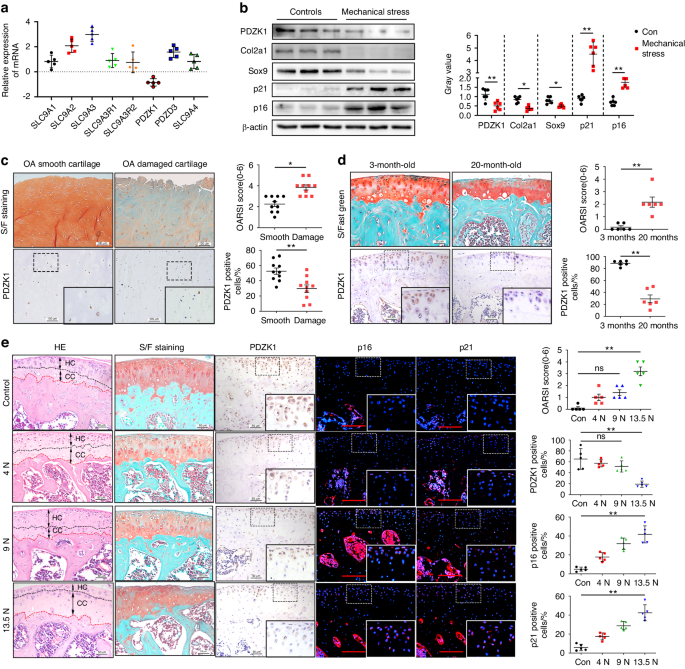
Pdzk1 protects against mechanical overload-induced chondrocyte senescence and osteoarthritis by targeting mitochondrial function
- Select a language for the TTS:
- UK English Female
- UK English Male
- US English Female
- US English Male
- Australian Female
- Australian Male
- Language selected: (auto detect) - EN
Play all audios:

ABSTRACT Mechanical overloading and aging are two essential factors for osteoarthritis (OA) development. Mitochondria have been identified as a mechano-transducer situated between
extracellular mechanical signals and chondrocyte biology, but their roles and the associated mechanisms in mechanical stress-associated chondrocyte senescence and OA have not been
elucidated. Herein, we found that PDZ domain containing 1 (PDZK1), one of the PDZ proteins, which belongs to the Na+/H+ Exchanger (NHE) regulatory factor family, is a key factor in
biomechanically induced mitochondrial dysfunction and chondrocyte senescence during OA progression. PDZK1 is reduced by mechanical overload, and is diminished in the articular cartilage of
OA patients, aged mice and OA mice. _Pdzk1_ knockout in chondrocytes exacerbates mechanical overload-induced cartilage degeneration, whereas intraarticular injection of adeno-associated
virus-expressing PDZK1 had a therapeutic effect. Moreover, PDZK1 loss impaired chondrocyte mitochondrial function with accumulated damaged mitochondria, decreased mitochondrion DNA (mtDNA)
content and increased reactive oxygen species (ROS) production. PDZK1 supplementation or mitoubiquinone (MitoQ) application alleviated chondrocyte senescence and cartilage degeneration and
significantly protected chondrocyte mitochondrial functions. MRNA sequencing in articular cartilage from _Pdzk1_ knockout mice and controls showed that PDZK1 deficiency in chondrocytes
interfered with mitochondrial function through inhibiting Hmgcs2 by increasing its ubiquitination. Our results suggested that PDZK1 deficiency plays a crucial role in mediating excessive
mechanical load-induced chondrocyte senescence and is associated with mitochondrial dysfunction. PDZK1 overexpression or preservation of mitochondrial functions by MitoQ might present a new
therapeutic approach for mechanical overload-induced OA. SIMILAR CONTENT BEING VIEWED BY OTHERS SPI1 ACTIVATES MITOCHONDRIAL UNFOLDED RESPONSE SIGNALING TO INHIBIT CHONDROCYTE SENESCENCE AND
RELIEVES OSTEOARTHRITIS Article Open access 14 April 2025 SIRT3-PINK1-PKM2 AXIS PREVENTS OSTEOARTHRITIS VIA MITOCHONDRIAL RENEWAL AND METABOLIC SWITCH Article Open access 14 March 2025
TBK1-MEDICATED DRP1 PHOSPHORYLATION ORCHESTRATES MITOCHONDRIAL DYNAMICS AND AUTOPHAGY ACTIVATION IN OSTEOARTHRITIS Article 25 August 2022 INTRODUCTION Osteoarthritis (OA) is the most common
degenerative joint disorder that represents a leading cause of disability and source of socioeconomic cost in older adults.1,2,3 It is recognized that mechanical overloading and advanced age
are the main risk factors involved in OA pathogenesis, but the mechanisms have, to date, not been elucidated.4,5,6 In our previous study, we found that excessive mechanical overload
promoted chondrocyte senescence and OA development.7 However, the molecular and cellular mechanisms that underlie the transduction of physical signals to biochemical signals resulting in OA
progression have not been fully elucidated.8,9,10 Mitochondria, which are membrane-enclosed organelles and produce cellular energy in the form of adenosine11 triphosphate (ATP), are
currently a focus of biomedical research because of their role in aging and the development of OA pathologies.12,13 Growing evidence suggests that mitochondrial dysfunction not only leads to
cellular senescence, but also accelerates organismal aging via mechanisms associated with mitochondrial reactive oxygen species (ROS) production, mitochondria DNA (mtDNA) mutations and
mitochondrial biogenesis.11,14,15,16 Targeted clearance of mitochondrial ROS production can significantly reduce Matrix Metallopeptidase (MMP) secretion by OA chondrocytes and delay OA
cartilage degeneration.17,18 Recent studies have identified mitochondria as a critical mechano-transducer situated between extracellular mechanical signals and chondrocyte biology, and their
function can be disordered as early as after a few minutes on mechanical overloading, leading to chondrocyte damage.19,20 Therefore, we propose that mitochondria play an important role in
chondrocyte senescence resulting from mechanical overload, but the specific mechanisms by which mechanical stimulation leads to alteration in mitochondrial function remains to be identified.
Recently, accumulating evidence has shown that chondrocyte homeostasis can be regulated by various ion channels, which are the first to respond to mechanical stimuli.21,22 Although
chondrocytes are non-excitable cells, they have a rich complement of ion channels, which includes potassium channels, sodium channels, transient receptor potential calcium or non-selective
cation channels and chloride channels.23 For instance, sodium-hydrogen exchanger1 (NHE1) and NHE regulatory factor1 (NHERF1) in chondrocytes is associated with OA.24,25 NHERF serve as acts
as a scaffold for a variety of proteins to modulate multiple NHE by forming complexes, in addition to binding cytoskeleton to perceive mechanical stress signals and transduce them into
biochemical signals.26 Therefore, it is reasonable to hypothesis that alterations of NHERF could be associated with OA pathogenesis under mechanical overloading. Our group preliminarily
examined this hypothesis by quantitative PCR (qPCR) analysis NHE and NHERF, and found that the gene encoding the PDZ domain containing 1 (PDZK1), was markedly decreased in chondrocytes
treated with mechanical overloading. Because the role of PDZK1 in OA has not previously been investigated, we herein explored the possible functions and underlying molecular mechanisms of
PDZK1 in OA pathogenesis. In this study, significant PDZK1 suppression was detected during chondrocyte senescence and OA progression in response to mechanical overload. _Pdzk1_ knockout in
chondrocytes exacerbated mechanical overload-induced cartilage degeneration, whereas supplementary PDZK1 administration had a therapeutic effect, indicating the essential role of PDZK1 in
maintaining cartilage homeostasis. Moreover, PDZK1 loss impaired chondrocyte mitochondrial function through protect 3-hydroxy-3-methylglutaryl-CoA synthase 2 (Hmgcs2) from ubiquitination.
