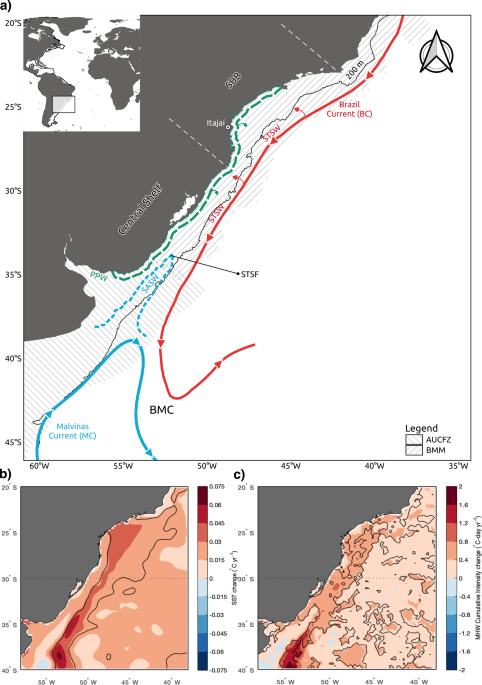
Tropicalization of demersal megafauna in the western south atlantic since 2013
- Select a language for the TTS:
- UK English Female
- UK English Male
- US English Female
- US English Male
- Australian Female
- Australian Male
- Language selected: (auto detect) - EN
Play all audios:

ABSTRACT Temperatures in the southwest South Atlantic Ocean have increased over recent decades due to changes in Brazil's Current dynamics affecting marine ecosystems. However, the
effects of ocean warming on demersal fauna in the region are poorly understood. Here, we analyse megafauna species composition, and changes in biomass of species with warm- and cold-water
affinities for 29,021 commercial demersal catches landed in the harbours of Santa Catarina state, Brazil, between 2000 and 2019. We find evidence of megafauna tropicalization in the
Brazilian Meridional Margin during this period. The mean temperature of catches has increased sharply since 2013, with the averaged optimal temperature preferences of all species in
commercial catches in one year increasing at a rate of 0.41 °C yr−1. These changes in the mean temperature of the catch correlate well with model-based bottom water temperatures (with 0 and
1-year time-lag) and transport volumes of the Brazil Current (with 3 and 4-year time-lag). SIMILAR CONTENT BEING VIEWED BY OTHERS CROSS-BASIN AND CROSS-TAXA PATTERNS OF MARINE COMMUNITY
TROPICALIZATION AND DEBOREALIZATION IN WARMING EUROPEAN SEAS Article Open access 08 March 2024 FUTURE TRENDS OF MARINE FISH BIOMASS DISTRIBUTIONS FROM THE NORTH SEA TO THE BARENTS SEA
Article Open access 05 July 2024 OCEAN ACIDIFICATION MAY SLOW THE PACE OF TROPICALIZATION OF TEMPERATE FISH COMMUNITIES Article 08 February 2021 INTRODUCTION Oceans have absorbed most of the
heat increase of the atmosphere since pre-industrial times gradually warming, on average, by 0.63 °C (1850–1900 period until 1986–20051). Direct observations have revealed important
geographic and depth variability in such change, as well as in related physical and biogeochemical transformations including sea level rise, increased frequency of storms, deceleration of
thermohaline circulation, expansion of areas with well-stratified water columns, a decrease of net primary productivity, and deoxygenation2,3,4,5. In addition, nearly 1/3 of CO2 added to the
atmosphere by anthropogenic activities has been absorbed in the ocean surface changing seawater chemistry towards a more acidic state, which decreases carbonate availability for the
development of numerous life forms6. Exposed to such environmental changes, marine species have shown alterations in abundance, phenology, and spatial distribution ranges (both bathymetric
and geographic), modifying community species composition (beta diversity), the structure of trophic chains and the metabolic and consumption rates of their trophic levels7,8,9,10,11,12,13.
Across different spatial scales, alterations in species diversity and functions are expected to disturb marine ecosystems functioning and the services they provide to society, including
fisheries14. Poleward expanding isotherms have favoured the invasion of tropical/subtropical species to suitable habitats in higher latitudes and the retraction of the equatorward limits of
temperate species distribution9,12. These processes have increased the diversity of pelagic and benthic fauna in subtropical regions by the addition of species with affinities for warmer
waters, eventually replacing those with an affinity for cooler waters that become progressively scarce15,16. In such regions, where multispecies fisheries have been historically developed,
reshuffling the diversity of fish and shellfish assemblages may have altered catch composition, gradually including higher and lower proportions of species with warm- and cold-water
affinities, respectively. Cheung et al.17 explored this concept by developing a metric defined as the ‘mean temperature of the catches’ (MTC), which involves averaging optimal temperature
preferences of all species included in commercial catches during one year, weighed by their annual catch. In their analysis, MTC annual variation between 1970 and 2006 evidenced ocean
warming signals in 52 large marine ecosystems and was shown to be related to increasing trends of regional sea surface temperatures. This global process was defined as a ‘tropicalization’ of
the catch, also characterized in different regional studies18,19,20,21,22. The Southwest South Atlantic Ocean (SWAO) extending from Cabo Frio (Brazil, 22°S) to Tierra del Fuego (Argentina,
55°S), comprises one of the world’s largest marine warming hotspots, as its water temperature has increased above the global average in recent years23,24. In this region, satellite-derived
sea surface temperatures have shown positive anomalies of 0.5–1.0 °C between 1950 and 1999, and of 0.5 °C between 2000–201623,25. These anomalies have been produced by a poleward
displacement of wind patterns over the South Atlantic leading to a southward expansion of the warm waters of the Brazil Current, which created, over the past decades, a warming region along
its path (Fig. 1)25,26,27. Ecosystem responses to this ocean warming process in the region have been poorly studied, but Franco et al.25 reviewed existing evidence of more frequent harmful
algal blooms, events of shellfish mass mortalities, and modifications in fisheries regimes. Particularly relevant was the study by Gianelli et al.21, which revealed an increasing trend of
MTC calculated for demersal catches at the Argentinian–Uruguayan Common Fishing Zone (AUCFZ, ~34°–40°S) between 1973 and 2017. Authors detected a decreasing representation of cold-water
species in the catches, a pattern significantly related to a sea surface temperature increase in the period. The Brazilian Meridional Margin (BMM- _sensu_ Alberoni et al.28) occupies the
northern sector of SWAO (~20°S–34°S) (Fig. 1). The region is influenced by the Brazil Current that flows southwards along the shelf break and upper slope carrying Tropical Waters, South
Atlantic Central Waters and, south of 28°S, deep Antarctic Intermediate Waters29. At ~38°S, the Brazil Current collides with subantarctic waters carried northwards by the Malvinas Current,
deflecting eastwards over the South Atlantic Ocean basin. This oceanographic front, known as Brazil–Malvinas Confluence (Fig. 1), has gradually displaced poleward over the past decades
(0.6–0.9° latitude per decade), as the Brazil Current expanded southwards, in association with the ocean temperature increasing trend in the region24,26,27. Along its path on the BMM, the
Brazil Current also influences shelf waters (a) by inducing shelf break upwellings of the South Atlantic Central Waters30,31 and (b) through lateral mixing of Tropical Waters with northward
flowing coastal waters, derived from the discharge plumes of the La Plata River and the Patos/Mirim Lagoon systems, forming Subtropical Shelf Waters32. At the southern extreme of the BMM,
this warm water mass is intersected by a wedge of cold Subantarctic Shelf Waters, derived from the Patagonian Continental Shelf, forming the Subtropical Shelf Front (Fig. 1), a shoreward
continuation of Brazil–Malvinas Confluence33. Along the SWAO, these ocean-shelf interactions suggest that global warming-induced changes in the Brazil Current dynamics, and the resulting
ocean warming process, may have extended to shelf waters altering species habitats and affecting fauna diversity21. Descriptions of demersal fauna geographic distribution patterns have long
