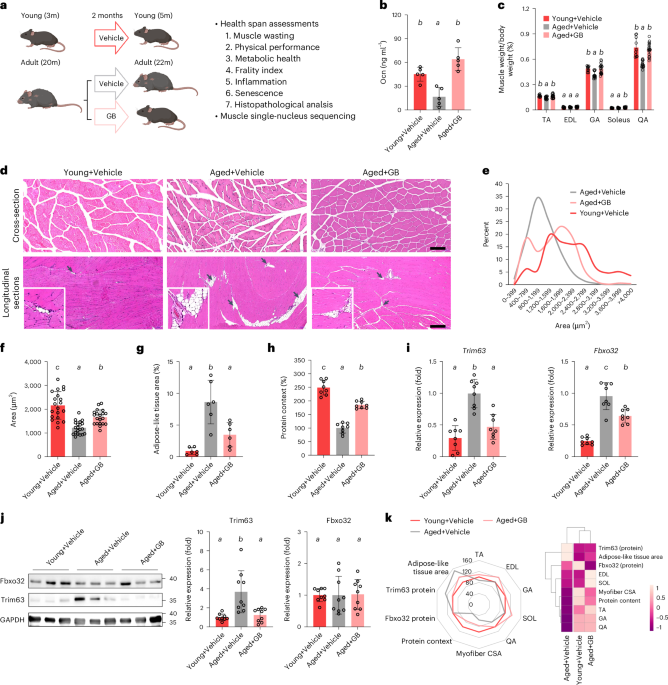
Ginkgolide b increases healthspan and lifespan of female mice
- Select a language for the TTS:
- UK English Female
- UK English Male
- US English Female
- US English Male
- Australian Female
- Australian Male
- Language selected: (auto detect) - EN
Play all audios:

ABSTRACT Various anti-aging interventions show promise in extending lifespan, but many are ineffective or even harmful to healthspan. Ginkgolide B (GB), derived from Ginkgo biloba, reduces
aging-related morbidities such as osteoporosis, yet its effects on healthspan and longevity have not been fully understood. In this study, we found that continuous oral administration of GB
to female mice beginning at 20 months of age extended median survival and median lifespan by 30% and 8.5%, respectively. GB treatment also decreased tumor incidence; enhanced muscle quality,
physical performance and metabolism; and reduced systemic inflammation and senescence. Single-nucleus RNA sequencing of skeletal muscle tissue showed that GB ameliorated aging-associated
changes in cell type composition, signaling pathways and intercellular communication. GB reduced aging-induced Runx1+ type 2B myonuclei through the upregulation of miR-27b-3p, which
suppresses Runx1 expression. Using functional analyses, we found that Runx1 promoted senescence and cell death in muscle cells. Collectively, these findings suggest the translational
potential of GB to extend healthspan and lifespan and to promote healthy aging. Access through your institution Buy or subscribe This is a preview of subscription content, access via your
institution ACCESS OPTIONS Access through your institution Access Nature and 54 other Nature Portfolio journals Get Nature+, our best-value online-access subscription $29.99 / 30 days cancel
any time Learn more Subscribe to this journal Receive 12 digital issues and online access to articles $119.00 per year only $9.92 per issue Learn more Buy this article * Purchase on
SpringerLink * Instant access to full article PDF Buy now Prices may be subject to local taxes which are calculated during checkout ADDITIONAL ACCESS OPTIONS: * Log in * Learn about
institutional subscriptions * Read our FAQs * Contact customer support SIMILAR CONTENT BEING VIEWED BY OTHERS TARGETING SENESCENCE INDUCED BY AGE OR CHEMOTHERAPY WITH A POLYPHENOL-RICH
NATURAL EXTRACT IMPROVES LONGEVITY AND HEALTHSPAN IN MICE Article Open access 01 July 2024 MITOCHONDRIAL AND METABOLIC DYSFUNCTION IN AGEING AND AGE-RELATED DISEASES Article 10 February 2022
DISTINCT AND ADDITIVE EFFECTS OF CALORIE RESTRICTION AND RAPAMYCIN IN AGING SKELETAL MUSCLE Article Open access 19 April 2022 DATA AVAILABILITY The datasets generated during the current
study are available in the Gene Expression Omnibus repository (GSE218027 and GSE254739), and all other data are available from the corresponding author upon reasonable request. REFERENCES *
Crimmins, E. M. Lifespan and healthspan: past, present, and promise. _Gerontologist_ 55, 901–911 (2015). PubMed PubMed Central Google Scholar * Partridge, L., Deelen, J. & Slagboom,
P. E. Facing up to the global challenges of ageing. _Nature_ 561, 45–56 (2018). CAS PubMed Google Scholar * Mercken, E. M., Carboneau, B. A., Krzysik-Walker, S. M. & de Cabo, R. Of
mice and men: the benefits of caloric restriction, exercise, and mimetics. _Ageing Res. Rev._ 11, 390–398 (2012). PubMed Google Scholar * Most, J., Tosti, V., Redman, L. M. & Fontana,
L. Calorie restriction in humans: an update. _Ageing Res. Rev._ 39, 36–45 (2017). PubMed Google Scholar * Mattson, M. P., Longo, V. D. & Harvie, M. Impact of intermittent fasting on
health and disease processes. _Ageing Res. Rev._ 39, 46–58 (2017). PubMed Google Scholar * Xie, K. et al. Every-other-day feeding extends lifespan but fails to delay many symptoms of aging
in mice. _Nat. Commun._ 8, 155 (2017). PubMed PubMed Central Google Scholar * Miller, R. A. et al. An Aging Interventions Testing Program: study design and interim report. _Aging Cell_
6, 565–575 (2007). CAS PubMed Google Scholar * Nadon, N. L. et al. Design of aging intervention studies: the NIA interventions testing program. _Age (Dordr.)_ 30, 187–199 (2008). CAS
PubMed Google Scholar * Zhang, Z. D. et al. Genetics of extreme human longevity to guide drug discovery for healthy ageing. _Nat. Metab._ 2, 663–672 (2020). PubMed PubMed Central Google
Scholar * Gomez-Linton, D. R. et al. Some naturally occurring compounds that increase longevity and stress resistance in model organisms of aging. _Biogerontology_ 20, 583–603 (2019).
PubMed Google Scholar * Argyropoulou, A., Aligiannis, N., Trougakos, I. P. & Skaltsounis, A. L. Natural compounds with anti-ageing activity. _Nat. Prod. Rep._ 30, 1412–1437 (2013). CAS
PubMed Google Scholar * Xu, Q. et al. The flavonoid procyanidin C1 has senotherapeutic activity and increases lifespan in mice. _Nat. Metab._ 3, 1706–1726 (2021). CAS PubMed PubMed
Central Google Scholar * Strong, R. et al. Evaluation of resveratrol, green tea extract, curcumin, oxaloacetic acid, and medium-chain triglyceride oil on life span of genetically
heterogeneous mice. _J. Gerontol. A Biol. Sci. Med. Sci._ 68, 6–16 (2013). CAS PubMed Google Scholar * Harrison, D. E. et al. Acarbose, 17-α-estradiol, and nordihydroguaiaretic acid
extend mouse lifespan preferentially in males. _Aging Cell_ 13, 273–282 (2014). CAS PubMed Google Scholar * Barzilai, N., Crandall, J. P., Kritchevsky, S. B. & Espeland, M. A.
