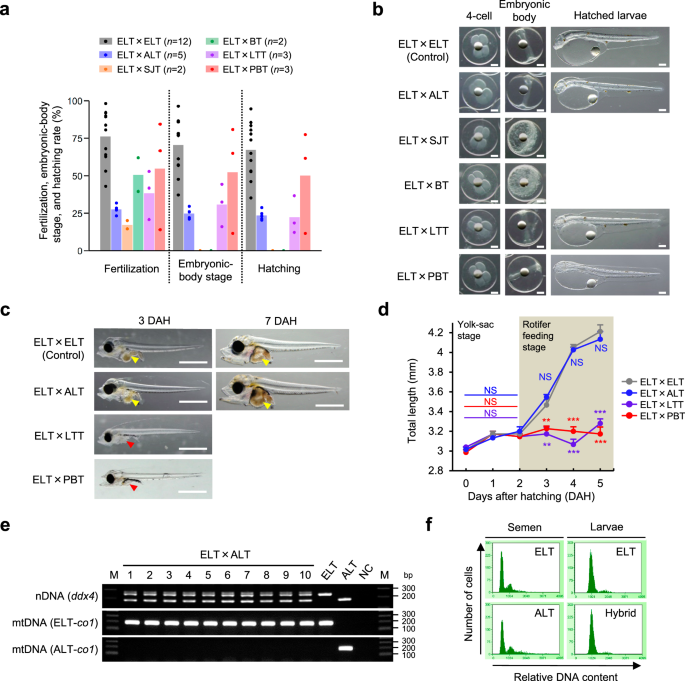
Bluefin tuna sperm production is hastened by surrogacy in small euthynnus
- Select a language for the TTS:
- UK English Female
- UK English Male
- US English Female
- US English Male
- Australian Female
- Australian Male
- Language selected: (auto detect) - EN
Play all audios:

ABSTRACT Pacific bluefin tuna (_Thunnus orientalis_) remains heavily depleted due to overexploitation. Aquaculture and stock enhancement based on artificial seedlings could be effective
solutions to this problem. However, widespread adoption of seedling production is limited because spawning in captivity of bluefin tuna, a large pelagic top predator, requires much space,
time, cost, and labour. To downsize and shorten their generation time, here, we show that xenogeneic germ cell transplantation enables a surrogate production of functional bluefin tuna sperm
in hybrid little tuna (genus: _Euthynnus_), which attain sexual maturity at a smaller body size and shorter time. Intraperitonially transplanted bluefin tuna germ cells migrate towards and
are incorporated into the gonads of little tuna larvae. These recipients produce functional donor-derived bluefin tuna sperm at a mere 8 months of age and 1 kg of body size. This result
represents an advance toward compact, low-cost, and time-efficient seedling production that will improve the efficiency of bluefin tuna aquaculture. SIMILAR CONTENT BEING VIEWED BY OTHERS
GERM CELL RECOVERY, CRYOPRESERVATION AND TRANSPLANTATION IN THE CALIFORNIA WHITE STURGEON, _ACIPENSER TRANSMONTANUS_ Article Open access 06 October 2023 RESCUE OF GERM CELLS IN _DND_
CRISPANT EMBRYOS OPENS THE POSSIBILITY TO PRODUCE INHERITED STERILITY IN ATLANTIC SALMON Article Open access 22 October 2020 A SUSTAINABLE NO-KILL SEA URCHIN AQUACULTURE METHOD TO OBTAIN
CAVIAR Article 08 July 2024 INTRODUCTION Bluefin tunas (_Thunnus thunnus_, _T. orientalis_, and _T. maccoyii_) are among the most commercially valuable fisheries resources worldwide, and
highly migratory large top predators play a key role in the pelagic ecosystem. However, researchers have been concerned about the declining stocks of all bluefin tuna globally because of
overexploitation1,2. According to the latest stock assessment from The International Scientific Committee for Tuna and Tuna-Like Species in the North Pacific Ocean (ISC), the current
spawning stock biomass of Pacific bluefin tuna (PBT) remains low at only 10.2% of the unfished level, which is substantially below the 20% threshold adopted as the limited reference point
indicating the disruption of sustainability in other tuna species3. Aquaculture could be an effective solution to alleviate the pressure on wild-capture fisheries. Although the full cycle of
cultivation techniques in PBT has been developed4 and the utilization of artificial seedlings for commercial aquaculture is slowly increasing in Japan, the current PBT aquaculture remains
heavily dependent on capture-based aquaculture, which comprises harvesting wild juveniles as seedlings and raising them to marketable size, leading to high demand for wild stocks5,6,7.