characterized the BMM as a transition zone between subtropical and temperate faunas34,35,36 formed as a consequence of historical processes of diversification in the Western Atlantic37 and
the influence of seasonal latitudinal fluctuations of Brazil–Malvinas Confluence and the Subtropical Shelf Front38. These fronts affect the latitudinal and seasonal distribution of
subtropical and warm-temperate species and the extent to which they seasonally overlap in the BMM39. The availability of this heterogeneous fauna has driven the development, since the 1960s,
of large-scale multispecies demersal fisheries which economically thrived from the catch of assorted subtropical and warm-temperate teleosts, elasmobranchs, crustaceans and cephalopod
species38,39,40,41,42,43. These species have sustained annual catches oscillating around 88,000 t between 1986 and 2004, which represented over 35% of total catches in the region, on
average41. Based on the fact that the BMM is inserted within the Southwest South Atlantic Ocean marine warming hotspot area (Fig. 1), and the warming catch patterns revealed by Gianelli et
al.21 at the AUCFZ, we postulate that: (a) changes in the demersal community have taken place in the BMM during the past decades towards a tropicalization scenario, (b) these changes have
produced detectable signals in the species composition of multispecies demersal catches and (c) they can be mostly explained by the variation of ocean temperature and the dynamics of the
Brazil Current in the region. We addressed these premises by analysing the annual variation of demersal catch composition, monitored in southern Brazil between 2000 and 2019, and the effect
of sea bottom temperature and transport volumes of the Brazil Current on that variation. Because these large-scale environmental changes may have a gradual and heterogeneous effect on
megafauna species13, we included time-lags (1–4 years) to allow for delayed mechanistic links between ocean warming and biological responses. The study explored two distinct analytical
approaches; the analysis of annual variation of the MTC index17, and the analysis of species composition and beta diversity applied to species recorded in the catches of the demersal
fisheries44. In a previous analysis, using reconstructed regional catch data, Cheung et al.17 obtained an oscillating pattern of MTC in the region. Conversely, a study on clupeoid fish
populations in coastal areas of Rio de Janeiro State (23°S) provided robust evidence of tropical species replacing subtropical ones45. In the present study, we reveal signals of
tropicalization of the catches during the past decades and identified patterns of abundance gains and losses of species with affinities for warm- and cold waters, respectively, throughout
this process. RESULTS AND DISCUSSION Catches throughout the study period reached maximum levels in 2006–2012, decreasing sharply thereafter reaching low levels in 2019 (Supplementary Fig.
1). The whitemouth croaker (_Micropogonias furnieri_) and the argentine croaker (_Umbrina canosai_) were the dominant species in the catches. Jointly, they represented, on average, over 50%
of the total landed biomass in the period. This biomass included other 78 species: 62 teleosts, 3 elasmobranchs, 8 crustaceans and 5 molluscs. Overall, catch composition maintained a 1.5:1
ratio of species with warm- and cold-water affinities from the beginning of the time series until 2012. After that, warm-water species abundance increased in the catches changing the
resulting ratio to 4.1:1 in 2019 (Fig. 2). MEAN TEMPERATURE OF THE CATCHES Annual MTC oscillated around 21 °C (SD = 0.63 °C) between 2000 and 2019. Until 2013, the MTC time-series exhibited
peaks (2005, 2010) and troughs (2008, 2013), but no particular trend was evidenced. After 2013, MTC increased continuously reaching maximum values in 2019 (Fig. 3). The segmented regression
model defined one significant discontinuity in 2012 (95% CI: 2010–2015), which delimited an early period (2000–2012) when MTC oscillated with no significant trend (_p_-value = 0.789), from a
late period (2013–2019) when MTC increased sharply at a 0.41 °C yr−1 (_p_-value < 0.001) (Table 1). Similar catch warming trends have been described in Large Marine Ecosystems around the
globe17 and in more limited regions including the Aegean and Ionian Seas18,19, the Yellow and East China Seas20 and the Bay of Biscay (Cantabrian Sea—NE Atlantic)46. Considering the entire
time-series, the MTC increase rate in the BMM was equal to 0.57 °C. decade−1, exceeding estimates for the world ocean (0.19 °C. decade−1) and for non-tropical regions (0.23 °C decade−1)17,
as well as for the regions above, except the Aegean Sea (1.01 °C decade−1) and the Ionian Sea (1.17 °C decade−1)19. During the 2013–2019 period, the decadal MTC increasing rate (4.11 °C
decade−1) largely exceeded any regional estimate reported, a pattern consistent with the expected ecosystem changes in a region of intense ocean temperature increase (Fig. 1)23,24. SBT
values remained relatively stable from 2001 to 2010 but increased continuously from 2013 to 2019 (Fig. 3), as confirmed by the significant positive linear trend (0.077 °C yr−1, _p_-value =
0.012) (Table 1). MTC variation was significantly explained by SBT with 0 and 1-year time-lag (_p_-value = 0.001 and _p_-value = 0.023, respectively) (Table 2). These results were also
consistent with global patterns17 but particularly relevant were the trends described in the Argentinean–Uruguayan Common Fishing Zone (AUCFZ, Fig. 1), where an MTC warming trend was
described from 1985 to 201721. This trend was explained by sea surface temperature (SST) variation which also increased steadily since mid-1990. Authors suggested that an important
‘oceanographic change has occurred in the region, which modulated the MTC index’. Because the MTC series analysed in the BMM is shorter (2000–2019) than the one analysed in the AUCFZ
(1973–2017), and considered temperature at the sea bottom rather than at the surface (SST), a direct comparison between regions is not fully informative. However, the INALT20 model-derived
SBT time-series, available since 195847 (Fig. 3), showed that positive anomalies became frequent from 1994 onwards, as observed in the AUCFZ SST time-series, indicating that such
oceanographic change was noticed in both adjacent regions of the SWAO. Notwithstanding, in the BMM a noticeable steady increase of both MTC and SBT seems to take place from 2012 onwards,
suggesting a more recent regional shift. If only this time period is analysed in the AUCFZ time series (Fig. 4 in ref. 21) positive anomalies would predominate approximately from 2010
onwards and years of maximum MTC anomalies would occur after 2014, coinciding with those identified in the BMM time series. This suggests that the signal of the second MTC shift is also
present in the AUCFZ. Several studies have demonstrated that an SWAO general warming process is associated with the poleward expansion of the Brazil Current and the Brazil–Malvinas
Confluence26,48. Artana et al.27 showed that this feature migrated southward between 1997 and 2006, oscillating widely thereafter (with southernmost positions in 1998, 2004, 2011, 2015 and
2017) and that Brazil's Current transport volumes tended to increase from 1998 to 2016. These trends are consistent with (a) periods of intensified SBT positive anomalies in the BMM