Metformin as a tool to target aging. _Cell Metab._ 23, 1060–1065 (2016). CAS PubMed PubMed Central Google Scholar * Austad, S. N. Sex differences in health and aging: a dialog between
the brain and gonad? _Geroscience_ 41, 267–273 (2019). PubMed PubMed Central Google Scholar * Antoch, M. P. et al. Physiological frailty index (PFI): quantitative in-life estimate of
individual biological age in mice. _Aging (Albany NY)_ 9, 615–626 (2017). CAS PubMed Google Scholar * Lamming, D. W. et al. Rapamycin-induced insulin resistance is mediated by mTORC2 loss
and uncoupled from longevity. _Science_ 335, 1638–1643 (2012). CAS PubMed PubMed Central Google Scholar * Mannick, J. B. & Lamming, D. W. Targeting the biology of aging with mTOR
inhibitors. _Nat.Aging_ 3, 642–660 (2023). CAS PubMed PubMed Central Google Scholar * Liu, J. et al. Ginkgolide B inhibits hydrogen peroxideinduced apoptosis and attenuates cytotoxicity
via activating the PI3K/Akt/mTOR signaling pathway in H9c2 cells. _Mol. Med. Rep._ 22, 310–316 (2020). CAS PubMed PubMed Central Google Scholar * Wu, T. et al. Synergistic effects of
Ginkgolide B and protocatechuic acid on the treatment of Parkinson’s disease. _Molecules_ 25, 3976 (2020). * Yang, Y. et al. Study on the attenuated effect of Ginkgolide B on ferroptosis in
high fat diet induced nonalcoholic fatty liver disease. _Toxicology_ 445, 152599 (2020). CAS PubMed Google Scholar * Zhao, Y. et al. Polymeric nanoparticles-based brain delivery with
improved therapeutic efficacy of Ginkgolide B in Parkinson’s disease. _Int. J. Nanomedicine_ 15, 10453–10467 (2020). PubMed PubMed Central Google Scholar * Luo, L. et al. Ginkgolide B
lowers body weight and ameliorates hepatic steatosis in high-fat diet-induced obese mice correlated with pregnane X receptor activation. _RSC Adv._ 7, 37858–37866 (2017). CAS Google Scholar
* Zhu, B., Xue, F., Zhang, C. & Li, G. Ginkgolide B promotes osteoblast differentiation via activation of canonical Wnt signalling and alleviates osteoporosis through a bone anabolic
way. _J. Cell. Mol. Med._ 23, 5782–5793 (2019). CAS PubMed PubMed Central Google Scholar * Shu, Z. M. et al. Ginkgolide B protects against ischemic stroke via modulating microglia
polarization in mice. _CNS Neurosci. Ther._ 22, 729–739 (2016). CAS PubMed PubMed Central Google Scholar * Lee, C. W. et al. Ginkgolide B monotherapy reverses osteoporosis by regulating
oxidative stress-mediated bone homeostasis. _Free Radic. Biol. Med._ 168, 234–246 (2021). CAS PubMed Google Scholar * Wang, B. Y. H. et al. Ginkgolide B facilitates muscle regeneration
via rejuvenating osteocalcin‐mediated bone‐to‐muscle modulation in aged mice. _J. Cachexia Sarcopenia Muscle_ 14, 1349–1364 (2023). PubMed PubMed Central Google Scholar * Mera, P. et al.
Osteocalcin signaling in myofibers is necessary and sufficient for optimum adaptation to exercise. _Cell Metab._ 23, 1078–1092 (2016). CAS PubMed PubMed Central Google Scholar * Mera,
P., Laue, K., Wei, J., Berger, J. M. & Karsenty, G. Osteocalcin is necessary and sufficient to maintain muscle mass in older mice. _Mol. Metab._ 5, 1042–1047 (2016). CAS PubMed PubMed
Central Google Scholar * Bradburn, S. et al. Association between osteocalcin and cognitive performance in healthy older adults. _Age Ageing_ 45, 844–849 (2016). PubMed PubMed Central
Google Scholar * Liu, X. et al. Associations of osteocalcin forms with metabolic syndrome and its individual components in older men: the Health In Men Study. _J. Clin. Endocrinol. Metab._
106, e3506–e3518 (2021). PubMed Google Scholar * Shen, C. et al. A sensitive LC-MS/MS method to determine ginkgolide B in human plasma and urine: application in a pharmacokinetics and
excretion study of healthy Chinese subjects. _Xenobiotica_ 50, 323–331 (2020). CAS PubMed Google Scholar * Shao, F. et al. Pharmacokinetics of ginkgolides A, B and K after single and
multiple intravenous infusions and their interactions with midazolam in healthy Chinese male subjects. _Eur. J. Clin. Pharmacol._ 73, 537–546 (2017). CAS PubMed Google Scholar *
Cruz-Jentoft, A. J. & Sayer, A. A. Sarcopenia. _Lancet_ 393, 2636–2646 (2019). PubMed Google Scholar * Wang, S., Lai, X., Deng, Y. & Song, Y. Correlation between mouse age and
human age in anti-tumor research: significance and method establishment. _Life Sci._ 242, 117242 (2020). CAS PubMed Google Scholar * Lin, I. H. et al. Skeletal muscle in aged mice reveals
extensive transformation of muscle gene expression. _BMC Genet._ 19, 55 (2018). CAS PubMed PubMed Central Google Scholar * Goodpaster, B. H. et al. The loss of skeletal muscle strength,
mass, and quality in older adults: the Health, Aging and Body Composition Study. _J. Gerontol. A Biol. Sci. Med. Sci._ 61, 1059–1064 (2006). PubMed Google Scholar * Visser, M. et al.
Muscle mass, muscle strength, and muscle fat infiltration as predictors of incident mobility limitations in well-functioning older persons. _J. Gerontol. A Biol. Sci. Med. Sci._ 60, 324–333
(2005). PubMed Google Scholar * Avin, K. G. & Law, L. A. Age-related differences in muscle fatigue vary by contraction type: a meta-analysis. _Phys. Ther._ 91, 1153–1165 (2011). PubMed
PubMed Central Google Scholar * Purves-Smith, F. M., Sgarioto, N. & Hepple, R. T. Fiber typing in aging muscle. _Exerc. Sport Sci. Rev._ 42, 45–52 (2014). PubMed Google Scholar *
Ungvari, Z., Tarantini, S., Donato, A. J., Galvan, V. & Csiszar, A. Mechanisms of vascular aging. _Circ. Res._ 123, 849–867 (2018). CAS PubMed PubMed Central Google Scholar * Prior,
S. J. et al. Sarcopenia is associated with lower skeletal muscle capillarization and exercise capacity in older adults. _J. Gerontol. A Biol. Sci. Med. Sci._ 71, 1096–1101 (2016). PubMed
PubMed Central Google Scholar * Ichinose, E., Kurose, T., Daitoku, D. & Kawamata, S. The skeletal muscle vascular supply closely correlates with the muscle fiber surface area in the
rat. _Arch. Histol. Cytol._ 71, 45–57 (2008). PubMed Google Scholar * Wang, H. et al. Apoptosis in capillary endothelial cells in ageing skeletal muscle. _Aging Cell_ 13, 254–262 (2014).