Our results suggested that PDZK1 deficiency plays a crucial role in mediating excessive mechanical load-induced chondrocyte senescence and is associated with mitochondrial dysfunction.
RESULTS CHONDROCYTE PDZK1 IS A POTENTIAL CANDIDATE DURING CHONDROCYTE SENESCENCE AND OA PROGRESSION IN RESPONSE TO MECHANICAL OVERLOAD To investigate the role of the NHE during OA under
mechanical overload, mouse primary chondrocytes were treated with 0.5 Hz and 20% cyclic tensile strain loading for 24 h and the levels of all NHE and NHERF family members were determined.
PDZK1 appeared to be a promising candidate because of its specific reduction, whereas others were upregulated in response to excessive mechanical loading (Fig. 1a). Western blotting (WB)
analysis of mouse primary chondrocytes confirmed a marked PDZK1 decrease by excessive mechanical loading, which had been previously proved to induce chondrocyte senescence (Fig. 1b). PDZK1
is a scaffold protein that connects plasma membrane proteins and regulatory components whose roles in chondrocytes and OA development are unknown. Immunohistochemical (IHC) staining
demonstrated a loss of PDZK1- chondrocytes in damaged cartilage that underwent excessive mechanical loading, compared to relatively normal cartilage that had not been mechanically loaded
(Fig. 1c and Fig. S1A). Moreover, immunofluorescence (IF) staining showed that the senescence-related markers p16ink4a and p21 were upregulated in association with inhibited PDZK1 in
patients with OA (Fig. S1b). Interestingly, PDZK1 was markedly decreased in senescent chondrocytes from aged (20-month-old) mice compared to young (3-month-old) animals, as well as in
degenerative cartilage that OA mice suffered following destabilization of the medial meniscus (DMM) surgery (Fig. 1d and Fig. S1c, d). We further evaluated associations for the expression
level of PDZK1 and OA severity of mouse which show a positive correlation was observed between changes in PDZK1 levels and corresponding articular cartilage OARSI score (_r_ = 0.701 6, _P_
< 0.000 1), post-DMM surgery time points (_r_ = 0.857 1, _P_ < 0.000 1) (Fig. S2). Moreover, we assessed PDZK1 expression in mouse articular cartilage in response to mechanical
stimulation. As expected, the application of multiple loading episodes at a peak load of 13.5 N for 14 days induced proteoglycan loss with significant fibrillation of the articular surface,
whereas no obvious changes were detected on low mechanical loading of 4 N or 9 N (Fig. 1e). PDZK1-positive articular chondrocytes were progressively reduced, whereas p21 and p16ink4a
increased in response to increasing mechanical load. Taken together, these observations showed that chondrocyte PDZK1 is reduced by mechanical overloading and is decreased in the articular
cartilage of OA patients, aged mice, and OA mice, implicating the potential role of PDZK1 to be a promising prognostic marker and therapeutic target for mechanical overload-induced OA.
PDZK1-KNOCKOUT (_PDZK1_KO) MICE EXHIBIT EXACERBATED SYMPTOMS OF MECHANICAL LOAD-INDUCED OA To determine the specific role of PDZK1 in OA pathophysiology, we generated _Pdzk1_KO mice using
CRISPR-Cas9 (Fig. S3a). Quantitative Real-time PCR (q-PCR), WB and IHC analyses were performed to confirm that PDZK1 was efficiently deleted in the chondrocytes (Fig. S3b–e). Weight and body
length assessment showed no significant phenotypic differences between the _Pdzk1_KO mice and the littermate controls (Fig. S3f). These data indicated that PDZK1 was successfully deleted in
chondrocytes and the PDZK1 loss did not induce skeletal developmental abnormalities in mice. In vitro study showed that _Pdzk1_ deletion promoted chondrocyte senescence because enhanced
senescence-associated β-galactosidase (SA-β-Gal) staining and upregulated γH2AX, a marker of DNA damage, were observed in _Pdzk1_-deficient chondrocyte cultured at passage 6 (Fig. 2a).
However, significant differences in NHE and NHERF family members were found between _Pdzk1_-deficient chondrocytes and littermate controls except SLC9A2 and SLC9A3R1(Fig. S3g). In addition,
p16ink4a- and p21-positive chondrocytes were upregulated in 18-month-old _Pdzk1_KO mice, though no obvious cartilage damage was presented compared to controls, indicating the pro-senescence
effect of PDZK1 loss in chondrocytes (Fig. 2b and Fig. S4a). We used 10-week-old male _Pdzk1_KO mice to establish a DMM OA animal model. The morphology of the tibia plateau was studied by
scanning electron microscopy. A slightly stripped cartilage and superficial avulsion were observed in controls, while _Pdzk1_KO mice presented a larger area of stripped cartilage and
exfoliation, and exposed subchondral bone with microcracks at 4 weeks post-OA surgery (Fig. 2c). In addition, several experimental methods were applied to study OA pathological changes under
_Pdzk1_ deletion. Compared to littermate controls, _Pdzk1_KO mice exhibited an accelerated loss of both proteoglycans and cellularity, leading to increased articular cartilage damage at 8
weeks post-OA surgery, which was further validated by Osteoarthritis Research Society International (OARSI) scale analysis. MMP13-positive chondrocytes were increased, whereas Collagen Type
II Alpha 1 (Col2a1) and SRY-Box Transcription Factor 9 (Sox9) were significantly decreased in _Pdzk1_KO mice compared to control mice. Senescence-related markers p16ink4a, p21, γH2AX and p53
were significantly upregulated by PDZK1 loss (Fig. 2d and Fig. S4b). In addition, we also detected synovial inflammation and subchondral bone changes in _Pdzk1_KO mice and their littermate
controls. In DMM model mice, staining scores exhibited no statistically significant difference in synovial inflammation and subchondral bone phenotype but _Pdzk1_KO mice developed more
MMP13-positive cells compared with littermate controls in synovial (Fig. S5). Collectively, these results suggested that PDZK1 plays a vital role in chondrocyte senescence and OA
progression, and PDZK1 deletion in chondrocytes exacerbates the mechanical overload-induced cartilage degeneration. PDZK1 OVEREXPRESSION DEFERS CHONDROCYTE SENESCENCE AND PROTECTS AGAINST OA
DEVELOPMENT Subsequently, mouse primary chondrocytes were treated with or without adenovirus containing PDZK1 (Ad-Pdzk1) under 20% elongation strain loading for 24 h. It was of particular
interest that the addition of PDZK1 rescued the promotion of the catabolic and senescent effects induced by excessive mechanical loading, with decreased p16ink4a and p21 expression in