time-series, and (b) the positive effect of SBT and BCt on MTC variation. BCt affected MTC with a 3-year (_p_-value = 0.031) and 4-year (_p_-value = 0.029) time-lags (Table 2). The reason
for such a delayed response is uncertain, but possible explanations could be related to the complex physical interactions between the Brazil Current and the BMM shelf waters. Gianelli et
al.21 argued that whereas many species in this dynamic transition region may be adapted to environmental oscillations, such a sustained oceanographic change would gradually provoke
‘unprecedented changes in the composition and structure of ecological assemblages’. The caveat here, however, is that fishing data may affect the MTC analyses in different ways, e.g. through
market-oriented behaviour of fishing fleets, which tend to establish temporal and spatial strategies in pursuit of profitable concentrations of their main fishing targets46. In the BMM,
demersal fishing fleets explore a great variety of resources available in geographical space and different seasons in order to attain economic stability42. By doing so, they tend to
integrate, in their catch composition, a wide spectrum of megafauna communities. However, trawl and gillnet vessels have developed a variety of _métiers_, i.e. a combination of target
species, fishing area, gear, and time of the year49,50, whose operational patterns in the BMM could partially modulate MTC variation. For instance, if fishing operations of a particular
_métier_ aiming at an abundant cold-water species predominated in relation to operations of other _métiers_ during a year, an environment-independent MTC drop would be observed in such a
year. We assessed these fishery-dependent effects in two ways. Firstly, an annual index of _métier_ diversity (Dm, based on Simpson species diversity index) was computed and used to express
the effect of dominance (low Dm values) vs. evenness (high Dm values) of _métiers_ in the catches. Annual _Dm_ did not exhibit any particular trend along the analysed time-series (_p_-value
> 0.08, Table 1) and affected negatively MTC with a 4-year time-lag (_p_-value = 0.002, Table 2). This approach was first proposed by Gianelli et al.21, who found similar results in the
AUCFZ, i.e. the effect of fishing _métiers_ on MTC time-series was either non-significant or in an opposed direction to that exerted by the ocean temperature. Secondly, we submitted the MTC
time-series to a species sensitivity analysis, showing that the accentuated positive trend of MTC, observed between 2013 and 2019, remained unchanged no matter which species we removed from
the analysis (Table 3). Between 2000 and 2012, when no particular trend was evidenced in the MTC time series (Table 1), the estimated slope of the linear model increased, becoming
significantly positive, when the codling _Urophycis mystacea_ was excluded from the time-series (Table 3). This is an abundant slope species (mean thermal preference = 16 °C) whose catches
remained above average between 2007 and 2013 (Supplementary Fig. 3), mostly through the activity of a double-rig trawl _métier_ which included slope species in the period (DR_1,
Supplementary Table 3). In the AUCFZ, the exclusion of the most abundant argentine hake (_Merluccius hubsi_; cold-water affinity) and the whitemouth croaker (_Micropogonias furnieri_;
warm-water affinity) from the catch time-series changed considerably MTC variation21. Important catch reductions of the former have been attributed to overfishing, which has also a potential
for modulating MTC time-series. This can be the case of several cold- and warm-water species that largely contributed to BMM demersal catches during the studied period, whose exploitation
regimes were categorized as unsustainable51. In this region, for instance, important abundance declines of the cold-water argentine croaker (_Umbrina canosai_, from 2010 onwards) and the
monkfish (_Lophius gastrophysus_, from 2000 onwards) in the BMM (Cardoso et al., unpublished results) could modulate MTC leading towards a catch warming scenario. However, at least in the
latter species, biomass levels have remained extremely low after 2010, a period when the fishing effort was maintained below critical levels, suggesting that factors other than fishing
pressure (i.e. ocean warming) could be driving temporal patterns of the species abundance. In any case, as pointed out in other MTC study regions19,20, overfishing effects on MTC seems
hardly dissociable from, for instance, poleward retractions of cold-water species and, in fact, may have a synergistic effect. CATCH COMPOSITION ANALYSIS Changes in species abundances in the
catches of the demersal fisheries in the BMM evidence strong contrasts between the early (2000–02) and late (2017–19) periods of the time-series. These periods were aggregated into two
largely dissimilar year-groups by the multiple regression tree-principal coordinate analysis (Fig. 4), which discriminated an initial scenario (Group I), when annual catches were
characterized by 15 main species, most of them with cold-water affinity (species on quadrants 1 and 2 in Fig. 4b), from a late scenario (Group IV) defined by scores attributed by eight
species mostly with warm-water affinity (species in quadrant 3, Fig. 4b). Such a contrast was also corroborated by (a) the elevated contributions of these year-groups to the estimated total
beta diversity, statistically significant in 2000 (14.2%), 2001 (10.8%), 2002 (9.2%) and 2019 (10.1%) (Supplementary Fig. 4), and (b) the Temporal Beta Diversity indices (TBI) comparing
years within Groups I and IV, which resulted in significant losses in species abundance (Fig. 5). It is important to note that mean biomass gains and losses were significant between these
groups (second largest among the periods compared), and dominated by warm- and cold-water species, respectively (Fig. 6, Supplementary Table 2). The argentine croaker (_Umbrina canosai_)
concentrated 15.4% of cold-water species biomass losses in the catches, along with the argentine hake (_Merluccius hubbsiI_), the argentine stiletto shrimp (_Artemesia longinaris_), the
monkfish (_Lophyus gastrophysus_) and others (Supplementary Table 2, Supplementary Fig. 3). The whitemouth croaker (_Micropogonias furnieri_) concentrated 25.6% of warm-water species biomass
gains in the catches, followed by the grey triggerfish (_Balistes capriscus_) and the spotted pink shrimp (_Penaeus brasiliensis_) (Supplementary Table 2, Supplementary Fig. 2). Jointly,
these biomass gains and losses contributed to a warming of the catches between the two extreme periods, and supported the process of tropicalization, as revealed by the MTC analysis. Catch
composition analysis also suggested that the important changes in the demersal assemblages, as proposed by Gianelli et al.21, may have taken place in the BMM between 2003 and 2012. Unlike in
the MTC analysis, however, a more precise shift period was not evident. The progression of years in the 2-D ordination plot (Fig. 4) suggested a temporal modification in catch composition
from the initial scenario (Group I), when cold-water species were abundant in the catches (species on quadrants 1 and 2 in Fig. 4b), to two intermediate scenarios (Groups II and III) when
these species were gradually less abundant and substituted by other cold-water species (on quadrant 4 in Fig. 4b). TBIs calculated between years within Groups I and II produced three
significant comparisons, two indicating losses (2000/2003, 2000/2006) and one indicating gains (2000/2007) (Fig. 5). There were important mean biomass gains between these two periods (Fig.