CAS PubMed Google Scholar * Martin-Montalvo, A. et al. Metformin improves healthspan and lifespan in mice. _Nat. Commun._ 4, 2192 (2013). PubMed Google Scholar * Pearson, K. J. et al.
Resveratrol delays age-related deterioration and mimics transcriptional aspects of dietary restriction without extending life span. _Cell Metab._ 8, 157–168 (2008). CAS PubMed PubMed
Central Google Scholar * Neff, F. et al. Rapamycin extends murine lifespan but has limited effects on aging. _J. Clin. Invest._ 123, 3272–3291 (2013). CAS PubMed PubMed Central Google
Scholar * Srikanthan, P. & Karlamangla, A. S. Muscle mass index as a predictor of longevity in older adults. _Am. J. Med._ 127, 547–553 (2014). PubMed PubMed Central Google Scholar *
Alley, D. E. et al. Changes in weight at the end of life: characterizing weight loss by time to death in a cohort study of older men. _Am. J. Epidemiol._ 172, 558–565 (2010). PubMed PubMed
Central Google Scholar * Lundsgaard, A. M. & Kiens, B. Gender differences in skeletal muscle substrate metabolism—molecular mechanisms and insulin sensitivity. _Front. Endocrinol.
(Lausanne)_ 5, 195 (2014). PubMed Google Scholar * Tramunt, B. et al. Sex differences in metabolic regulation and diabetes susceptibility. _Diabetologia_ 63, 453–461 (2020). PubMed Google
Scholar * Whitehead, J. C. et al. A clinical frailty index in aging mice: comparisons with frailty index data in humans. _J. Gerontol. A Biol. Sci. Med. Sci._ 69, 621–632 (2014). PubMed
Google Scholar * Franceschi, C. et al. Inflamm-aging. An evolutionary perspective on immunosenescence. _Ann. N. Y. Acad. Sci._ 908, 244–254 (2000). CAS PubMed Google Scholar *
Franceschi, C. & Campisi, J. Chronic inflammation (inflammaging) and its potential contribution to age-associated diseases. _J. Gerontol. A Biol. Sci. Med. Sci._ 69, S4–S9 (2014). PubMed
Google Scholar * Wilson, D., Jackson, T., Sapey, E. & Lord, J. M. Frailty and sarcopenia: the potential role of an aged immune system. _Ageing Res. Rev._ 36, 1–10 (2017). PubMed
Google Scholar * Normand, R. et al. Found In Translation: a machine learning model for mouse-to-human inference. _Nat. Methods_ 15, 1067–1073 (2018). CAS PubMed Google Scholar * Di
Micco, R., Krizhanovsky, V., Baker, D. & d’Adda di Fagagna, F. Cellular senescence in ageing: from mechanisms to therapeutic opportunities. _Nat. Rev. Mol. Cell Biol._ 22, 75–95 (2021).
PubMed Google Scholar * Baker, D. J. et al. Clearance of p16Ink4a-positive senescent cells delays ageing-associated disorders. _Nature_ 479, 232–236 (2011). CAS PubMed PubMed Central
Google Scholar * Lei, Q. et al. Extracellular vesicles deposit PCNA to rejuvenate aged bone marrow-derived mesenchymal stem cells and slow age-related degeneration. _Sci. Transl. Med._ 13,
eaaz8697 (2021). * Kubben, N. et al. Repression of the antioxidant NRF2 pathway in premature aging. _Cell_ 165, 1361–1374 (2016). CAS PubMed PubMed Central Google Scholar * Shuai, Y. et
al. Melatonin treatment improves mesenchymal stem cells therapy by preserving stemness during long-term in vitro expansion. _Theranostics_ 6, 1899–1917 (2016). CAS PubMed PubMed Central
Google Scholar * Braun, F. et al. Altered lipid metabolism in the aging kidney identified by three layered omic analysis. _Aging (Albany NY)_ 8, 441–457 (2016). CAS PubMed Google Scholar
* Tietz, N. W., Shuey, D. F. & Wekstein, D. R. Laboratory values in fit aging individuals—sexagenarians through centenarians. _Clin. Chem._ 38, 1167–1185 (1992). CAS PubMed Google
Scholar * Murgia, M. et al. Single muscle fiber proteomics reveals fiber-type-specific features of human muscle aging. _Cell Rep._ 19, 2396–2409 (2017). CAS PubMed Google Scholar *
Petrany, M. J. et al. Single-nucleus RNA-seq identifies transcriptional heterogeneity in multinucleated skeletal myofibers. _Nat. Commun._ 11, 6374 (2020). CAS PubMed PubMed Central
Google Scholar * Kadi, F., Charifi, N., Denis, C. & Lexell, J. Satellite cells and myonuclei in young and elderly women and men. _Muscle Nerve_ 29, 120–127 (2004). PubMed Google
Scholar * McKellar, D. W. et al. Large-scale integration of single-cell transcriptomic data captures transitional progenitor states in mouse skeletal muscle regeneration. _Commun. Biol._ 4,
1280 (2021). CAS PubMed PubMed Central Google Scholar * Clemens, Z. et al. The biphasic and age-dependent impact of klotho on hallmarks of aging and skeletal muscle function. _eLife_
10, e61138 (2021). * Morabito, S., Reese, F., Rahimzadeh, N., Miyoshi, E. & Swarup, V. hdWGCNA identifies co-expression networks in high-dimensional transcriptomics data. _Cell Rep.
Methods_ 3, 100498 (2023). CAS PubMed PubMed Central Google Scholar * Drummond, M. J. et al. Aging and microRNA expression in human skeletal muscle: a microarray and bioinformatics
analysis. _Physiol. Genomics_ 43, 595–603 (2011). CAS PubMed Google Scholar * Pardo, P. S., Hajira, A., Boriek, A. M. & Mohamed, J. S. MicroRNA-434-3p regulates age-related apoptosis
through eIF5A1 in the skeletal muscle. _Aging (Albany NY)_ 9, 1012–1029 (2017). CAS PubMed Google Scholar * Yang, X. et al. miR-27b-3p attenuates muscle atrophy by targeting Cbl-b in
skeletal muscles. _Biomolecules_ 12, 191 (2022). * Li, H. et al. MiR-27b augments bone marrow progenitor cell survival via suppressing the mitochondrial apoptotic pathway in type 2 diabetes.