Ad-PDZK1-treated chondrocytes (Fig. 3a and b). Subsequently, the effect of PDZK1 overexpression on OA pathogenesis was examined via intra-articular injection of PDZK1-expressing
adeno-associated virus (AAV-Pdzk1) once a week from 7 days after excessive mechanical stress-induced OA surgery. IHC staining indicated that intra-articular injection of adeno-associated
virus elevated PDZK1 expression mainly in articular cartilage, not in subchondral bone of AAV-Pdzk1-treated mice, demonstrating successful AAV-delivered PDZK1 overexpression (Fig. 3c). As
expected, AAV-Pdzk1 effectively alleviated OA development in mice, as manifested by reduced cartilage destruction and proteoglycan loss caused by mechanical overload for 28 days, together
with increased Col2a1 and decreased MMP13 expression, indicating the chondrogenesis effect of PDZK1 overexpression on OA pathogenesis (Fig. 3d). Of note, senescence-related markers p16ink4a,
p21, γH2AX and p53 were significantly downregulated by PDZK1 supplementation (Fig. 3d). Furthermore, the protective effect against chondrocyte senescence and cartilage degeneration by PDZK1
was further verified in the DMM OA model (Fig. S6a). Collectively, these results indicated that PDZK1 plays an essential role in maintaining cartilage homeostasis, and its overexpression
protects mice against OA development. PDZK1 DEFICIENCY IN CHONDROCYTES INHIBITS MITOCHONDRIAL FUNCTIONS Previous studies have shown that excessive mechanical load can impair chondrocyte
mitochondrial function during OA pathogenesis.27 However, the relationship between mitochondrial function and mechanically induced chondrocyte senescence and the regulatory mechanism remains
unclear. Consequently, we examined the role of PDZK1 in mitochondrial function during mechanical overload-induced chondrocyte senescence and cartilage degeneration. The JC-1 staining
technique was applied to detect the mitochondrial membrane potential, which has been shown to be a sensitive indicator of mitochondrial permeability.28 Interestingly, primary chondrocytes
from _Pdzk1_KO mice displayed a significantly increased JC-1 green fluorescence when compared to controls, indicating the accumulation of damaged mitochondria in response to PDZK1 deficiency
(Fig. 4a). The mtDNA content was subsequently analyzed by investigating cytochrome c oxidase subunit 2 (COX2), an important mitochondrially encoded oxidative phosphorylation (OXPHOS)
complex IV subunit to transfer electrons from cytochrome c to oxygen. The mRNA expression levels of mtDNA‐encoded OXPHOS complex III subunit cytochrome b, complex V subunit ATP synthase 6,
and complex IV subunits cytochrome c oxidase 1–3 (COX1, COX2 and COX3) were determined. The mtDNA content and all of these mitochondrially encoded OXPHOS genes were significantly decreased
in primary chondrocytes from _Pdzk1_KO mice (Fig. 4b, c). Chondrocyte mitochondrial mass was analyzed by MitoTracker green and MitoSOX red staining, while ultrastructural analysis of
mitochondria was performed by transmission electron microscopy (TEM). The MitoSOX staining showed that mitochondrial ROS production was increased in _Pdzk1_KO chondrocytes, as assessed by
the mean fluorescence (Fig. 4e). In TEM analyses, normal round or rod‐shaped mitochondria and a regular arrangement of mitochondrial cristae were detected in control mice, while mitochondria
became small and ovate, with little apparent cristae structure in _Pdzk1_KO mice (Fig. 4d). Additionally, there was no discernible distinction of rate-limiting enzymes associated with
glucose metabolism and lipid metabolism between _Pdzk1_KO chondrocytes and littermate controls (Fig. S7). Subsequently, the outer mitochondrial membrane protein translocase of outer
mitochondrial membrane 20 (TOMM20) and mitophagy markers PTEN-induced putative kinase 1 and E3 ubiquitin protein ligase (PARKIN) were assessed in _Pdzk1_KO mice and littermate controls that
underwent DMM surgery. As expected, TOMM20-, PINK1- and PARKIN-positive chondrocytes were markedly decreased during OA, and further still in PDZK1-deficient chondrocytes (Fig. 4f). Overall,
PDZK1 plays an important role in maintaining mitochondria intact and their natural function in chondrocytes. MITOQUINONE (MITOQ) PRESERVES MITOCHONDRIAL FUNCTIONS AND INHIBITS CHONDROCYTE
SENESCENCE INDUCED BY PDZK1 LOSS To further investigate the role of PDZK1 in mitochondrial dysfunction and its association with excessive mechanical stress-induced cellular senescence,
primary chondrocytes from _Pdzk1_KO mice or controls were treated at passage 3 with the mitochondria-targeted antioxidant MitoQ. Damaged mitochondria and elevated mitochondrial ROS
production were rescued by MitoQ treatment in PDZK1-deficient chondrocytes, indicating that MitoQ can restore the mitochondrial function impaired by PDZK1 loss (Fig. 5b). Additionally, MitoQ
inhibited chondrocyte senescence by maintaining membrane integrity (Fig. 5a). PDZK1 loss induced increased γH2AX in chondrocytes, which were significantly reduced by MitoQ, which was
further confirmed by SA-β-Gal staining (Fig. 5b and online Supplemental Fig. S8). We cultured chondrocytes in 3D to simulate environment in vivo. The results showed that MitoQ remarkably
decreased 3D-cultured chondrocyte senescence caused by devoid of oxygen (Fig. S9). Moreover, the protective effect of MitoQ in mechanical overload-induced cellular senescence was verified
through experiments in cartilage explants from 3-week-old male mice. Significant cartilage destruction and proteoglycan loss was observed in cartilage explant under the application of
multiple loading episodes at a peak load of 4 N for 24 h, together with markedly inhibited PDZK1 expression. Of note, MitoQ treatment not only alleviated cartilage destruction, but also
restored chondrocyte physiological function impaired by mechanical overload, together with increasing Col2a1 and decreasing MMP13, p16ink4a and p21 expression (Fig. 5c). Collectively, these
results suggested that MitoQ protected against chondrocyte senescence and cartilage destruction caused by PDZK1 loss. PDZK1 DEFICIENCY IN CHONDROCYTES INTERFERES WITH MITOCHONDRIAL FUNCTION
THROUGH HMGCS2 To investigate the mechanisms through which PDZK1 regulates mitochondrial function and chondrocyte senescence, the mRNA expression profile of articular cartilage from
_Pdzk1_KO mice and their littermate controls was analyzed (Fig. 6a). By performing Gene Ontology and Kyoto Encyclopedia of Genes and Genomes analysis, we found that genes associated
mitochondrial function were considerably abated in _Pdzk1_KO mouse cartilage, and Hmgcs2 (3-Hydroxy-3-Methylglutaryl-CoA Synthase 2) was the most highly downregulated gene induced by