6), concentrated in the warm-water whitemouth croaker (_M. furnieri_, 39.0%) and striped weakfish (_Cynoscion guatucupa_, 10.3%), and the cold-water argentine croaker (14.9%) and codling
(13.1%) (Supplementary Table 2). Biomass changes were limited between years within Groups II and III. Cold-water species, chiefly the codling (_U. mystacea_) and the argentine croaker (_U.
canosai_), dominated both gains and losses of biomass, respectively (Fig. 6, Supplementary Table 2), but TBI comparisons indicated that these were not significant changes (Fig. 5). The last
transition in the catch composition (Groups III and IV) was marked by great biomass losses mostly of cold-water species (Fig. 6), including the codling (_U. mystacea_, 16.3%), Argentine
croaker (_M. furnieri_, 15.3%), the Argentine stiletto shrimp (_A. longinaris_, 9.7%), the Argentine hake (_M. hubbsi_, 6.9%), the monkfish (_L. gastrophysus_, 2.4%) and others
(Supplementary Table 2). TBI comparisons also indicated species losses, but they were significant only in relation to the year 2019 (Fig. 5). Interpreting such changes in demersal catch
composition, in light of the warming trend in the SWAO, required prior consideration of the physical processes associated with known patterns of the spatial distribution of fish and
shellfish populations46. In the BMM, demersal fauna diversity tends to change from typically subtropical in the South Brazil Bight (23°S–28°S) to a mixed subtropical/warm-temperate towards
the ‘central shelf’ subregion (south of 28°S, Fig. 1), which comprises the continental shelf area off southern Brazil, Uruguay and northern Argentina33. In this subregion, off southern
Brazil, Martins and Haimovici39 described four teleost fish demersal assemblages formed by species with similar temperature affinities, whose latitudinal and bathymetric distribution are
associated with seasonal interactions of coastal, subantarctic and subtropical shelf water masses. A ‘cold shelf assemblage’ was shown to expand over mid-shelf bottoms during the austral
winter, as driven by the increased influence of subantarctic shelf waters and the northward displacement of Subtropical Shelf Front. This assemblage contained some abundant cold-water
species present in the demersal catches, including the argentine croaker (_U. canosai_) and the argentine hake (_M. hubbsi_), which have accounted for important biomass losses (>20%) in
the BMM. In addition, a ‘coastal’ and a ‘warm shelf’ assemblages were shown to expand southwards over the shelf during the austral summer. These assemblages contained fish species with
warm-water affinity, including the whitemouth croaker (_M. furnieri_), which alone accounted for 25% of biomass gains in demersal catches. In an ocean warming scenario, induced by the
southward displacement of the Brazil Current and its influence over the shelf, a southward retraction of the ‘cold water shelf’ assemblage and expansion of the ‘coastal’ and ‘warm water
shelf’ assemblages would be expected, justifying the observed _MTC_ trends and temporal patterns of species abundance in the BMM demersal catches. Deviations from this general pattern,
however, were also characterized partially because targeted species may display different levels of adaptation and respond differently to a warming environment13. For instance, the
whitemouth croaker (_M. furnieri_) contributed significantly to warm-water species biomass gains in the period, but also to biomass losses. The species exhibits a complex stock structure
that includes three spatially delimited stocks: one occupying the South Brazil Bight (‘Southeastern Brazil Stock’) and two extending over the central shelf off southern Brazil (‘Southern
Brazilian Stock’) and at the AUCFZ (‘common Argentinean—Uruguayan stock’)52. Despite its warm-water affinity, the species exhibits a wide thermal tolerance, making it plausible that these
stocks display some level of adaptation to local conditions and respond differently to the ocean warming process they have been exposed to in the SWAO. In addition, the La Plata River and
the Patos/Mirim Lagoon systems are important nursery grounds for this species that may also respond to other climate-change-related effects such as freshwater discharge variability in these
systems21. In that sense, the general tropicalization scenario characterized in the BMM demersal catches may be affected in different ways by multiple specific population processes operating
at smaller spatial scales. An additional source of error may derive from thermal preferences being attributed to demersal species from sea surface temperature distribution17. How much this
affected MTC temporal trends in the BMM is uncertain but it was likely dampened by (a) the large proportion of catches originating from species in shallow shelf areas where temperatures are
more homogeneous throughout the water column and (b) a positive correlation between SST and SBT values derived from the INALT20 model (_r_ = 0.84) suggesting that, regionally, MTC calculated
with surface temperatures should still be related to thermal changes on the seafloor. Finally, annual MTC may also be modulated by spatial factors (e.g. latitude, depth) and trophic
levels22,46. Addressing these components is critical for a more comprehensive analysis of temperature-related community changes in the BMM. Notwithstanding such limitations, historical catch
data has proven to be an effective proxy for global climate effects on marine ecosystems regionally, with the advantage of further signalling future changes in the economic performance of
current fishing regimes. How will the demersal fishing industry adapt to changes in the availability of traditional and non-traditional targets in the BMM? Which _métiers_ will no longer be
viable and which ones may emerge to explore expanding stocks of subtropical species? What adaptive measures can be incorporated into fishing management regimes (both national and
transnational) to attain ecological and economic objectives in the coming decades? These are critical questions that could influence industry adaptive strategies and guide management
measures over the next decades in the BMM, but whose answers will require extended analyses, with a database expanded to include spatial components and fisheries economic descriptors. As a
preliminary approach, however, we may infer that most _métiers_ have included cold-water species among their most frequent targets (Supplementary Table 3) and will potentially face the need
to adapt their fishing strategies. Particularly relevant seems the future performance of double-rig trawlers (_métier_ DR_1) that have largely dominated the demersal fishing activity in the
study period (Supplementary Table 1). Their operations, however, have long been driven by a generalist fishing behaviour and will likely be adaptable to new opportunities, as seen in the
past49. Gillnet fishing (_métier_ GN_1) was the second most active demersal fishing activity heavily focused on the whitemouth croaker (Supplementary Table 3). Whereas this may appear as a
winner species under an ocean warming scenario, it is uncertain how long could it sustain fishing pressures increased by the effect of the progressively scarce cold-water target-species
(e.g. _métier_ GN_2 and others)53. Trawling for the warm-water sea-bob shrimp (_Xyphopenaeus kroyeri_ - _métier_ DR_2) can be an opportunity for trawlers in the future, especially those
targeting cold-water shrimps (_A. longinaris_ and _Pleoticus muelleri_—DR_3) (Supplementary Table 3). Such a perspective, however, will likely be limited by the increased competition with
artisanal fisheries that have long exploited this coastal species in the BMM54. Finally, the likely decline of the argentine croaker in the catches may threaten the future viability of trawl
(_métiers_ PT_2, ST_2, ST_3) and gillnet (_métier_ GN_2) operations that currently concentrate in the central shelf region. These catches have been mostly sustained by a population that
migrates between the northern Patagonian shelf and southern Brazil55 suggesting that, in the future, these _métiers_ could only be viable south of Brazilian waters. These are some possible
scenarios that indicate how demersal fisheries can be reshuffled at the BMM, calling for adaptive management that will require rigorous assessments of sustainable catches of subtropical
species, and include actions that redistribute effort in areas that take into account species with changing distribution patterns, including transboundary stock management56. METHODS CATCH
COMPOSITION, THERMAL PREFERENCES AND OCEANOGRAPHIC DATA Analysed data included catches reported in the harbours of Santa Catarina state (Itajaí and Navegantes) from 2000 to 2019. These
harbours have historically concentrated approximately 25% of total catches reported in the region57 and a significant part of the demersal fishing fleet that operates widely on the BMM (Fig.