_Am. J. Physiol. Endocrinol. Metab._ 313, E391–E401 (2017). CAS PubMed PubMed Central Google Scholar * Yuan, R. et al. Aging in inbred strains of mice: study design and interim report
on median lifespans and circulating IGF1 levels. _Aging Cell_ 8, 277–287 (2009). CAS PubMed Google Scholar * Asadi Shahmirzadi, A. et al. Alpha-ketoglutarate, an endogenous metabolite,
extends lifespan and compresses morbidity in aging mice. _Cell Metab._ 32, 447–456 (2020). CAS PubMed PubMed Central Google Scholar * Chen, Y. F. et al. Cisd2 deficiency drives premature
aging and causes mitochondria-mediated defects in mice. _Genes Dev._ 23, 1183–1194 (2009). CAS PubMed PubMed Central Google Scholar * Barardo, D. et al. The DrugAge database of
aging-related drugs. _Aging Cell_ 16, 594–597 (2017). CAS PubMed PubMed Central Google Scholar * Moskalev, A. et al. Geroprotectors.org: a new, structured and curated database of current
therapeutic interventions in aging and age-related disease. _Aging (Albany NY)_ 7, 616–628 (2015). CAS PubMed Google Scholar * Zhang, X. et al. Characterization of cellular senescence in
aging skeletal muscle. _Nat. Aging_ 2, 601–615 (2022). CAS PubMed PubMed Central Google Scholar * Kang, M. J. et al. Metformin induces muscle atrophy by transcriptional regulation of
myostatin via HDAC6 and FoxO3a. _J. Cachexia Sarcopenia Muscle_ 13, 605–620 (2022). PubMed Google Scholar * McLeod, M., Breen, L., Hamilton, D. L. & Philp, A. Live strong and prosper:
the importance of skeletal muscle strength for healthy ageing. _Biogerontology_ 17, 497–510 (2016). PubMed PubMed Central Google Scholar * Gilbert, W. et al. Stromal cell-derived factor-1
(CXCL12) and its role in bone and muscle biology. _Cytokine_ 123, 154783 (2019). CAS PubMed PubMed Central Google Scholar * Zembron-Lacny, A., Dziubek, W., Wolny-Rokicka, E., Dabrowska,
G. & Wozniewski, M. The relation of inflammaging with skeletal muscle properties in elderly men. _Am. J. Mens Health_ 13, 1557988319841934 (2019). PubMed PubMed Central Google Scholar
* Kraemer, W. J. et al. Influence of HMB supplementation and resistance training on cytokine responses to resistance exercise. _J. Am. Coll. Nutr._ 33, 247–255 (2014). CAS PubMed Google
Scholar * Li, H. et al. Muscle-secreted granulocyte colony-stimulating factor functions as metabolic niche factor ameliorating loss of muscle stem cells in aged mice. _EMBO J._ 38, e102154
(2019). CAS PubMed PubMed Central Google Scholar * Minamino, K. et al. Macrophage colony-stimulating factor (M-CSF), as well as granulocyte colony-stimulating factor (G-CSF), accelerates
neovascularization. _Stem Cells_ 23, 347–354 (2005). CAS PubMed Google Scholar * McKay, B. R. et al. Association of interleukin-6 signalling with the muscle stem cell response following
muscle-lengthening contractions in humans. _PLoS ONE_ 4, e6027 (2009). PubMed PubMed Central Google Scholar * Toth, K. G. et al. IL-6 induced STAT3 signalling is associated with the
proliferation of human muscle satellite cells following acute muscle damage. _PLoS ONE_ 6, e17392 (2011). CAS PubMed PubMed Central Google Scholar * Begue, G. et al. Early activation of
rat skeletal muscle IL-6/STAT1/STAT3 dependent gene expression in resistance exercise linked to hypertrophy. _PLoS ONE_ 8, e57141 (2013). CAS PubMed PubMed Central Google Scholar *
Serrano, A. L., Baeza-Raja, B., Perdiguero, E., Jardi, M. & Munoz-Canoves, P. Interleukin-6 is an essential regulator of satellite cell-mediated skeletal muscle hypertrophy. _Cell
Metab._ 7, 33–44 (2008). CAS PubMed Google Scholar * Chowdhury, S. et al. Muscle-derived interleukin 6 increases exercise capacity by signaling in osteoblasts. _J. Clin. Invest._ 130,
2888–2902 (2020). CAS PubMed PubMed Central Google Scholar * Kim, M. et al. Single-nucleus transcriptomics reveals functional compartmentalization in syncytial skeletal muscle cells.
_Nat. Commun._ 11, 6375 (2020). CAS PubMed PubMed Central Google Scholar * Dos Santos, M. et al. Single-nucleus RNA-seq and FISH identify coordinated transcriptional activity in
mammalian myofibers. _Nat. Commun._ 11, 5102 (2020). PubMed PubMed Central Google Scholar * Yang, X. et al. Changes of gene expression patterns of muscle pathophysiology-related
transcription factors during denervated muscle atrophy. _Front. Physiol._ 13, 923190 (2022). PubMed PubMed Central Google Scholar * Hunt, E. R. et al. Temporal disruption of neuromuscular
communication and muscle atrophy following noninvasive ACL injury in rats. _J. Appl. Physiol. (1985)_ 132, 46–57 (2022). CAS PubMed Google Scholar * Lin, H. et al. Decoding the
transcriptome of denervated muscle at single-nucleus resolution. _J. Cachexia Sarcopenia Muscle_ 13, 2102–2117 (2022). PubMed PubMed Central Google Scholar * Umansky, K. B. et al. Runx1
transcription factor is required for myoblasts proliferation during muscle regeneration. _PLoS Genet._ 11, e1005457 (2015). PubMed PubMed Central Google Scholar * Wildey, G. M. &
Howe, P. H. Runx1 is a co-activator with FOXO3 to mediate transforming growth factor beta (TGFβ)-induced Bim transcription in hepatic cells. _J. Biol. Chem._ 284, 20227–20239 (2009). CAS
PubMed PubMed Central Google Scholar * Kang, Y. J., Yoo, J. I. & Baek, K. W. Differential gene expression profile by RNA sequencing study of elderly osteoporotic hip fracture patients
with sarcopenia. _J. Orthop. Translat._ 29, 10–18 (2021). PubMed PubMed Central Google Scholar * Aging Atlas, C. Aging Atlas: a multi-omics database for aging biology. _Nucleic Acids