excessive mechanical stress (Fig. 6b). Because PDZK1 can influence protein expression by preventing its ubiquitination,29 we examined the effect of PDZK1 silencing and overexpression on the
levels of Hmgcs2 ubiquitination in the presence of the proteasome inhibitor MG132. Results showed that PDZK1 associated with Hmgcs2, and medicated Hmgcs2 ubiquitination and degradation by
proteasomes (Fig. 6c). Hmgcs2 is a mitochondrial enzyme that catalyzes the first reaction of ketogenesis, which includes acetoacetic acid, β-hydroxybutyric(BHB) acid and acetone. IHC
staining showed that Hmgcs2 was expressed abundantly in normal chondrocytes, whereas it was significantly decreased during OA and in PDZK1-deficient chondrocytes (Fig. S10a–d). Importantly,
the protective effect of PDZK1 against mitochondrial dysfunction and chondrocyte senescence induced by excessive mechanical stress was further diminished by silencing Hmgcs2, as confirmed by
the detection of elevated senescence markers γH2AX, SA-β-Gal and MitoSOX staining (Fig. 6d and Fig. S10e). Besides, mitochondrial functions were turned upside down by silencing Hmgcs2 of
primary chondrocytes (Fig. S11). In addition, we further deciphered the effects of BHB on chondrocyte senescence and cartilage homeostasis using mouse primary chondrocytes incubated with
either mechanical stress or PDZK1 siRNA. Results showed that BHB rescued the promotion of the catabolic and senescent effects induced by excessive mechanical loading or siRNA, with decreased
p16ink4a and p21 expression in BHB-treated chondrocytes (Fig. 6e, f). Taken together, the above findings indicated that PDZK1 loss may promote chondrocyte mitochondrial dysfunction and
senescence through Hmgcs2. DISCUSSION In the present study, we identified PDZK1 as a key factor in biomechanically-induced mitochondrial dysfunction and chondrocyte senescence during OA
progression. We showed that PDZK1 expression was markedly downregulated by mechanical overload, which in turn impaired chondrocyte mitochondrial functions through inhibiting Hmgcs2
posttranslationally by increasing its ubiquitination, consequently exacerbating chondrocyte senescence and cartilage degeneration (Fig. 7). PDZK1 supplementation or preservation of
mitochondrial functions is thus a potential therapeutic target for OA treatment. Mechanical overloading and aging are two essential factors for OA. We previously proved that excessive
mechanical loading stimulates chondrocyte senescence and consequently initiates and accelerates OA development, but the mechanism is not completely understood.7 The mitochondrion, an energy
factory that converts nutritional molecules into ATP via oxidative phosphorylation, has been identified as a mechano-transducer situated between extracellular mechanical signals and cell
biology.27,30 It has been implied that mechanical stress affects mitochondrial ATP production and induces membrane potential change and increased oxytoxin generation and release, which act
as a causal link between mitochondrial dysfunction and OA pathogenesis.31,32 However, the role and associated mechanism of the mitochondrion in mechanical stress-associated chondrocyte
senescence and OA are not elucidated. Therefore, a better understanding of mitochondrial function would be of great significance to ameliorate aging and delay OA progression. PDZK1 is a
member of the PDZ protein family, contains four PDZ domains, can bind to various proteins through its PDZ domain, and participates in tumor formation and development.33,34 It is involved in
regulating multiple signal transduction pathways, which have the functions of protein regulation, membrane protein modification, and playing an important role in ion transport, protein
localization, cell proliferation and differentiation, and intercellular interaction.35,36 Although PDZK1 has been widely studied, its role in mitochondrial function and chondrocyte
senescence has not been reported. In the current study, we found that PDZK1 was markedly downregulated in chondrocytes on excessive mechanical loading in vitro and in vivo. PDZK1 deletion in
chondrocytes resulted in a decreased number of mitochondria and abnormal structure associated with mitochondrial dysfunction, which contributes to chondrocyte senescence and articular
cartilage degeneration and consequently exacerbation of OA. In addition, we detected senescent chondrocytes in articular cartilage of DMM-OA mice and on mechanically overloading mice, which
was exacerbated by PDZK1 deletion and reversed by PDZK1 overexpression or MitoQ supplementation. Therefore, we propose that mechanical overloading reduces PDZK1 expression in chondrocytes,
which represents a novel mechanism for mitochondrial dysfunction and chondrocyte senescence during OA. To identify the PDZK1 downstream mechanisms during chondrocyte senescence and OA
development, we demonstrated the protein interaction of PDZK1 with Hmgcs2, which was downregulated in chondrocytes after mechanical overloading. The protein encoded by _Hmgcs2_, as a member
of the HMG-CoA synthase family, is a kind of mitochondrial enzyme that catalyzes the first reaction of ketogenesis, a metabolic pathway that provides lipid-derived energy for various organs
during times of carbohydrate deprivation.37,38 However, the role of Hmgcs2 in maintaining mitochondria function and OA pathogenesis has not been studied. We found that Hmgcs2 participated in
mechanical overloading-induced mitochondrial dysfunction and was downregulated in the articular cartilage of OA and _Pdzk1_KO mice. In the present study, we found that PDZK1 inhibition by
excessive mechanical stress strongly decreased Hmgcs2 expression and subsequently induced ROS accumulation, resulting in enhanced cell senescence. Additionally, the protective effect of
PDZK1 by Ad-_Pdzk1_ can be diminished by silencing Hmgcs2. These results indicated that the PDZK1-Hmgcs2 pathway plays an important role in mechanical stress-induced chondrocyte
mitochondrial dysfunction and senescence. In conclusion, our study found that PDZK1 deficiency-induced mitochondrial dysfunction plays an essential role in mechanical stress-associated
chondrocyte senescence and OA. Overexpression of PDZK1, its the preservation of mitochondrial functions by MitoQ, or supplement BHB might present a new therapeutic approach for mechanical
overload-induced OA. MATERIALS AND METHODS HUMAN OA SPECIMENS Human tibia plateaus were obtained from OA patients undergoing total knee replacement surgery who had signed informed consents.