1), from 21°S to the southern border of the Brazilian Economic Exclusive Zone (34°S), and from the coastal areas down to 500 m depths. Landed catches were monitored by the University of
‘Vale do Itajaí’ along a sequence of scientific projects and contracts developed to meet governmental demands for oceanic and deep fisheries development and management and in support of the
licencing processes of the offshore oil and gas exploration activities. Monitored demersal catches have been dominated by sciaenid fish (e.g. the whitemouth croaker (_M. furnieri_), the
argentine croaker (_U. canosai_), the striped weakfish (_C. guatucupa_), the southern king weakfish (_Macrodon atricauda_) and the shrimps _X. kroyeri_ (Atlantic sea-bob shrimp), _A.
longinaris_ (argentine stiletto shrimp), _Penaeus paulensis_ (São Paulo pink shrimp) and _Penaeus brasiliensis_ (spotted pink shrimp). Demersal fishing expanded to the upper slope from 2001
onwards adding some new fishing resources, e.g. the codling _U. mystacea_ and the monkfish _L. gastrophysus_43. We analysed landings of 29,021 fishing trips conducted by double-rig trawlers
(56.4%), pair trawlers (6.9%), stern trawlers (5.7%) and gillnet vessels (31.0%) (Supplementary Table 1). The number of fishing trips recorded each year varied between 561 and 2,036, and
total catches varied between 11,000 and 53,000 t yr−1 during the studied period (2000–2019) (Supplementary Fig. 1). Reported catches contained records of 133 fish and shellfish categories.
These were defined by single species or groups of species (e.g. rays, _Squalus_ spp.). Only single species categories were included in the analysis, which resulted in a total of 78 species
jointly representing 81.3% of total reported biomass. A temperature preference was assigned to each of the considered species, as obtained from global compilations made available by Cheung
et al.17 and in FishBase58. In both compilations, thermal preferences derive from considerations about the species distribution ranges and sea surface temperature maps (e.g. ref. 17,
Supplementary material). An overall mean temperature preference value was calculated for all 78 species combined (21.1 °C) and used to assign warm- or cold-water affinities for species whose
thermal preferences were above or below this value, respectively. The mean temperature of the catch—MTC was estimated for each year (_y_) of the time series, as proposed by Cheung et al.17:
$${{{\rm {MTC}}}}_{y}=\frac{{\sum }_{i}^{n}{T}_{i}{C}_{i,y}}{{\sum }_{i}^{n}{C}_{i,y}}$$ (1) where _n_ is the total number of species recorded in one year, _T__i_ is the temperature
preference of the _i_th species and _C__i,y_ is the recorded catch of the _i_th species in the _y_th year. The composition of demersal catches and MTC time-series are publicly available at
Perez and Sant’Ana59. Sea bottom temperature (SBT) was considered a predictor of MTC variation during the study period. SBT was derived from estimates provided by the high-resolution ocean
general circulation model INALT2047 for the study period (2000–2019), and was calculated by averaging the temperatures over 0.25° × 0.25° grid cells of the BMM and a water column up to 50 m
above the seafloor. Additionally, MTC was confronted to annual volume transports of the Brazil Current (BCt) near the Brazil–Malvinas confluence (in Sverdrups, Sv) as estimated between
2000–2017 by Artana et al.27 using high‐resolution (1/12°) global Mercator Ocean reanalysis (GLORYS12) from Copernicus Marine Environment Monitoring Service (CMEMS,
http://marine.copernicus.eu/). All BCt values are negative, as they represent north–south transport27. Annual values of both variables were normalized by their mean value over the time
series and expressed as anomalies. In Fig. 1, daily gridded sea surface temperature (SST) data were obtained from the National Oceanic and Atmospheric Administration Optimum Interpolation
Sea Surface Temperature (OISST) V2.0 with a horizontal resolution of 1/4° for the period 1982–202060. The Mann and Kendall test was used to determine where the trends were statically
significant at the 99th confidence interval. In addition, we tested the effect of fishers’ behaviour over catch composition, which could introduce environment-independent signals in the MTC
(e.g. driven by market oscillations and other factors). For that purpose, individual fishing trips within the database were firstly classified by _métiers_ (i.e. combination of target
species, gear, and time of the year) using the _K_-means clustering algorithm61. Within the years of the time-series, catches of each _métier_ were summed and used to calculate an annual
index of _métier_ diversity (Dm) using the Simpson diversity index formulation21: $${{Dm}}_{y}=1-{\sum }_{m=1}^{M}{\left(\frac{{C}_{m,y}}{{C}_{y}}\right)}^{2}$$ (2) where _m_ is the fishing
_métier_ and _M_ is the total number of _métiers_ defined in the time-series. Temporal trends of MTC, SBT, BCt and Dm were explored by fitting linear models to their variation through time.
A segmented regression model was also adjusted to MTC time series in order to detect potential trend shifts through time and their association with SBT, BCt and Dm variation. Estimated MTC
trends were tested for the influence of individual species in the catch data (species sensitivity analysis). This procedure intended to verify whether catch variation of the most abundant
species could modulate MTC variation, significantly masking the combined effect of the wider group of species present in the catches. In this analysis, the linear models fitted to MTC along
time were adjusted to scenarios where the species were iteratively excluded one-by-one. In each scenario, the estimated slope of the regression was compared to the slope obtained with all
species included and verified whether the original trend was maintained or significantly changed. The effect of the environmental predictors _SBT_, BCt and Dm, over MTC variation was tested
by fitting linear models that included a time-lag structure of 0–4 years, intended to verify any delayed responses of MTC to SBT, BCt and Dm variation. CATCH COMPOSITION ANALYSIS The
patterns of change in the abundance of species present in the BMM demersal catches along the 19-year time-series were explored using ordination methods and estimates of beta diversity.
