis patient-derived motor neurons. _Cell Rep._ 7, 1–11 (2014).
Article CAS PubMed PubMed Central Google Scholar * Ho, R. _et al_. ALS disrupts spinal motor neuron maturation and aging pathways within gene co-expression networks. _Nat. Neurosci._
19, 1256–1267 (2016). Article CAS PubMed PubMed Central Google Scholar * Fritz, E. _et al_. Mutant SOD1-expressing astrocytes release toxic factors that trigger motoneuron death by
inducing hyperexcitability. _J. Neurophysiol._ 109, 2803–2814 (2013). Article CAS PubMed PubMed Central Google Scholar * Rojas, F., Cortes, N., Abarzua, S., Dyrda, A. & van Zundert,
B. Astrocytes expressing mutant SOD1 and TDP43 trigger motoneuron death that is mediated via sodium channels and nitroxidative stress. _Front Cell Neurosci_ 8, 24 (2014). Article PubMed
PubMed Central CAS Google Scholar * Kuo, J. J., Siddique, T., Fu, R. & Heckman, C. J. Increased persistent Na(+) current and its effect on excitability in motoneurones cultured from
mutant SOD1 mice. _J. Physiol_ 563, 843–854 (2005). Article CAS PubMed PubMed Central Google Scholar * Pieri, M., Carunchio, I., Curcio, L., Mercuri, N. B. & Zona, C. Increased
persistent sodium current determines cortical hyperexcitability in a genetic model of amyotrophic lateral sclerosis. _Exp. Neurol._ 215, 368–379 (2009). Article CAS PubMed Google Scholar
* Zona, C., Pieri, M. & Carunchio, I. Voltage-dependent sodium channels in spinal cord motor neurons display rapid recovery from fast inactivation in a mouse model of amyotrophic
lateral sclerosis. _J. Neurophysiol._ 96, 3314–3322 (2006). Article PubMed Google Scholar * Quinlan, K. A., Schuster, J. E., Fu, R., Siddique, T. & Heckman, C. J. Altered postnatal
maturation of electrical properties in spinal motoneurons in a mouse model of amyotrophic lateral sclerosis. _J. Physiol_ 589, 2245–2260 (2011). Article CAS PubMed PubMed Central Google
Scholar * Moldovan, M. _et al_. Nerve excitability changes related to axonal degeneration in amyotrophic lateral sclerosis: Insights from the transgenic SOD1(G127X) mouse model.
_Experimental Neurology_ 233, 408–420 (2012). Article CAS PubMed Google Scholar * Maglemose, R. _et al_. Potassium channel abnormalities are consistent with early axon degeneration of
motor axons in the G127X SOD1 mouse model of amyotrophic lateral sclerosis. _Exp. Neurol_ (2017). * Evans, M. D. _et al_. Calcineurin signaling mediates activity-dependent relocation of the
axon initial segment. _J. Neurosci._ 33, 6950–6963 (2013). Article CAS PubMed PubMed Central Google Scholar * Grubb, M. S. & Burrone, J. Activity-dependent relocation of the axon
initial segment fine-tunes neuronal excitability. _Nature_ 465, 1070–1074 (2010). Article ADS CAS PubMed PubMed Central Google Scholar * Meehan, C. F. _et al_. Intrinsic properties of
lumbar motor neurones in the adult G127insTGGG superoxide dismutase-1 mutant mouse in vivo: evidence for increased persistent inward currents. _Acta Physiol (Oxf)_ 200, 361–376 (2010).
Article CAS Google Scholar * Evans, M. D., Dumitrescu, A. S., Kruijssen, D. L., Taylor, S. E. & Grubb, M. S. Rapid Modulation of Axon Initial Segment Length Influences Repetitive
Spike Firing. _Cell Rep._ 13, 1233–1245 (2015). Article CAS PubMed PubMed Central Google Scholar * Delestree, N. _et al_. Adult spinal motoneurones are not hyperexcitable in a mouse
model of inherited amyotrophic lateral sclerosis. _J. Physiol_ 592, 1687–1703 (2014). Article CAS PubMed PubMed Central Google Scholar * Sasaki, S., Maruyama, S., Yamane, K., Sakuma, H.