Specimens from osteoarthritic cartilage were classified as relatively smooth cartilage or severely damaged cartilage (Fig. S1a). Patients with malignant tumors, diabetes, or other serious
chronic diseases were excluded. All samples were obtained from the Department of Orthopedics, The Third Affiliated Hospital of Southern Medical University. PRIMARY CHONDROCYTE ISOLATION AND
CULTURE Primary chondrocytes were isolated from C57BL/6 J and _Pdzk1_KO mice (1-week-old). Specifically, cartilage tissues were obtained from the tibial plateau and first digested for 30 min
in 0.25% trypsin-EDTA at 37 °C. Cartilage tissues were cut into 1–2 mm pieces and digested in 0.2% collagenase II for 6 h at 37 °C in a constant-temperature shaker (Hecheng, Shanghai,
China). Subsequently, chondrocytes were collected and resuspended in DMEM/F12 supplemented with 10% fetal bovine serum and 1% penicillin-streptomycin (Corning, USA). 3D CULTURE To mimic the
environment in vivo, we cultured the chondrocytes in the VitroGel® RGD High Concentration hydrogel (The Well Bioscience, TWG003). We adjusted the concentration of VitroGel by diluting the
VitroGel with VitroGel Dilution Solution. After dilution, we gently mixed the diluted VitroGel with a cell suspension of 2 × 106 cells/mL without introducing bubbles. The hydrogel mixture
were transferred to 6-well plate and waited 20 min at room temperature for a soft gel formation. Then we covered cover hydrogel with additional medium to further stabilize the hydrogel and
changed cover medium every 48 h. IN VITRO MECHANICAL OVERLOADING EXPERIMENT Aberrant mechanical force was performed using a Flexcell FX-5000 tension system (Flexcell International Corp,
USA), as previously described.7 Primary chondrocytes in the present study were treated with 20% tension intensity at 0.5 Hz for 24 h and collected for further analyses. EXPERIMENTAL OA AND
HISTOLOGICAL ANALYSES All C57BL/6 J male mice were purchased from the Laboratory Animal Center of Southern Medical University (Guangzhou, China). Experimental OA was induced by DMM surgery
using 10-week-old male mice.39 Cartilages were processed for histological and biochemical analyses. Knee joints were fixed in 4% paraformaldehyde for 24 h, decalcified in 10% EDTA (pH 7.4)
for 21 days (replenished every 3 days), dehydrated and embedded in paraffin. The paraffin blocks were sectioned at 4-µm thickness and sectioned joints were stained by safranin O and HE
staining. The degree of cartilage degradation was scored at grades 0–6 using the OARSI scoring system and evaluated by the ratio of hyaline cartilage layer thickness to calcified cartilage
layer thickness. The images were acquired using a ZEISS Axio Scope A1 (ZEISS, German). For Krenn’s synovitis inflammation scoring system, safranin O slides were to evaluate by scoring
enlargement of the synovial lining cell layer, density of the resident cells, and inflammatory infiltrate, graded separately (from 0 to 3). For subchondral bone grading, safranin O slides
were to evaluate by subchondral bone sclerosis, subchondral bone plate thickness and trabeculae grading four-stage. IN VIVO MECHANICAL OVERLOAD ANIMAL MODEL Right knee joints of mice were
subjected to 60 cycles of 4 N, 9 N or 13.5 N axial compressive loads at 0.1 Hz twice a week to perform mechanical overload-induced OA with the Electro Force 5100 (USA). Control mice were
placed within the test frame and their knees were subjected to 10 min of a static axial compressive load at 0.5 N. After 4 weeks, mice were euthanized for the collection of knee-joint
specimens. Subsequently, the samples were fixed, decalcified, embedded and sectioned. The Southern Medical University Animal Care and Use Committee approved all procedures involving mice.
GENERATION OF _PDZK1_KO MICE Global _Pdzk1_KO mice were generated by germline transmission of an RGEN-induced mutant allele. PDZK1-specific guide RNA and Cas9 protein (each 50 ng/μL) were
injected into the cytoplasm of C57BL/6 J mouse eggs and transferred into the pseudo-pregnant foster female mice. The absence of PDZK1 in the mutants was confirmed by routine tail DNA
genotyping and WB. The absence of PDZK1 was confirmed by standard PCR genotyping (Fig. S2). CARTILAGE EXPLANTS Three-week-old male C57 mice were euthanized to isolate tibial plateau
cartilage explants. A stereo microscope (Olympus, Tokyo, Japan) was used to blunt dissect cartilage from underlying bone. Explants were cultured for 3 days in DMEM/F12 containing 10% fetal
bovine serum and 1% penicillin-streptomycin in six-well plates (Corning) before further processing. The explants were subjected to 4 N compressive loads at 0.1 Hz for 24 h in BioDynamic
instrument with the Electro Force 5100 (USA). INTRA-ARTICULAR DELIVERY OF PDZK1 OVEREXPRESSION ADENO-ASSOCIATED VIRUS (AAV-PDZK1) IN EXPERIMENTAL OA Overexpressing adeno-associated virus
(AAV-Pdzk1) (IgeBio, GuangZhou, China) was administered to C57BL/6 J male mice with DMM- or mechanical stress-induced OA by intra-articular injection performed once a week post DMM surgery
and mechanical stress-induced OA. The control groups were all treated with negative control (AAV-NC) for the same periods. IMMUNOHISTOCHEMISTRY AND IMMUNOFLUORESCENCE Mouse and human knee
cartilage samples were processed for histology as described previously.7 For IHC, sections were digested with Tris-EDTA buffer at pH 9 for 6 h at 60 °C and incubated overnight at 4 °C with
primary antibody. Horseradish peroxidase (HRP)-conjugated antibody (Jackson ImmunoResearch Laboratories, Inc., West Grove, PA, USA) was used as a secondary antibody and staining substrate,
which was stained with DAB substrate kit (cat# DAB057). For IF, sections were stained with Alexa 488 or Alexa 594 dye-labeled secondary antibodies (Invitrogen, Thermo Fisher Scientific,
USA). Nuclei were labeled with 4, 6-diamidino-2-phenylindole (Sigma, USA) and images were obtained using a FluoView FV1000 confocal microscope (Olympus). Sections were randomly coded and
scored by two blinded observers for three sections per joint. TRANSMISSION ELECTRON MICROSCOPY To assess mitochondrial morphology, primary chondrocytes were collected and fixed in 2.5%
glutaraldehyde/0.1 mol/L phosphate buffer. Ultrathin sections (60 nm) were prepared by a routine procedure40 and examined under an electron microscope (HITACHI HT7800). SCANNING ELECTRON
MICROSCOPY To assess articular cartilage surface structure, right legs were collected and fixed in 2.5% glutaraldehyde/0.1 mol/L phosphate buffer at 4 °C overnight. The samples were rinsed
three times with 0.1 mol/L, pH 7.0 phosphate buffer for 15 min each time, fixed in 1% osmic acid solution for 1 h and the osmic acid waste solution was carefully removed. The samples were