Res._ 49, D825–D830 (2021). Google Scholar * Casella, G. et al. Transcriptome signature of cellular senescence. _Nucleic Acids Res._ 47, 7294–7305 (2019). CAS PubMed PubMed Central
Google Scholar * Duan, S. et al. PTEN deficiency reprogrammes human neural stem cells towards a glioblastoma stem cell-like phenotype. _Nat. Commun._ 6, 10068 (2015). CAS PubMed Google
Scholar * Wang, W. et al. A genome-wide CRISPR-based screen identifies _KAT7_ as a driver of cellular senescence. _Sci. Transl. Med._ 13, eabd2655 (2021). * Ma, S. et al. Caloric
restriction reprograms the single-cell transcriptional landscape of _Rattus norvegicus_ aging. _Cell_ 180, 984–1001 (2020). CAS PubMed Google Scholar * Wotton, S. F. et al. RUNX1
transformation of primary embryonic fibroblasts is revealed in the absence of p53. _Oncogene_ 23, 5476–5486 (2004). CAS PubMed Google Scholar * Wolyniec, K. et al. RUNX1 and its fusion
oncoprotein derivative, RUNX1-ETO, induce senescence-like growth arrest independently of replicative stress. _Oncogene_ 28, 2502–2512 (2009). CAS PubMed PubMed Central Google Scholar *
Cai, X. et al. Runx1 deficiency decreases ribosome biogenesis and confers stress resistance to hematopoietic stem and progenitor cells. _Cell Stem Cell_ 17, 165–177 (2015). CAS PubMed
PubMed Central Google Scholar * Wu, D., Ozaki, T., Yoshihara, Y., Kubo, N. & Nakagawara, A. Runt-related transcription factor 1 (RUNX1) stimulates tumor suppressor p53 protein in
response to DNA damage through complex formation and acetylation. _J. Biol. Chem._ 288, 1353–1364 (2013). CAS PubMed Google Scholar * Geng, L. et al. Low-dose quercetin positively
regulates mouse healthspan. _Protein Cell_ 10, 770–775 (2019). PubMed PubMed Central Google Scholar * Messi, M. L. et al. Resistance training enhances skeletal muscle innervation without
modifying the number of satellite cells or their myofiber association in obese older adults. _J. Gerontol. A Biol. Sci. Med. Sci._ 71, 1273–1280 (2016). PubMed Google Scholar * Mitchell,
S. J. et al. Effects of sex, strain, and energy intake on hallmarks of aging in mice. _Cell Metab._ 23, 1093–1112 (2016). CAS PubMed PubMed Central Google Scholar * Richardson, N. E. et
al. Lifelong restriction of dietary branched-chain amino acids has sex-specific benefits for frailty and lifespan in mice. _Nat. Aging_ 1, 73–86 (2021). PubMed PubMed Central Google
Scholar * Miller, R. A. et al. Methionine-deficient diet extends mouse lifespan, slows immune and lens aging, alters glucose, T4, IGF-I and insulin levels, and increases hepatocyte MIF
levels and stress resistance. _Aging Cell_ 4, 119–125 (2005). CAS PubMed Google Scholar * Salvestrini, V., Sell, C. & Lorenzini, A. Obesity may accelerate the aging process. _Front.
Endocrinol. (Lausanne)_ 10, 266 (2019). PubMed Google Scholar * Hussain, S. M. et al. Associations of change in body size with all-cause and cause-specific mortality among healthy older
adults. _JAMA Netw. Open_ 6, e237482 (2023). PubMed PubMed Central Google Scholar * Cheng, F. W. et al. Body mass index and all-cause mortality among older adults. _Obesity (Silver
Spring)_ 24, 2232–2239 (2016). PubMed Google Scholar * Leong, D. P. et al. Prognostic value of grip strength: findings from the Prospective Urban Rural Epidemiology (PURE) study. _Lancet_
386, 266–273 (2015). PubMed Google Scholar * Group, S. R. et al. A randomized trial of intensive versus standard blood-pressure control. _N. Engl. J. Med._ 373, 2103–2116 (2015). Google
Scholar * Alharbi, T. A. et al. Self-reported early and later life weight and the risk of all-cause mortality in older adults. _J. Nutr. Health Aging_ 27, 301–308 (2023). CAS PubMed
PubMed Central Google Scholar * Lv, Z. et al. Ginkgolide B treatment regulated intestinal flora to improve high-fat diet induced atherosclerosis in ApoE−/− mice. _Biomed. Pharmacother._
134, 111100 (2021). CAS PubMed Google Scholar * Kane, A. E., Sinclair, D. A., Mitchell, J. R. & Mitchell, S. J. Sex differences in the response to dietary restriction in rodents.
_Curr. Opin. Physiol._ 6, 28–34 (2018). PubMed PubMed Central Google Scholar * Horstman, A. M., Dillon, E. L., Urban, R. J. & Sheffield-Moore, M. The role of androgens and estrogens
on healthy aging and longevity. _J. Gerontol. A Biol. Sci. Med. Sci._ 67, 1140–1152 (2012). PubMed PubMed Central Google Scholar * Yucel, A. D. & Gladyshev, V. N. The long and winding
road of reprogramming-induced rejuvenation. _Nat. Commun._ 15, 1941 (2024). CAS PubMed PubMed Central Google Scholar * Das, N. M. et al. In vivo quantitative microcomputed tomographic
analysis of vasculature and organs in a normal and diseased mouse model. _PLoS ONE_ 11, e0150085 (2016). PubMed PubMed Central Google Scholar * Metsalu, T. & Vilo, J. ClustVis: a web
tool for visualizing clustering of multivariate data using Principal Component Analysis and heatmap. _Nucleic Acids Res._ 43, W566–W570 (2015). CAS PubMed PubMed Central Google Scholar *
Pang, Z. et al. MetaboAnalyst 5.0: narrowing the gap between raw spectra and functional insights. _Nucleic Acids Res._ 49, W388–W396 (2021). CAS PubMed PubMed Central Google Scholar *
Lee, C.-W. et al. Demystifying the long noncoding RNA landscape of small EVs derived from human mesenchymal stromal cells. _J. Adv. Res._ 39, 73–88 (2021). PubMed PubMed Central Google
Scholar * Chen, W. J. et al. Ribonucleotide reductase M2B in the myofibers modulates stem cell fate in skeletal muscle. _NPJ Regen. Med._ 7, 37 (2022). PubMed PubMed Central Google
Scholar * Hao, Y. et al. Integrated analysis of multimodal single-cell data. _Cell_ 184, 3573–3587 (2021). CAS PubMed PubMed Central Google Scholar * Young, M. D. & Behjati, S.