& Takeishi, M. Swellings of proximal axons in a case of motor neuron disease. _Ann. Neurol._ 25, 520–522 (1989). Article CAS PubMed Google Scholar * Sasaki, S. & Maruyama, S.
Increase in diameter of the axonal initial segment is an early change in amyotrophic lateral sclerosis. _J. Neurol. Sci._ 110, 114–120 (1992). Article CAS PubMed Google Scholar *
Kernell, D. Synaptic influence on the repetitive activity eleicted in cat lumbosacral motoneurones by long-lasting injected currents. _Acta Physiol Scand_ 63, 409–410 (1965). Article CAS
PubMed Google Scholar * Jørgensen, H. S., Lehnhoff, J., Jakobsen, M. H., Jensen, D. B. & Meehan, C. F. Axon initial segment geometry in normal mouse spinal motoneurones and in the
symptomatic G127X mouse model of amyotrophic lateral sclerosis. Program No 423.24. 2015 Neuroscience Meeting Planner. _Chicago. Society for Neuroscience_ 2016. * Shoenfeld, L. _et al_. Soma
size and Cav1.3 channel expression in vulnerable and resistant motoneuron populations of the SOD1G93A mouse model of ALS. _Physiol Rep_. 2 (2014). Article PubMed PubMed Central CAS
Google Scholar * Hedegaard, A. _et al_. Postactivation depression of the Ia EPSP in motoneurons is reduced in both the G127X SOD1 model of amyotrophic lateral sclerosis and in aged mice.
_J. Neurophysiol._ 114, 1196–1210 (2015). Article CAS PubMed PubMed Central Google Scholar * Sasaki, S., Maruyama, S., Yamane, K., Sakuma, H. & Takeishi, M. Ultrastructure of
swollen proximal axons of anterior horn neurons in motor neuron disease. _J. Neurol. Sci._ 97, 233–240 (1990). Article CAS PubMed Google Scholar * Sasaki, S., Warita, H., Abe, K. &
Iwata, M. Impairment of axonal transport in the axon hillock and the initial segment of anterior horn neurons in transgenic mice with a G93A mutant SOD1 gene. _Acta Neuropathol._ 110, 48–56
(2005). Article CAS PubMed Google Scholar * Button, D. C., Gardiner, K., Marqueste, T. & Gardiner, P. F. Frequency-current relationships of rat hindlimb alpha-motoneurones. _J.
Physiol_ 573, 663–677 (2006). Article CAS PubMed PubMed Central Google Scholar * Guertin, P. A. & Hounsgaard, J. Non-volatile general anaesthetics reduce spinal activity by
suppressing plateau potentials. _Neuroscience_ 88, 353–358 (1999). Article CAS PubMed Google Scholar * Bui, T. V., Ter-Mikaelian, M., Bedrossian, D. & Rose, P. K. Computational
estimation of the distribution of L-type Ca(2+) channels in motoneurons based on variable threshold of activation of persistent inward currents. _J. Neurophysiol._ 95, 225–241 (2006).
Article CAS PubMed Google Scholar * Carlin, K. P., Jones, K. E., Jiang, Z., Jordan, L. M. & Brownstone, R. M. Dendritic L-type calcium currents in mouse spinal motoneurons:
implications for bistability. _Eur. J. Neurosci._ 12, 1635–1646 (2000). Article CAS PubMed Google Scholar * Grande, G., Bui, T. V. & Rose, P. K. Estimates of the location of L-type
Ca2+ channels in motoneurons of different sizes: a computational study. _J. Neurophysiol._ 97, 4023–4035 (2007). Article PubMed Google Scholar * Westenbroek, R. E., Hoskins, L. &
Catterall, W. A. Localization of Ca2+ channel subtypes on rat spinal motor neurons, interneurons, and nerve terminals. _J. Neurosci._ 18, 6319–6330 (1998). Article CAS PubMed PubMed
Central Google Scholar * Schwindt, P. C. & Crill, W. E. Factors influencing motoneuron rhythmic firing: results from a voltage-clamp study. _J. Neurophysiol._ 48, 875–890 (1982).