rinsed three times with 0.1 mol/L, pH 7.0 phosphate buffer for 15 min each time, dehydrated using a gradient concentration (including 50%, 70%, 80%, 90% and 95% five concentrations) of
ethanol solutions for 15 min at each concentration, and finally treated with 100% ethanol twice, both times for 20 min. The samples were subsequently treated with a mixture of ethanol and
isoamyl acetate (V/V = 1/1) for 30 min followed by pure isoamyl acetate for 1 h or left overnight. Critical point drying was performed and the sections were coated and observed using a
scanning electron microscope (HITACHI SU8010). MITOTRACKER AND MITOSOX STAINING Mitochondrial superoxide was detected using the fluorescent MitoSOX probe (Invitrogen) and the fluorescent
MitoTracker probe (Yeasen, Shanghai, China). Cells were incubated in Hank’s buffer with 2 μmol/L MitoSOX-Red and 2 μmol/L MitoTracker-Green for 30 min at 37 °C in a 5% CO2 atmosphere, washed
with phosphate-buffered saline (PBS) and assessed by confocal microscopy (Olympus FV1200). ANALYSIS OF MITOCHONDRIAL MEMBRANE POTENTIAL BY FLOW CYTOMETRY Following digestion with 0.25%
trypsin, the cells were observed to become round under the microscope. Serum-containing medium was added to stop the digestion, the cell-containing fluid was collected and centrifuged at 1
000 r/min for 5 min, the supernatant was discarded and 1 mL DMEM/F12 was added to each tube. The cells were resuspend in clear basal medium, while an appropriate amount of JC-1 (200 μmol/L)
was warmed to room temperature, of which 10 μL was added to the medium in each well to a final concentration of 2 μmol/L, shaken, mixed well and incubated for 15–20 min at 37 °C. The
supernatant was removed by suction and the cells washed twice with PBS. After adding 500 μL PBS, the samples were analyzed by flow cytometry (green fluorescence: Ex/Em = 510/527 nm; red
fluorescence: Ex/Em = 585/590 nm) using FlowJo 7.6 software. SA-Β-GAL ASSAY The primary chondrocytes of _Pdzk1_KO mice and control mice were stained using the SA-β-Gal staining kit
(Biovision, Milpitas, CA, USA) according to the manufacturer’s protocol. Following overnight staining at 37 °C, senescent chondrocytes were dyed blue. The positive cells were counted in four
randomly selected fields per treatment (_n_ = 5). MEASUREMENT OF MITOCHONDRIA MTDNA MtDNA was isolated from chondrocytes using genomic and mtDNA isolation kits according to the
manufacturer’s protocol (BioVision Research Products, Mountain View, CA, USA). MtDNA was amplified using primers for the _Cox2_ gene and normalized to ribosomal protein s18. The sequences of
the primer pairs are shown in Table S1. WESTERN BLOTTING ANALYSIS Tissues and cells were lysed using RIPA buffer (Keygen, China) at 0 °C for 10 min. Samples were separated by SDS-PAGE for
90 min, blotted onto nitrocellulose membranes for 2 h and incubated with primary antibodies (in 5% bovine serum albumin, 0.2% NaN3) at 4 °C overnight. Samples were incubated with secondary
antibodies at 37 °C for 1 h. The proteins were detected using an enhanced chemiluminescence kit (ECL kit, Fdbio, China). RNA ISOLATION AND QUANTITATIVE REVERSE TRANSCRIPTION-POLYMERASE CHAIN
REACTION (RT-PCR) Total RNA was isolated from primary chondrocytes and cartilage of mice with TRIzol reagent (Takara Bio Inc, Shiga, Japan). For mRNA quantification, 1 mg total RNA was
purified with genomic DNA remover and reverse transcribed using 5× HiScript II qRT Super-Mix II (Vazyme Biotech, Nanjing, China). Each PCR reaction consisted of 10 μL 2× ChamQ SYBR qPCR
Master Mix (Vazyme), 10 μmol/L forward and reverse primers, and 500 ng cDNA on a light cycler (Roche). Each gene was normalized to the expression level of the housekeeping gene _Gapdh_. The
sequences of the primer pairs are shown in Table S1. ANTIBODIES The following antibodies were used: rabbit anti-PDZK1 (1:1 000 for WB, 1:200 for IF and IHC; ProteinTech, USA), rabbit
anti-γH2AX (1:100 for IF; Cell Signaling Technology), rabbit anti-MMP13 (1:200 for IF, Abcam), rabbit anti-collagen II (1:200 for IF, Abcam), mouse anti-p16ink4a (1:1 000 for WB, 1:200 for
IF; Abcam), rabbit anti-p21 (1:2 000 for WB, 1:200 for IF; Abcam), mouse anti-GAPDH (1:3 000 for WB; ProteinTech), anti-rabbit IgG light chain (1:2 000 for WB; Abcam), HRP-labeled goat
anti-rabbit IgG H&L (1:3 000 for WB, 1:200 for IHC; Abcam), HRP-labeled goat anti-mouse IgG H&L (1:3 000 for WB; Abcam), Alexa-Fluor-594-labeled goat anti-rabbit IgG H&L (1:800
for IF; Thermo Fisher Scientific), Alexa-Fluor-488-labeled goat anti-rabbit IgG H&L (1:800 for IF; Thermo Fisher Scientific), Alexa-Fluor-594-labeled goat anti-mouse IgG H&L (1:800
for IF; Thermo Fisher Scientific) and Alexa-Fluor-488-labeled goat anti-mouse IgG H&L (1:800 for IF; Thermo Fisher Scientific). STATISTICAL ANALYSIS All experiments were performed at
least three times. All data are presented as the means ± SD using the SPSS version 20.0 software, and graphs were generated with GraphPad Prism 8.0. Differences between two groups were
analyzed using Student’s _t_-tests. For comparison among more than two groups, one-way analysis of variance (ANOVA) followed by Tukey’s or Dunnett’s multiple comparison test was performed.
For comparisons among multiple groups and different time points, two-way ANOVA followed by Sidak’s or Dunnett’s multiple comparisons test was applied. Values of _P_ < 0.05 were considered
to be statistically significant. The experiments were randomized, and the investigators were blinded to allocation during experiments and outcome assessment. DATA AVAILABILITY All data
generated or analyzed during this study are included in this submitted article and its additional files. The mRNA sequencing data has been deposited in the NCBlSRA database under accession
codes PRINA1080460. REFERENCES * Hunter, D. J. & Bierma-Zeinstra, S. Osteoarthritis. _Lancet_ 393, 1745–1759 (2019). Article CAS PubMed Google Scholar * Sharma, L. Osteoarthritis of
the Knee. _N. Engl. J. Med._ 384, 51–59 (2021). Article CAS PubMed Google Scholar * Glyn-Jones, S. et al. Osteoarthritis. _Lancet_ 386, 376–387 (2015). Article CAS PubMed Google
Scholar * Visser, A. W. et al. The relative contribution of mechanical stress and systemic processes in different types of osteoarthritis: the NEO study. _Ann. Rheum. Dis._ 74, 1842–1847
(2015). Article CAS PubMed Google Scholar * Coryell, P. R., Diekman, B. O. & Loeser, R. F. Mechanisms and therapeutic implications of cellular senescence in osteoarthritis. _Nat.
Rev. Rheumatol._ 17, 47–57 (2021). Article PubMed Google Scholar * Rose, J. et al. DNA damage, discoordinated gene expression and cellular senescence in osteoarthritic chondrocytes.