Initially, a multiple regression tree procedure was applied to Hellinger-transformed annual species catches (abundance data) using the _mvpart_ fuction of R package mvpart62. The size of the
tree (i.e. number of splits) was selected after calculating the cross-validation error and deciding between the best-fitted and more parsimonious models44. A principal coordinate analysis
was applied to the Hellinger distances to ordinate years in the 2-D (Euclidean) space and explore patterns of similarity/dissimilarity among years and among groups of years as previously
defined by the MRT analysis44. The total non-directional beta diversity (BDtotal) was estimated by computing the total sum of squares (SStotal) of the years vs. species matrix and the total
variance by dividing SStotal by _n_−1. BDtotal was further partitioned into relative contributions of years (here named YCBD)63. YCBD estimates were tested for significance by 999 random
independent permutations of the columns of years _vs_. species matrix, using the _beta.div_ function of R package adespatia’64. This analysis was used to identify year(s) when the catch
composition was particularly altered. Temporal changes in catch composition were investigated by computing temporal beta diversity indices (TBI), using the TBI function of R package
adespatial64. This procedure involved computing Percentage Difference dissimilarity indices between years (two-by-two) and partitioning these dissimilarities into gains (1 > TBI > 0)
and losses (0 > TBI > −1)65. The computed difference between gains and losses was tested using a paired _t_-test. Patterns of gains and losses between time periods (e.g. groups of
similar years as defined by the ordination methods) were investigated by analysing catch variation of individual species and thermal preferences. REPORTING SUMMARY Further information on
research design is available in the Nature Research Reporting Summary linked to this article. DATA AVAILABILITY The time series (2000–2019) of catch composition and mean catch temperature
for demersal species in the Brazilian Meridional Margin that support the findings of this study are available in Pangea Data Publisher for Earth & Environmental Science with the
identifier https://doi.org/10.1594/PANGAEA.94629259. CODE AVAILABILITY The figures and data analyses presented in this manuscript were conducted using R 4.2.0, along with the following R
packages: mvpart 62, adespatial 64. Custom code for the analyses can be accessed here: https://github.com/rodrigosantana/DemersalTropicalizationBMM. All other code used to make figures is
available upon request by contacting the corresponding author. REFERENCES * IPCC. Summary for Policymakers. In _IPCC Special Report on the Ocean and Cryosphere in a Changing Climate_ (eds.
Pörtner, H.-O. et al.) (IPCC, 2019). * Fu, W., Randerson, J. & Moore, J. K. Climate change impacts on net primary production (NPP) and export production (EP) regulated by increasing
stratification and phytoplankton community structure in the CMIP5 models. _Biogeosciences_ 13, 5151–5170 (2016). Article Google Scholar * Schmidtko, S., Stramma, J. & Visbeck, M.
Decline in global oceanic oxygen content during the past five decades. _Nature_ 542, 335–339 (2017). Article CAS Google Scholar * Trenberth, K. E., Cheng, L., Jacobs, P., Zhang, Y. &
Fasullo, J. Hurricane Harvey links to ocean heat content and climate change adaptation. _Earth’s Future_ 6, 730–744 (2018). Article Google Scholar * Caesar, L., McCarthy, G. D.,
Thornalley, D. J. R., Cahill, N. & Rahmstorf, S. Current Atlantic Meridional Overturning Circulation weakest in last millennium. _Nat. Geosci._ 14, 118–120 (2021). Article CAS Google
Scholar * Doney, S. C., Fabry, V. J., Feely, R. A. & Kleypas, J. A. Ocean Acidification: the other CO2 problem. _Annu. Rev. Mar. Sci._ 2009.1, 169–192 (2009). Article Google Scholar *
Jennings, S. et al. Global-scale predictions of community and ecosystem properties from simple ecological theory. _Proc. Biol. Sci._ 275, 1375–1383 (2008). Google Scholar * Dulvy, N. et
al. Climate change and deepening of the North Sea fish assemblage: a biotic indicator of warming seas. _J. Appl. Ecol._ https://doi.org/10.1111/j.1365-2664.2008.01488.x (2008). * Cheung, W.
L. et al. Projecting global marine biodiversity impacts under climate change scenarios. _Fish Fish._ 10, 235–251 (2009). Article Google Scholar * Blanchard, J. L. et al. Potential
consequences of climate change for primary production and fish production in large marine ecosystems. _Philos. Trans. R. Soc. B_ 367, 2979–2989 (2012). Article Google Scholar *
Poloczanska, E. S., Brown, C. J., Sydeman, W. J., Kiessling, W. & Schoeman, D. S. Global imprint of climate change on marine life. _Nat. Clim. Change_ 3, 919–925 (2013). Article Google
Scholar * Poloczanska, E. S. et al. Responses of marine organisms to climate change across oceans. _Front. Mar. Sci._ 3, 62 (2016). Article Google Scholar * Hu, N., Bourdeau, P. E.,
Harlos, C., Liu, Y. & Hollander, J. Meta-analysis reveals variance in tolerance to climate change across marine trophic levels. _Sci. Total Environ._ 827, 154244 (2022). Article CAS
Google Scholar * Pecl, G. T. et al. Biodiversity redistribution under climate change: Impacts on ecosystems and human well-being. _Science_ 355, eaai9214 (2017). Article Google Scholar *
Fujiwara, M. et al. Climate-related factors cause changes in the diversity of fish and invertebrates in subtropical coast of the Gulf of Mexico. _Commun. Biol._ 2, 403 (2019). Article
Google Scholar * Chaudhary, C., Richardson, A. J., Schoeman, D. S. & Costello, M. J. Global warming is causing a more pronounced dip in marine species richness around the equator.
_Proc. Natl Acad. Sci. USA_ 118, e2015094118 (2021). Article CAS Google Scholar * Cheung, W. L., Watson, R. & Pauly, D. Signature of ocean warming in global fisheries catch. _Nature_
497, 365–369 (2013). Article CAS Google Scholar * Keskin, Ç. & Pauly, D. Changes in the ‘Mean Temperature of the Catch’: application of a new concept to the North-eastern Aegean Sea.
_Acta Adriat._ 55, 213–218 (2014). Google Scholar * Tsikliras, A. C., Peristeraki, P., Tserpes, G. & Stergiou, K. I. Mean temperature of the catch (MTC) in the Greek Seas based on
landings and survey data. _Front. Mar. Sci._ 2, 23 (2015). Article Google Scholar * Liang, C., Xian, W. & Pauly, D. Impacts of ocean warming on China’s Fisheries catches: an
application of “mean temperature of the catch” concept. _Front. Mar. Sci._ 5, 26 (2018). Article Google Scholar * Gianelli, I., Ortega, L., Marín, Y., Piola, A. R. & Defeo, O. Evidence
of ocean warming in Uruguay’s fisheries landings: the mean temperature of the catch approach. _Mar. Ecol. Prog. Ser._ 625, 115–125 (2019). Article Google Scholar * Lekanda, A., Tolimieri,
N. & Nogueira, A. The effects of bottom temperature and fishing on the structure and composition of an exploited demersal fish assemblage in West Greenland. _ICES J. Mar. Sci._ 78, 1–12
(2021). Article Google Scholar * Hobday, A. J. & Pecl, G. T. Identification of global marine hotspots: sentinels for change and vanguards for adaptation action. _Rev. Fish. Biol.