Article CAS PubMed Google Scholar * Bennett, D. J., Hultborn, H., Fedirchuk, B. & Gorassini, M. Synaptic activation of plateaus in hindlimb motoneurons of decerebrate cats. _J.
Neurophysiol._ 80, 2023–2037 (1998). Article CAS PubMed Google Scholar * Harvey, P. J., Li, Y., Li, X. & Bennett, D. J. Persistent sodium currents and repetitive firing in
motoneurons of the sacrocaudal spinal cord of adult rats. _J. Neurophysiol._ 96, 1141–1157 (2006). Article CAS PubMed Google Scholar * Kuo, J. J., Lee, R. H., Zhang, L. & Heckman, C.
J. Essential role of the persistent sodium current in spike initiation during slowly rising inputs in mouse spinal neurones. _J. Physiol_ 574, 819–834 (2006). Article CAS PubMed PubMed
Central Google Scholar * Hadzipasic,M. _et al_. Reduced high-frequency motor neuron firing, EMG fractionation, and gait variability in awake walking ALS mice. _Proc. Natl. Acad. Sci. U. S.
A_ 113, E7600-E7609 (2016). Article CAS Google Scholar * Gorassini, M., Eken, T., Bennett, D. J., Kiehn, O. & Hultborn, H. Activity of hindlimb motor units during locomotion in the
conscious rat. _J. Neurophysiol._ 83, 2002–2011 (2000). Article CAS PubMed Google Scholar * Kirkwood, P. A. & Munson, J. B. The incidence of initial doublets in the discharges of
motoneurones of two different inspiratory muscles in the cat. _J. Physiol_ 493(Pt 2), 577–587 (1996). Article CAS PubMed PubMed Central Google Scholar * Meehan, C. F., Mayr, K. A.,
Manuel, M., Nakanishi, S. T. & Whelan, P. J. Decerebrate mouse model for studies of the spinal cord circuits. _Nat. Protoc._ 12, 732–747 (2017). Article CAS PubMed PubMed Central
Google Scholar * Mrowczynski, W., Celichowski, J., Raikova, R. & Krutki, P. Physiological consequences of doublet discharges on motoneuronal firing and motor unit force. _Front Cell
Neurosci_ 9, 81 (2015). Article PubMed PubMed Central CAS Google Scholar * Jonsson, P. A. _et al_. Minute quantities of misfolded mutant superoxide dismutase-1 cause amyotrophic lateral
sclerosis. _Brain_ 127, 73–88 (2004). Article PubMed Google Scholar * Meehan, C. F., Sukiasyan, N., Zhang, M., Nielsen, J. B. & Hultborn, H. Intrinsic properties of mouse lumbar
motoneurons revealed by intracellular recording in vivo. _J. Neurophysiol._ 103, 2599–2610 (2010). Article CAS PubMed Google Scholar * Lipski, J. Antidromic activation of neurones as an
analytic tool in the study of the central nervous system. _J. Neurosci. Methods_ 4, 1–32 (1981). Article CAS PubMed Google Scholar * Shneider, N. A., Brown, M. N., Smith, C. A., Pickel,
J. & Alvarez, F. J. Gamma motor neurons express distinct genetic markers at birth and require muscle spindle-derived GDNF for postnatal survival. _Neural Dev._ 4, 42 (2009). Article
PubMed PubMed Central CAS Google Scholar Download references ACKNOWLEDGEMENTS This work was supported by a grant from the Lundbeck foundation. Karin Graffmo and Stefan Marklund, Umea
University, originally provided the G127X SOD1 mouse line. Thanks also to Dennis Bo Jensen for careful proof reading of the manuscript. All confocal analysis was performed at the Core
Facility for Integrated Microscopy (CFIM) at the University of Copenhagen, Faculty of Health. AUTHOR INFORMATION Author notes * These authors contributed equally: V. S. Bonnevie and K. P.