_Osteoarthr. Cartil._ 20, 1020–1028 (2012). Article CAS Google Scholar * Zhang, H. et al. Mechanical overloading promotes chondrocyte senescence and osteoarthritis development through
downregulating FBXW7. _Ann. Rheum. Dis._ 81, 676–686 (2022). Article CAS PubMed Google Scholar * Griffin, T. M. & Guilak, F. The role of mechanical loading in the onset and
progression of osteoarthritis. _Exerc. Sport Sci. Rev._ 33, 195 (2005). * Yuan, X. L. et al. Bone-cartilage interface crosstalk in osteoarthritis: potential pathways and future therapeutic
strategies. _Osteoarthr. Cartil._ 22, 1077–1089 (2014). Article CAS Google Scholar * Zhang, Y. et al. Primary cilium-mediated mechanotransduction in cartilage chondrocytes. _Exp. Biol.
Med._ 248, 1279–1287 (2023). Article CAS Google Scholar * Silva-Pinheiro, P. & Minczuk, M. The potential of mitochondrial genome engineering. _Nat. Rev. Genet_ 23, 199–214 (2022).
Article CAS PubMed Google Scholar * Chan, D. C. Mitochondrial dynamics and its involvement in disease. _Annu Rev. Pathol._ 15, 235–259 (2020). Article CAS PubMed Google Scholar *
Gorman, G. S. et al. Mitochondrial diseases. _Nat. Rev. Dis. Prim._ 2, 16080 (2016). Article PubMed Google Scholar * Valdes, A. M. & Goldring, M. B. Mitochondrial DNA haplogroups and
ageing mechanisms in osteoarthritis. _Ann. Rheum. Dis._ 76, 939–941 (2017). Article CAS PubMed Google Scholar * Zorov, D. B., Juhaszova, M. & Sollott, S. J. Mitochondrial reactive
oxygen species (ROS) and ROS-induced ROS release. _Physiol. Rev._ 94, 909–950 (2014). Article CAS PubMed PubMed Central Google Scholar * Cleveland, K. H. & Schnellmann, R. G.
Pharmacological targeting of mitochondria in diabetic kidney disease. _Pharm. Rev._ 75, 250–262 (2023). Article CAS PubMed Google Scholar * Reed, K. N., Wilson, G., Pearsall, A. &
Grishko, V. I. The role of mitochondrial reactive oxygen species in cartilage matrix destruction. _Mol. Cell Biochem._ 397, 195–201 (2014). Article CAS PubMed Google Scholar * Li, H. et
al. Reprogramming macrophage polarization, depleting ROS by astaxanthin and thioketal-containing polymers delivering rapamycin for osteoarthritis treatment. _Adv. Sci._ (2023). * Goetz, J.
E. et al. Time-dependent loss of mitochondrial function precedes progressive histologic cartilage degeneration in a rabbit meniscal destabilization model. _J. Orthop. Res._ 35, 590–599
(2017). Article PubMed PubMed Central Google Scholar * Bartell, L. R. et al. Mitoprotective therapy prevents rapid, strain-dependent mitochondrial dysfunction after articular cartilage
injury. _J. Orthop. Res._ 38, 1257–1267 (2020). Article CAS PubMed Google Scholar * Barrett-Jolley, R., Lewis, R., Fallman, R. & Mobasheri, A. The emerging chondrocyte channelome.
_Front. Physiol._ 1, 135 (2010). Article CAS PubMed PubMed Central Google Scholar * Kefauver, J. M., Ward, A. B. & Patapoutian, A. Discoveries in structure and physiology of
mechanically activated ion channels. _Nature_ 587, 567–576 (2020). Article CAS PubMed PubMed Central Google Scholar * Mobasheri, A. et al. The chondrocyte channelome: A narrative
review. _Jt. Bone Spine_ 86, 29–35 (2019). Article CAS Google Scholar * Milner, P. I., Wilkins, R. J. & Gibson, J. S. The role of mitochondrial reactive oxygen species in pH
regulation in articular chondrocytes. _Osteoarthr. Cartil._ 15, 735–742 (2007). * Hopwood, B., Tsykin, A., Findlay, D. M. & Fazzalari, N. L. Microarray gene expression profiling of
osteoarthritic bone suggests altered bone remodelling, WNT and transforming growth factor-beta/bone morphogenic protein signalling. _Arthritis Res. Ther._ 9, R100 (2007). * Donowitz, M. et
al. NHERF family and NHE3 regulation. _J. Physiol._ 567, 3–11 (2005) * Jiang, W. et al. Mechanisms linking mitochondrial mechanotransduction and chondrocyte biology in the pathogenesis of
osteoarthritis. _Ageing Res. Rev._ 67, 101315 (2021). Article CAS PubMed Google Scholar * Chazotte, B. Labeling mitochondria with JC-1. _Cold Spring Harb. Protoc._ 2011,
https://doi.org/10.1101/pdb.prot065490 (2011). * Kim, H. et al. Correlation between PDZK1, Cdc37, Akt and breast cancer malignancy: the role of PDZK1 in cell growth through Akt stabilization
by increasing and interacting with Cdc37. _Mol. Med._ 20, 270–279 (2014). Article PubMed PubMed Central Google Scholar * Bolduc, J. A., Collins, J. A. & Loeser, R. F. Reactive
oxygen species, aging and articular cartilage homeostasis. _Free Radic. Biol. Med_ 132, 73–82 (2019). Article CAS PubMed Google Scholar * Chang, S. H. et al. Excessive mechanical loading
promotes osteoarthritis through the gremlin-1-NF-κB pathway. _Nat. Commun._ 10, 1442 (2019). Article PubMed PubMed Central Google Scholar * Jiang, W. et al. Mechanical stress
abnormalities promote chondrocyte senescence - The pathogenesis of knee osteoarthritis. _Biomed. Pharmacother._ 167, 115552 (2023). Article CAS PubMed Google Scholar * Qi, Y. et al. Long
noncoding RNA PENG upregulates PDZK1 expression by sponging miR-15b to suppress clear cell renal cell carcinoma cell proliferation. _Oncogene_ 39, 4404–4420 (2020). Article CAS PubMed
Google Scholar * Zheng, J. et al. Low level of PDZ domain containing 1 (PDZK1) predicts poor clinical outcome in patients with clear cell renal cell carcinoma. _EBioMedicine_ 15, 62–72
(2017). Article PubMed Google Scholar * Cha, B. et al. PDZ domain-dependent regulation of NHE3 protein by both internal Class II and C-terminal Class I PDZ-binding motifs. _J. Biol.