Fish._ 24, 415–425 (2014). Article Google Scholar * Popova, E. et al. From global to regional and back again: common climate stressors of marine ecosystems relevant for adaptation across
five ocean warming hotspots. _Global Change Biol._ https://doi.org/10.1111/gcb.13247 (2016). * Franco, B. et al. Climate change impacts on the atmospheric circulation, ocean, and fisheries
in the southwest South Atlantic Ocean: a review. _Clim. Change_ 1, https://doi.org/10.1007/s10584-020-02783-6 (2020). * Lumpkin, R. & Garzoli, S. Interannual to decadal changes in the
western South Atlantic’s surface circulation. _J. Geophys. Res._ 116, C01014 (2011). Google Scholar * Artana, C. et al. The Malvinas Current at the confluence with the Brazil Current:
inferences from 25 years of Mercator Ocean reanalysis. _J. Geophys. Res.: Oceans_ 124, 7178–7200 (2019). Article Google Scholar * Alberoni. A. A. L., Jeck, I. K., Silva, C. G. &
Torres, L. C. The new Digital Terrain Model (DTM) of the Brazilian Continental Margin: detailed morphology and revised undersea feature names. _Geo-Mar. Lett._
https://doi.org/10.1007/s00367-019-00606-x (2019). * Silveira, I. C. A., Napolitano, D. C. & Farias, I. U. Water masses and oceanic circulation of the Brazilian Continental Margin and
adjacent abyssal plain. In _Brazilian marine biodiversity_ (eds Sumida, P. Y. G., Bernardino, A. F. & DeLeo, F. C.) 7–36 (Springer, 2020). * Campos, E. J. D., Velhote, D. & Silveira,
I. C. A. Shelf break upwelling events driven by the Brazil current cyclonic meanders. _Geophys. Res. Lett._ 27, 751–754 (2000). Article Google Scholar * Palma, E. D. & Matano, R. P.
Disentangling the upwelling mechanisms of the South Brazil Bight. _Cont. Shelf Res._ 29, 1525–1534 (2009). Article Google Scholar * Piola, A. R., Möller, O. O. Jr., Guerrero, R. A. &
Campos, E. J. D. Variability of the subtropical shelf front off eastern South America: Winter 2003 and Summer 2004. _Cont. Shelf Res._ 28, 1639–1649 (2008). Article Google Scholar * Piola,
A. R. et al. Physical oceanography of the SW Atlantic Shelf: a review. In _Plankton Ecology of the Southwestern Atlantic_ (eds Hoffmeyer, M. S. et al.) 37–56 (Springer, 2018). * Briggs, J.
C. & Bowen, B. W. A realignment of marine biogeographic provinces with particular reference to fish distributions. _J. Biogeogr._ 39, 12–30 (2012). Article Google Scholar * Spalding,
M. D. et al. Marine ecoregions of the world: a bioregionalization of coastal and shelf areas. _BioScience_ 57, 573–583 (2017). Article Google Scholar * Pinheiro, H. T. et al. South-western
Atlantic reef fishes: zoogeographical patterns and ecological drivers reveal a secondary biodiversity centre in the Atlantic Ocean. _Divers. Distrib._ 24, 951–965 (2018). Article Google
Scholar * Caires, R. Biogeografia dos peixes marinhos do Atlântico Sul ocidental: Padrões e Processos. _Arq. Zool. Mus. Zool. Univ. São Paulo_ 45, 5–24 (2014). Google Scholar * Haimovici.
M. Demersal and Benthic Teleosts. In _Subtropical Convergence Environments_ (eds Seeliger, U., Odebrecht, C., Castello, J. P.) 129–135 (Springer-Verlag, 1997). * Martins, A. S. &
Haimovici, M. Seasonal mesoscale shifts of demersal nekton assemblages in the subtropical South-western Atlantic. _Mar. Biol. Res._ https://doi.org/10.1080/17451000.2016.1217025 (2016). *
Haimovici, M., Martins, A. S., Figueiredo, J. L. & Vieira, P. C. Demersal bony fish of the outer shelf and upper slope of the southern Brazil subtropical convergence ecosystem. _Mar.
Ecol. Prog. Ser._ 108, 59–77 (1994). Article Google Scholar * Valentini. H. & Pezzuto. P. R. _Análise das principais pescarias comerciais da região Sudeste-Sul do Brasil com base na
produção controlada do período 1986–2004_. _Série Documentos Revizee: Score Sul_ (Instituto Oceanográfico—USP, São Paulo, 2006). * Rossi-Wongtschowski, C. L. D. B., Bernardes, R. A. &
Cergole, M. C. Dinâmica das _Frotas Pesqueiras Comerciais da Região Sudeste-Sul do Brasil_. _Série Documentos Revizee: Score-Sul_ 343 (Instituto Oceanográfico, Universidade de São Paulo, São
Paulo, 2007). * Perez, J. A. A., Pezzuto, P. R., Wahrlich, R. & Soares, A. L. S. Deep-water fisheries in Brazil: history. status and perspectives. _Lat. Am. J. Aquat. Res._ 37, 513–541
(2009). Article Google Scholar * Legendre, P. & Legendre, L. _Numerical Ecology_ 3rd English edn (Elsevier Science BV, 2012). * Araújo, F. G., Teixeira, T. P., Guedes, A. P. P.,
Azevedo, M. C. C. & Pessanha, A. L. M. Shifts in the abundance and distribution of shallow water fish fauna on the southeastern Brazilian coast: a response to climate change.
_Hydrobiologia_ 814, 205–218 (2018). Article Google Scholar * Punzón, A. et al. Tracking the effect of temperature in marine demersal fish communities. _Ecol. Indic._ 121, 107142 (2021).
Article Google Scholar * Schwarzkopf, F. U. et al. The INALT family—a set of high-resolution nests for the Agulhas Current system within global NEMO ocean/sea-ice configurations. _Geosci.
Model Dev._ 12, 3329–3355 (2019). Article Google Scholar * Matano, R. P., Palma, E. D. & Piola, A. R. 2010. The influence of the Brazil and Malvinas Currents on the Southwestern
Atlantic Shelf circulation. _Ocean Sci._ 6, 983–995 (2010). Article Google Scholar * Dias, M. C. & Perez, J. A. A. Multiple strategies developed by bottom trawlers to exploit fishing
resources in deep areas off Brazil. _Lat. Am. J. Aquat. Res._ 44, 1055–1068 (2016). Article Google Scholar * Pio, V. M., Pezzuto, P. R. & Wahrlich, R. Only two fisheries?