SoupX removes ambient RNA contamination from droplet-based single-cell RNA sequencing data. _Gigascience_ 9, giaa151 (2020). * Barron, M. & Li, J. Identifying and removing the cell-cycle
effect from single-cell RNA-Sequencing data. _Sci Rep._ 6, 33892 (2016). CAS PubMed PubMed Central Google Scholar * Wu, T. et al. clusterProfiler 4.0: a universal enrichment tool for
interpreting omics data. _Innovation (Camb.)_ 2, 100141 (2021). CAS PubMed Google Scholar * Jin, S. et al. Inference and analysis of cell–cell communication using CellChat. _Nat. Commun._
12, 1088 (2021). CAS PubMed PubMed Central Google Scholar * Langfelder, P. & Horvath, S. WGCNA: an R package for weighted correlation network analysis. _BMC Bioinformatics_ 9, 559
(2008). PubMed PubMed Central Google Scholar * Andrews, S. FastQC. Babraham Bioinformatics. https://www.bioinformatics.babraham.ac.uk/projects/fastqc/ (2010). * Chen, S., Zhou, Y., Chen,
Y. & Gu, J. fastp: an ultra-fast all-in-one FASTQ preprocessor. _Bioinformatics_ 34, i884–i890 (2018). PubMed PubMed Central Google Scholar * Kim, D., Paggi, J. M., Park, C., Bennett,
C. & Salzberg, S. L. Graph-based genome alignment and genotyping with HISAT2 and HISAT-genotype. _Nat. Biotechnol._ 37, 907–915 (2019). CAS PubMed PubMed Central Google Scholar *
Danecek, P. et al. Twelve years of SAMtools and BCFtools. _Gigascience_ 10, giab008 (2021). * Liao, Y., Smyth, G. K. & Shi, W. featureCounts: an efficient general purpose program for
assigning sequence reads to genomic features. _Bioinformatics_ 30, 923–930 (2014). CAS PubMed Google Scholar * Love, M. I., Huber, W. & Anders, S. Moderated estimation of fold change
and dispersion for RNA-seq data with DESeq2. _Genome Biol._ 15, 550 (2014). PubMed PubMed Central Google Scholar * RStudio Team. RStudio: Integrated Development for R.
http://www.rstudio.com (2020). * Wickham, H. _ggplot2: Elegant Graphics for Data Analysis_ (Springer, 2016). * Gu, Z., Eils, R. & Schlesner, M. Complex heatmaps reveal patterns and
correlations in multidimensional genomic data. _Bioinformatics_ 32, 2847–2849 (2016). CAS PubMed Google Scholar Download references ACKNOWLEDGEMENTS This work was supported by the
National Science and Technology Council, Taiwan (111-2320-B-039-070-MY3 to C.-W.L.) and China Medical University Hospital (EXO-113-004, DMR-112-147 and DMR-113-106 to C.-W.L.). Experiments
and data analysis were performed in part through the use of the Medical Research Core Facilities Center, Office of Research & Development, at China Medical University. We thank the RNA
Technology Platform and Gene Manipulation Core Facility (RNAi core) of the National Core Facility for Biopharmaceuticals at Academia Sinica in Taiwan for providing reagents and related
services. Illustrations were created in BioRender: Lee, C. (2024) https://BioRender.com/j81t493 and Lee, C. (2020) https://BioRender.com/k43w200. AUTHOR INFORMATION Author notes * These
authors jointly supervised this work: Chien-Wei Lee, Oscar Kuang-Sheng Lee. AUTHORS AND AFFILIATIONS * Translational Cell Therapy Center, China Medical University Hospital, Taichung, Taiwan
Chien-Wei Lee, Yu-Fan Chen, Hao-Hsiang Wu, Po-Yu Cheng & Zong-Han Chou * Department of Biomedical Engineering, China Medical University, Taichung, Taiwan Chien-Wei Lee & Yu-Fan Chen
* Center for Neuromusculoskeletal Restorative Medicine, CUHK InnoHK Centres, Hong Kong Science Park, Hong Kong, China Belle Yu-Hsuan Wang & Wayne Yuk-Wai Lee * Li Ka Shing Institute of
Health Sciences, The Chinese University of Hong Kong, Hong Kong, China Belle Yu-Hsuan Wang, Allen Wei-Ting Hsiao & Wayne Yuk-Wai Lee * School of Biomedical Sciences, Faculty of Medicine,
The Chinese University of Hong Kong, Hong Kong, China Shing Hei Wong, Qin Cao, Sin-Hang Fung & Stephen Kwok Wing Tsui * Ph.D. Program for Translational Medicine, College of Medical
Science and Technology, Taipei Medical University, Taipei, Taiwan Yi-Fan Chen * Graduate Institute of Translational Medicine, College of Medical Science and Technology, Taipei Medical
University, Taipei, Taiwan Yi-Fan Chen * International Ph.D. Program for Translational Science, College of Medical Science and Technology, Taipei Medical University, Taipei, Taiwan Yi-Fan
Chen * Master Program in Clinical Genomics and Proteomics, School of Pharmacy, Taipei Medical University, Taipei, Taiwan Yi-Fan Chen * Department of Orthopaedics and Traumatology, The
Chinese University of Hong Kong, Hong Kong, China Allen Wei-Ting Hsiao & Wayne Yuk-Wai Lee * SH Ho Scoliosis Research Laboratory, Joint Scoliosis Research Centre of the Chinese
University of Hong Kong and Nanjing University, The Chinese University of Hong Kong, Hong Kong, China Wayne Yuk-Wai Lee * Department of Biotechnology Medicine, MacKay Memorial Hospital,
Taipei, Taiwan Oscar Kuang-Sheng Lee Authors * Chien-Wei Lee View author publications You can also search for this author inPubMed Google Scholar * Belle Yu-Hsuan Wang View author
publications You can also search for this author inPubMed Google Scholar * Shing Hei Wong View author publications You can also search for this author inPubMed Google Scholar * Yi-Fan Chen
View author publications You can also search for this author inPubMed Google Scholar * Qin Cao View author publications You can also search for this author inPubMed Google Scholar * Allen
Wei-Ting Hsiao View author publications You can also search for this author inPubMed Google Scholar * Sin-Hang Fung View author publications You can also search for this author inPubMed
Google Scholar * Yu-Fan Chen View author publications You can also search for this author inPubMed Google Scholar * Hao-Hsiang Wu View author publications You can also search for this author
inPubMed Google Scholar * Po-Yu Cheng View author publications You can also search for this author inPubMed Google Scholar * Zong-Han Chou View author publications You can also search for
this author inPubMed Google Scholar * Wayne Yuk-Wai Lee View author publications You can also search for this author inPubMed Google Scholar * Stephen Kwok Wing Tsui View author publications
You can also search for this author inPubMed Google Scholar * Oscar Kuang-Sheng Lee View author publications You can also search for this author inPubMed Google Scholar CONTRIBUTIONS
C.-W.L. designed the study, performed the experiments and data analysis, wrote the manuscript, provided funding and supervised the study. B.Y.-H.W. performed and analyzed data of in vivo
experiments. S.H.W. and S.-H.F. performed single-nucleus RNA sequencing analysis. A.W.-T.H. performed data analyses of in vitro experiments. Y.-F.C. provided critical technical support and
feedback regarding the manuscript. Q.C. offered technical support for single-nucleus RNA sequencing analysis and manuscript editing. P.-Y.C. and Z.-H.C. performed and analyzed data of in
vitro experiments and bulk RNA sequencing. H.-H.W., Y.-F.C., W.Y.-W.L. and S.K.W.T. provided input for the manuscript. O.K.-S.L. supervised the study. All authors read and approved the final
manuscript. CORRESPONDING AUTHORS Correspondence to Chien-Wei Lee or Oscar Kuang-Sheng Lee. ETHICS DECLARATIONS COMPETING INTERESTS The authors disclose that a patent application related to
the findings presented in this paper has been submitted. The details of the patent are as follows: Patent Applicant: China Medical University; Inventors: O.K.-S.L., C.-W.L., Y.-F.C. and
H.-H.W.; Application Numbers: US14307 and 112144291; Status: Pending. The other authors declare no conflicts of interest. PEER REVIEW PEER REVIEW INFORMATION _Nature Aging_ thanks the
anonymous reviewer(s) for their contribution to the peer review of this work. ADDITIONAL INFORMATION PUBLISHER’S NOTE Springer Nature remains neutral with regard to jurisdictional claims in
published maps and institutional affiliations. EXTENDED DATA EXTENDED DATA FIG. 1 GB INCREASES MYOFIBRE SIZE AND GRIP STRENGTH IN SIX-MONTH-OLD MICE. (A) Experimental design and GB
structure: C57BL/6 female mice (six-month-old) received GB or vehicle by oral gavage for two months (6 m+Vehicle, n = 4; 6 m+GB, n = 6). Illustration was created in BioRender.com. (B)
Expression of circulating osteocalcin after GB administration for two months. (C) Representative haematoxylin and eosin staining (H&E) cross-section images of the tibialis anterior.