Chem._ 292, 8279–8290 (2017). Article CAS PubMed PubMed Central Google Scholar * Zhao, C. et al. Loss of PDZK1 expression activates PI3K/AKT signaling via PTEN phosphorylation in
gastric cancer. _Cancer Lett._ 453, 107–121 (2019). Article PubMed Google Scholar * Puchalska, P. & Crawford, P. A. Multi-dimensional roles of ketone bodies in fuel metabolism,
signaling, and therapeutics. _Cell Metab._ 25, 262–284 (2017). Article CAS PubMed PubMed Central Google Scholar * Bai, M. et al. LONP1 targets HMGCS2 to protect mitochondrial function
and attenuate chronic kidney disease. _EMBO Mol. Med._ 15, e16581 (2023). Article CAS PubMed PubMed Central Google Scholar * Culley, K. L. et al. Mouse models of osteoarthritis:
surgical model of post-traumatic osteoarthritis induced by destabilization of the medial meniscus. _Methods Mol. Biol._ 2221, 223–260 (2021). Article CAS PubMed Google Scholar * Tizro,
P., Choi, C. & Khanlou, N. Sample preparation for transmission electron microscopy. _Methods Mol. Biol._ 1897, 417–424 (2019). Article CAS PubMed Google Scholar Download references
ACKNOWLEDGEMENTS This work was supported by grants from Natural Science Foundation of China grant No 82172491 (CN), National Natural Science Funds for Excellent Young Scholar No 82322044
(CN), National Key Research and Development Program of China (2022YFC3601902), Youth Talent Support Programme of Guangdong Provincial Association for Science and Technology (SKXRC202308) and
State-funded postdoctoral researcher program No GZC20231062 (CN). AUTHOR INFORMATION Author notes * These authors contributed equally: Yan Shao, Hongbo Zhang, Hong Guan AUTHORS AND
AFFILIATIONS * Department of Joint Surgery, Center for Orthopedic Surgery, The Third Affiliated Hospital of Southern Medical University, Guangzhou, China Yan Shao, Hongbo Zhang, Hong Guan,
Chunyu Wu, Weizhong Qi, Lingfeng Yang, Jianbin Yin, Haiyan Zhang, Liangliang Liu, Yuheng Lu, Yitao Zhao, Chun Zeng, Xiaochun Bai & Daozhang Cai * Department of Orthopedics, Orthopedic
Hospital of Guangdong Province, Academy of Orthopedics Guangdong Province, The Third Affiliated Hospital of Southern Medical University, Guangzhou, China Yan Shao, Hongbo Zhang, Hong Guan,
Chunyu Wu, Weizhong Qi, Lingfeng Yang, Jianbin Yin, Haiyan Zhang, Liangliang Liu, Yuheng Lu, Yitao Zhao, Chun Zeng, Xiaochun Bai & Daozhang Cai * The Third School of Clinical Medicine,
Southern Medical University, Guangzhou, China Yan Shao, Hongbo Zhang, Hong Guan, Chunyu Wu, Weizhong Qi, Lingfeng Yang, Jianbin Yin, Haiyan Zhang, Liangliang Liu, Yuheng Lu, Yitao Zhao, Chun
Zeng, Xiaochun Bai & Daozhang Cai * Orthopedics Department, Qingyuan People’s Hospital, The Sixth Affiliated Hospital of Guangzhou Medical University, Qingyuan, Guangdong, China Hong
Guan & Guiqing Wang * Department of Cell Biology, School of Basic Medical Sciences, Southern Medical University, Guangzhou, Guangdong, China Sheng Zhang & Xiaochun Bai Authors * Yan
Shao View author publications You can also search for this author inPubMed Google Scholar * Hongbo Zhang View author publications You can also search for this author inPubMed Google Scholar
* Hong Guan View author publications You can also search for this author inPubMed Google Scholar * Chunyu Wu View author publications You can also search for this author inPubMed Google
Scholar * Weizhong Qi View author publications You can also search for this author inPubMed Google Scholar * Lingfeng Yang View author publications You can also search for this author
inPubMed Google Scholar * Jianbin Yin View author publications You can also search for this author inPubMed Google Scholar * Haiyan Zhang View author publications You can also search for
this author inPubMed Google Scholar * Liangliang Liu View author publications You can also search for this author inPubMed Google Scholar * Yuheng Lu View author publications You can also
search for this author inPubMed Google Scholar * Yitao Zhao View author publications You can also search for this author inPubMed Google Scholar * Sheng Zhang View author publications You
can also search for this author inPubMed Google Scholar * Chun Zeng View author publications You can also search for this author inPubMed Google Scholar * Guiqing Wang View author
publications You can also search for this author inPubMed Google Scholar * Xiaochun Bai View author publications You can also search for this author inPubMed Google Scholar * Daozhang Cai
View author publications You can also search for this author inPubMed Google Scholar CONTRIBUTIONS Y.S, X.C.B and D.Z.C conceived the ideas for experimental designs, analyzed data and wrote
the manuscript. Y.S and H.B.Z conducted the majority of the experiments and helped with manuscript preparation. H.G conducted the majority of the experiments and analyzed data. C.Y.W, W.Z.Q
and L.F.Y performed immunohistochemistry and immunofluorescence and confocal imaging. J.B.Y, Y.T.Z and H.Y.Z conducted cell cultures and western blot experiments. L.L.L, Y.H.L and S.Z
collected human tissue samples. C.Z, G.Q.W and H.B.Z revised the manuscript. X.C.B and D.Z.C developed the concept, supervised the project and conceived the experiments. All authors approved
the final version of the manuscript. D.Z.C accepted full responsibility for the finished work, had access to the data and controlled the decision to publish. Y.S, H.B.Z and H.G contributed
equally to this work. CORRESPONDING AUTHORS Correspondence to Xiaochun Bai or Daozhang Cai. ETHICS DECLARATIONS COMPETING INTERESTS The authors declare no competing interests. SUPPLEMENTARY
INFORMATION SUPPLEMENTARY INFORMATION RIGHTS AND PERMISSIONS OPEN ACCESS This article is licensed under a Creative Commons Attribution 4.0 International License, which permits use, sharing,
adaptation, distribution and reproduction in any medium or format, as long as you give appropriate credit to the original author(s) and the source, provide a link to the Creative Commons
licence, and indicate if changes were made. The images or other third party material in this article are included in the article’s Creative Commons licence, unless indicated otherwise in a
credit line to the material. If material is not included in the article’s Creative Commons licence and your intended use is not permitted by statutory regulation or exceeds the permitted
use, you will need to obtain permission directly from the copyright holder. To view a copy of this licence, visit http://creativecommons.org/licenses/by/4.0/. Reprints and permissions ABOUT
THIS ARTICLE CITE THIS ARTICLE Shao, Y., Zhang, H., Guan, H. _et al._ PDZK1 protects against mechanical overload-induced chondrocyte senescence and osteoarthritis by targeting mitochondrial
function. _Bone Res_ 12, 41 (2024). https://doi.org/10.1038/s41413-024-00344-6 Download citation * Received: 25 December 2023 * Revised: 29 April 2024 * Accepted: 12 May 2024 * Published: 17
July 2024 * DOI: https://doi.org/10.1038/s41413-024-00344-6 SHARE THIS ARTICLE Anyone you share the following link with will be able to read this content: Get shareable link Sorry, a
shareable link is not currently available for this article. Copy to clipboard Provided by the Springer Nature SharedIt content-sharing initiative