Dimintiyanova. AUTHORS AND AFFILIATIONS * Department of Neuroscience, University of Copenhagen, Panum Institute, Blegdamsvej 3, DK-2200, Copenhagen N, Denmark V. S. Bonnevie, K. P.
Dimintiyanova, A. Hedegaard, J. Lehnhoff, L. Grøndahl, M. Moldovan & C. F. Meehan Authors * V. S. Bonnevie View author publications You can also search for this author inPubMed Google
Scholar * K. P. Dimintiyanova View author publications You can also search for this author inPubMed Google Scholar * A. Hedegaard View author publications You can also search for this author
inPubMed Google Scholar * J. Lehnhoff View author publications You can also search for this author inPubMed Google Scholar * L. Grøndahl View author publications You can also search for
this author inPubMed Google Scholar * M. Moldovan View author publications You can also search for this author inPubMed Google Scholar * C. F. Meehan View author publications You can also
search for this author inPubMed Google Scholar CONTRIBUTIONS V.S.B.: Immunohistochemistry experiments and analysis of anatomical data, edited manuscript. K.P.D.: Immunohistochemistry
experiments and analysis of anatomical data. Co-wrote manuscript and prepared figures. A.H.: Analysis (electrophysiological data), edited manuscript. J.L.: Analysis (electrophysiological
data), edited manuscript. L.G.: Electrophysiological experiments. M.M.: Conception of experiments, provided the G127X SOD1 mice and edited manuscript. C.F.M.: Conception and design of
experiments, electrophysiological experiments and co-wrote manuscript. All authors approved the final version of the manuscript. CORRESPONDING AUTHOR Correspondence to C. F. Meehan. ETHICS
DECLARATIONS COMPETING INTERESTS The authors declare no competing interests. ADDITIONAL INFORMATION PUBLISHER’S NOTE Springer Nature remains neutral with regard to jurisdictional claims in
published maps and institutional affiliations. RIGHTS AND PERMISSIONS OPEN ACCESS This article is licensed under a Creative Commons Attribution 4.0 International License, which permits use,
sharing, adaptation, distribution and reproduction in any medium or format, as long as you give appropriate credit to the original author(s) and the source, provide a link to the Creative
Commons license, and indicate if changes were made. The images or other third party material in this article are included in the article’s Creative Commons license, unless indicated
otherwise in a credit line to the material. If material is not included in the article’s Creative Commons license and your intended use is not permitted by statutory regulation or exceeds
the permitted use, you will need to obtain permission directly from the copyright holder. To view a copy of this license, visit http://creativecommons.org/licenses/by/4.0/. Reprints and
permissions ABOUT THIS ARTICLE CITE THIS ARTICLE Bonnevie, V.S., Dimintiyanova, K.P., Hedegaard, A. _et al._ Shorter axon initial segments do not cause repetitive firing impairments in the
adult presymptomatic G127X SOD-1 Amyotrophic Lateral Sclerosis mouse. _Sci Rep_ 10, 1280 (2020). https://doi.org/10.1038/s41598-019-57314-w Download citation * Received: 29 July 2019 *
Accepted: 19 December 2019 * Published: 28 January 2020 * DOI: https://doi.org/10.1038/s41598-019-57314-w SHARE THIS ARTICLE Anyone you share the following link with will be able to read
this content: Get shareable link Sorry, a shareable link is not currently available for this article. Copy to clipboard Provided by the Springer Nature SharedIt content-sharing initiative