Scale bar, 50 μm. (D–E) Quantification and distribution of tibialis anterior (D) and soleus (E) myofibre cross-sectional area. Results are measured from 11 images from four mice for the
vehicle group and 15 images from six mice for the GB group. (F) Forelimb grip strength assay. Quantitative data are presented as the means ± SD in the histogram with data points. Statistical
analyses are performed using two-tailed Student’s t-test, with significance set at p < 0.05. (*p < 0.05; **p < 0.01; ***p < 0.001). Source data EXTENDED DATA FIG. 2 GB
ATTENUATES MUSCLE WASTING AND IMPROVES PHYSICAL ACTIVITY IN POSTMENOPAUSAL MICE. (A) Experimental design (n = 10). After OVX for one month, OVX mice received two doses of GB daily (3 and 12
mg/kg body weight) for 2 months. Illustration was created in BioRender.com. (B) Quadriceps weight-to-body weight. (C) Representative H&E staining of quadriceps. (D–E) Quantification (D)
and distribution (E) of the myofibre cross-sectional area are measured from 30 images of 10 mice. Scale bar, 100 μm. (F–G) Healthspan was measured by grip strength (F), rotarod (G), and
balance beam test (H) (n = 5). Quantitative data are presented as the means ± SD in the histogram with the data point. Statistical analyses are performed using one-way ANOVA with Tukey’s
multiple comparison test. Means not sharing any letters are significantly different (p < 0.05). Source data EXTENDED DATA FIG. 3 GINKGOLIDE B REPRESSES H2O2-INDUCED SENESCENCE IN MUSCS.
(A) Experimental design and senescence-related assessments on MuSCs. Senescence was induced by H2O2 stimulation for 2 hours in MuSCs. Following a PBS wash, the senescent C2C12 were treated
with or without GB (5 mg/L). Sen, H2O2-induced senescence. PAX7 positive cells represent MuSCs. Scale bar, 50 μm. (B-C) Representative images (B) and quantification (C) of P21 and γ-H2AX
staining (n = 5). Scale bar, 50 μm. (D) Representative images and proportion of SA-β-gal positive cells (n = 3). Scale bar, 50 μm. Quantitative data are presented as the means ± SD in the
histogram with the data point. Statistical analyses are performed using one-way ANOVA with Tukey’s multiple comparison test. This means not share any letter are significantly different (p
< 0.05). Source data EXTENDED DATA FIG. 4 THE EFFECT OF GB ON MULTIPLE ORGANS OF AGED MICE. (A) Organ weight-to-body weight in aged mice after GB administration for two months. (B)
H&E staining of the kidney, heart, spleen, and liver. Scale bar, 100 μm for kidney, 50 μm for heart, 400 μm for spleen, and 200 μm for liver. (C) Glomerular size (n = 5). (D)
Quantification of the cardiac myofibre cross-sectional area (n = 5). (E) Quantification of the white pulp area (n = 32-35 white pulps from 5 individual mice per group) and white pulp/red
pulp ratio of spleen (n = 5). (F) Quantification of hepatic microgranulomas (indicated by arrows) (n = 10 images from 5 individual mice per group). (G) Nile red staining reveals hepatic
lipid accumulation in aged mice (n = 5). Liver section without Nile red staining served as a negative control. Scale bar, 50 μm. (H) Masson’s trichrome staining of the kidney, heart, spleen,
and liver. Scale bar, 50 μm for kidney, 100 μm for heart, 100 μm for spleen, and 200 μm for liver. (I–L) Quantification of Masson’s trichrome staining in the kidney (I), heart (J), spleen
(K), and liver (L) (n = 5). Quantitative data are presented as the means ± SD in the histogram with data point. Statistical analyses are performed using one-way ANOVA with Tukey’s multiple
comparison test. Means not sharing any letter are significantly different (p < 0.05). Source data EXTENDED DATA FIG. 5 SINGLE-NUCLEUS LANDSCAPE OF SKELETAL MUSCLES. (A) Graphical scheme
of the experimental design. Illustration was created in BioRender.com. (B) Isolated nuclei with DAPI staining (n = 3). Scale bar, 20 μm and 5 μm from top to bottom panels. (C–D) UMAP diagram
from integrated datasets (C) and separated datasets (D) revealed the coordinates of nuclear types in GA muscle of Young+Vehicle, Aged+Vehicle, and Aged+GB groups. Type 2B myonucleus-1, Type
2B-1; Type 2B myonucleus-2, Type 2B-2; Type 2X myonucleus, Type 2X; Type 2 A myonucleus, Type 2A; myotendinous Junction, MTJ; fibro/adipogenic progenitors, FAPs; endothelial cells, EC;
pericytes, Peri; satellite cells, SatC; adipocytes, Adip; tenocytes, Teno; immune cells, IC; smooth muscles SM; Schwann cells, SchwC. (E) Venn diagrams illustrating the reversion of nuclear
types by GB administration. Percentage change in proportion over 10% was considered significant. (F) Rose diagrams revealed the numbers of aging DEGs, GB DEGs, and Rescue DEGs of various
cell types in skeletal muscles (DEG, FDR < 0.05, absolute log2FC > 0.25). (G) Rescue DEGs from various cell types and representative GO pathways in skeletal muscles. EXTENDED DATA FIG.
6 SINGLE-NUCLEUS RNA SEQUENCING ANALYSIS OF MYONUCLEI. (A–B) Volcano plot (A) and heatmap (B) of the pseudo-bulk DEGs between Aged+Vehicle vs Young+Vehicle and Aged+GB vs Aged+Vehicle
groups. Log2 fold-change (log2 FC) > 1 and an adj. p < 0.05 are used to identify DEGs. (C) Venn diagrams illustrating convergence and noncongruence of pseudo-bulk DEGs between groups.