Characteristics of the industrial bottom gillnet fisheries in southeastern and southern Brazil and their implications for management. _Lat. Am. J. Aquat. Res._ 44, 882–897 (2016). Article
Google Scholar * Haimovici, M. & Cardoso, L. G. Long-term changes in the fisheries in the Patos Lagoon estuary and adjacent coastal waters in Southern Brazil. _Mar. Biol. Res._
https://doi.org/10.1080/17451000.2016.1228978 (2017). * Haimovici, M., Cardoso, L. G. & Umpierre, R. G. Stocks and management units of _Micropogonias furnieri_ (Desmarest, 1823) in
southwestern Atlantic. _Lat. Am. J. Aquat. Res._ 44, 1080–1095 (2016). Article Google Scholar * Haimovici, M., Cavole, M. L., Cope, J. & Cardoso, L. G. Long-term changes in population
dynamics and life history contribute to explain the resilience of a stock of _Micropogonias furnieri_ (Sciaenidae, Teleostei) in the SW Atlantic. _Fish. Res._ 237, 105878 (2021). Article
Google Scholar * Franck, K. M. & Dantas-Filho, J. V. A pesca de camarões na região sul do Brasil. _Rev. Gest. Sustentab. Ambient._ 8, 499–520 (2019). Google Scholar * Kikuchi, E.,
Cardoso, L. G., Canel, D., Timi, J. T. & Haimovici, M. Using growth rates and otolith shape to identify the population structure of _Umbrina canosai_ (Sciaenidae) from the Southwestern
Atlantic. _Mar. Biol. Res._ 17, 272–285 (2021). Article Google Scholar * Poulain, F. et al. _FAO Fisheries and Aquaculture Technical Paper_ 627. 535–566 (FAO, 2018). * BRASIL/MPA. _Boletim
Estatístico da Pesca e Aquicultura_ (Ministério da Pesca e Aquicultura (MPA), 2012). * Froese, R. & Pauly, D. FishBase. _World Wide Web electronic publication_. www.fishbase.org version
02/2022 (2022). * Perez, J. A. A. & Sant’Ana, R. Time series (2000–2019) of catch composition and mean catch temperature for demersal species in the Brazilian Meridional Margin. PANGAEA
https://doi.org/10.1594/PANGAEA.946292 (2022). * Reynolds, R. W. et al. Daily high-resolution-blended analyses for sea surface temperature. _J. Clim._ 20, 5473–5496 (2007). Article Google
Scholar * Steinley, D. & Brusco, M. J. Initializing _k_-means batch clustering: a critical evaluation of several techniques. _J. Classif._ 24, 99–121 (2007). Article Google Scholar *
Therneau, T. M. & Atkinson, B. _mvpart: multivariate partitioning_. R package version 1.6-2 https://CRAN.R-project.org/package=mvpart (2014). * Legendre, P. & De Cáceres, M. Beta
diversity as the variance of community data: dissimilarity coefficients and partitioning. _Ecol. Lett._ 16, 951–963 (2013). Article Google Scholar * Dray, S. et al. _adespatial:
multivariate multiscale spatial analysis_. R package version 0.3-14 https://CRAN.R-project.org/package=adespatial (2021). * Legendre, P. A temporal beta-diversity index to identify sites
that have changed in exceptional ways in space-time surveys. _Ecol. Evol._ 9, 3500–3514 (2019). Article Google Scholar Download references ACKNOWLEDGEMENTS Authors acknowledge the support
of the EU H2020-BG-2018-2020 project iAtlantic—‘Integrated Assessment of Atlantic Marine Ecosystems in Space and Time’ (Grant Agreement 818123) and of the State of Santa Catarina Research
and Innovation Foundation—FAPESC (Grant number: 2021TR001239). We are indebted to Kristin Burmeister (Scottish Association for Marine Science, UK) and Regina Rodrigues Rodrigues (Federal
University of Santa Catarina, Brazil) for the provision of the oceanographic data series analysed in this study. J.A.A.P. is supported by the National Council for Scientific and Technologic
Development—CNPq, through the National Institute of Science and Technology—INCT Mar-COI (Process 400551/2014-4) and a productivity fellowship (Process 307992/2019-5). AUTHOR INFORMATION
AUTHORS AND AFFILIATIONS * School of Sea, Science and Technology – EMCT, University of Vale do Itajaí – UNIVALI, Itajaí, SC, Brazil Jose Angel Alvarez Perez & Rodrigo Sant’Ana Authors *
Jose Angel Alvarez Perez View author publications You can also search for this author inPubMed Google Scholar * Rodrigo Sant’Ana View author publications You can also search for this author
inPubMed Google Scholar CONTRIBUTIONS J.A.A.P. and R.S. contributed equally to the conception of this study and interpretation of the results. J.A.A.P. led the writing and preparation of the
submitted manuscript. R.S. led quantitative data processing and analysis. CORRESPONDING AUTHOR Correspondence to Jose Angel Alvarez Perez. ETHICS DECLARATIONS COMPETING INTERESTS The
authors declare no competing interests. PEER REVIEW PEER REVIEW INFORMATION _Communications Earth & Environment_ thanks Bárbara Franco, Julia Mason and the other, anonymous, reviewer(s)
for their contribution to the peer review of this work. Primary Handling Editors: Clare Davis. Peer reviewer reports are available. ADDITIONAL INFORMATION PUBLISHER’S NOTE Springer Nature
remains neutral with regard to jurisdictional claims in published maps and institutional affiliations. SUPPLEMENTARY INFORMATION SUPPLEMENTARY INFORMATION PEER REVIEW FILE REPORTING SUMMARY
RIGHTS AND PERMISSIONS OPEN ACCESS This article is licensed under a Creative Commons Attribution 4.0 International License, which permits use, sharing, adaptation, distribution and
reproduction in any medium or format, as long as you give appropriate credit to the original author(s) and the source, provide a link to the Creative Commons license, and indicate if changes
were made. The images or other third party material in this article are included in the article’s Creative Commons license, unless indicated otherwise in a credit line to the material. If
material is not included in the article’s Creative Commons license and your intended use is not permitted by statutory regulation or exceeds the permitted use, you will need to obtain
permission directly from the copyright holder. To view a copy of this license, visit http://creativecommons.org/licenses/by/4.0/. Reprints and permissions ABOUT THIS ARTICLE CITE THIS
ARTICLE Perez, J.A.A., Sant’Ana, R. Tropicalization of demersal megafauna in the western South Atlantic since 2013. _Commun Earth Environ_ 3, 227 (2022).
https://doi.org/10.1038/s43247-022-00553-z Download citation * Received: 18 April 2022 * Accepted: 09 September 2022 * Published: 04 October 2022 * DOI:
https://doi.org/10.1038/s43247-022-00553-z SHARE THIS ARTICLE Anyone you share the following link with will be able to read this content: Get shareable link Sorry, a shareable link is not
currently available for this article. Copy to clipboard Provided by the Springer Nature SharedIt content-sharing initiative