(D) Pseudo-bulk GSEA analysis of myonuclei between Young+Vehicle vs Aged+Vehicle and Aged+GB vs Aged+Vehicle datasets. The similar pattern between the two datasets indicates GB reversed
aging-related changes in the indicative transcriptome. Gene rank metric = sign(log2FC) x –log10(adj. p-val). (E) Top 10 specific DEGs in Runx1+ myonucleus. (F) GO enrichment analysis by
biological process of top 30 specific genes in Runx1+ type2B myonuclei from integrated dataset. (G) hdWGCNA dendrograms identified 31 co-expression modules. (H) Visualization of module
eigengene value over myonucleus. (I) Visualization of turquoise module eigengene value over myonucleus. Left, all genes with turquoise module; right, top 25 hub genes within turquoise
module. (J) GO analysis of DEGs within turquoise module. (K) Volcano plot of the DEGs in Runx1+ type2B myonuclei between Aged+Vehicle vs Young+Vehicle and Aged+GB vs Aged+Vehicle groups.
Reported p-values are adjusted (p-adj) to account for multiple comparisons. EXTENDED DATA FIG. 7 EFFECT OF RUNX1 OVEREXPRESSION AND KNOCKDOWN IN C2C12. (A) The chronological series of
assessment time slots. (B) Evaluation of transfection efficiency at 24 hours post-transfection, as determined by the proportion of GFP positive C2C12 (n = 5). Control-GFP, cells transfected
with pcDNA3.1( + )-GFP. (C-D) Representative flow cytometry plot (C) and quantitation (D) of Annexin V and PI staining in C2C12 (n = 3). Control-GFP, cells transfected with pcDNA3.1(+);
Runx1 OE, cells transfected with pcDNA3.1(+)-Runx1 for 24 and 48 hours; H2O2, cells exposed to 1.5 mM H2O2 for 6 hours. (E) Detection of senescence markers by real-time PCR in Runx1
knockdown C2C12 (n = 4). (F-I) Cellular morphology, cell size (F), doubling time (G) γ-H2AX staining (H) and senescence markers (I) of Runx1 knockdown C2C12 under H2O2 stimulation (n = 5-6).
Doubling time = Duration x ln(2) / ln(Final concentration/ Initial concentration). Quantitative data are presented as the means ± SD in the histogram with the data point. Statistical
analyses are performed using two-way ANOVA or two-tailed Student’s t-test (*P < 0.05; **P < 0.01; ***P < 0.001), depending on experiment design. Source data EXTENDED DATA FIG. 8
MIR-27B-3P MEDIATES THE EFFECT OF GB ON RUNX1 AND SENESCENCE. (A) Veen diagram revealed the prediction of potential Runx1 targeting human and mouse miRNAs. (B) Expression of miR-27b-3p in
aged muscle (n = 6). (C) Expression of miR-27b-3p in H2O2-induced senescent C2C12 (n = 5). Sen, H2O2-induced senescence. (D) Correlation comparisons of transcript levels of miR-27b-3p with
transcript of Runx1, IL-6 and SA-β-gal activity (n = 5). Correlations are measured by Pearson coefficient (R) with corresponding 95% confidence intervals and assessed using two-tailed
p-value analysis. (E) Expression of miR-27b-3p and Runx1 in H2O2-induced senescent C2C12 with GB and miR-27b-3p antagonist treatment (n = 3). (F) Cell viability (n = 6). (G) Representative
morphology and cell size (n = 5). Scale bar, 100 μm. (H) Representative images and quantification of SA-β-gal staining (n = 5). Scale bar, 50 μm. (I) Representative images and quantification
of ϒ-H2AX staining (n = 5). Scale bar, 50 μm. (J) Potential mechanism of action of GB. Source data EXTENDED DATA FIG. 9 SINGLE-NUCLEUS RNA SEQUENCING ANALYSIS REVEALS THE CHANGES IN
CELL-CELL COMMUNICATION. (A–B) Circle plots (A) and heatmap (B) showed the differential cell-cell communication between Young+Vehicle vs Aged+Vehicle and Aged+GB vs Aged+Vehicle datasets in
skeletal muscle. The similar signaling pattern between the two datasets indicates GB reversed aging-related changes in cell-cell communication. (C) Plot revealed the contribution of outgoing
and incoming signalling between various cells. Circle size represents the counts in each nucleus type. (D) Chord diagram showing interaction count and weight of cell-to-muscle
communication. The thickness of the line represents interaction count and weight of ligand-receptor interaction. (E) Differential cell-to-muscle signaling. SUPPLEMENTARY INFORMATION
SUPPLEMENTARY INFORMATION Supplementary Figs. 1–11. REPORTING SUMMARY SUPPLEMENTARY TABLE Supplementary Tables 1–11. SOURCE DATA SOURCE DATA FIG. 1 Statistical source data. SOURCE DATA FIG.
2 Statistical source data. SOURCE DATA FIG. 3 Statistical source data. SOURCE DATA FIG. 4 Statistical source data. SOURCE DATA FIG. 5 Statistical source data. SOURCE DATA FIG. 7 Statistical
source data. SOURCE DATA FIG. 8 Statistical source data. SOURCE DATA WB Unprocessed western blots. SOURCE DATA EXTENDED DATA FIG. 1 Statistical source data. SOURCE DATA EXTENDED DATA FIG. 2
Statistical source data. SOURCE DATA EXTENDED DATA FIG. 3 Statistical source data. SOURCE DATA EXTENDED DATA FIG. 4 Statistical source data. SOURCE DATA EXTENDED DATA FIG. 7 Statistical
source data. SOURCE DATA EXTENDED DATA FIG. 8 Statistical source data. RIGHTS AND PERMISSIONS Springer Nature or its licensor (e.g. a society or other partner) holds exclusive rights to this
article under a publishing agreement with the author(s) or other rightsholder(s); author self-archiving of the accepted manuscript version of this article is solely governed by the terms of
such publishing agreement and applicable law. Reprints and permissions ABOUT THIS ARTICLE CITE THIS ARTICLE Lee, CW., Wang, B.YH., Wong, S.H. _et al._ Ginkgolide B increases healthspan and
lifespan of female mice. _Nat Aging_ 5, 237–258 (2025). https://doi.org/10.1038/s43587-024-00802-0 Download citation * Received: 10 May 2023 * Accepted: 20 December 2024 * Published: 31
January 2025 * Issue Date: February 2025 * DOI: https://doi.org/10.1038/s43587-024-00802-0 SHARE THIS ARTICLE Anyone you share the following link with will be able to read this content: Get
shareable link Sorry, a shareable link is not currently available for this article. Copy to clipboard Provided by the Springer Nature SharedIt content-sharing initiative