Improvement and simplification of artificial seed production is urgently needed to promote its widespread adoption to address this situation. One of the major obstacles to obtaining
fertilized eggs in PBT is large body size of mature adults. PBT requires 3–5 years to reach sexual maturity and reaches nearly a hundred kilograms in body weight (BW)8,9,10. Accordingly, the
maintenance and spawning of such large PBT broodstocks in captivity require vast sea cages, substantial feeding costs, and intensive labor9,10. Additionally, the long generation time makes
this breeding program a time- and labour-intensive process. Indeed, PBT does not have any domesticated strains with superior genetic traits. This issue is also one of the reasons why the
widespread usage of artificial seedlings is limited. To solve these problems, we propose the surrogate production of PBT gametes by small-bodied scombrids with shorter generation times using
germ cell transplantation technology11,12. The realization of this approach would revolutionize the artificial seed production of PBT into a compact, low-cost, and time-efficient process
through small land-based fish tanks and accelerate breeding by reducing the generation time. Using rainbow trout as a model, we previously demonstrated that germline stem cells transplanted
into the peritoneal cavity of newly hatched larvae migrate to the embryonic gonad, into which they are incorporated and initiate gametogenesis13. Importantly, we also found that donor
germline stem cells can be transplanted into not only allogeneic but also xenogeneic recipients without immune rejection because the newly hatched larvae used as recipients do not have a
mature immune system14,15. By employing these fundamental findings, we attempted to explore suitable recipients for xenogeneic PBT sperm production within scombrids that attain sexual
maturity at a smaller body size and in a shorter generation time. Consequently, we revealed that xenogeneic germ cell transplantation facilitates surrogate production of functional PBT sperm
in hybrid little tuna (genus: _Euthynnus_) at a mere eight months of age and 1 kg body size. Although the production efficiency of donor-derived PBT gametes still has to be improved, this
study opens the possibility of highly efficient seed production of PBT. RESULTS SELECTION OF SUITABLE SPECIES FOR SURROGATE RECIPIENTS To identify a suitable species to be used as a
surrogate recipient for transplantation of PBT germ cells, we first compared transplantation efficiency using three small-bodied scombrids with varying spawning temperatures, namely,
internal body temperature during the larval stage: chub mackerel (_Scomber japonicus_), which is distributed in temperate waters; striped bonito (_Sarda orientalis_); and Eastern little tuna
(ELT; _Euthynnus affinis_), both of which are distributed in tropical to subtropical waters. PBT testicular germ cells prepared from immature testes were labelled with the red fluorescent
dye PKH26 and intraperitoneally transplanted into these recipient larvae. At 7–14 days after transplantation, donor PBT germ cells showing red fluorescence were incorporated into the gonads
of striped bonitos (12.0%, 3/25 individuals) and ELT (19.2%, 20/104 individuals) more efficiently than those of chub mackerel (1.3%, 3/225 individuals). This result suggested that striped
bonito and ELT, which are expected to have a similar internal body temperature during the larval stage with PBT, are suitable as surrogate recipients for supporting the incorporation of PBT
germ cells into recipient’s genital ridges. Furthermore, ELT exhibited the highest incorporation rate of donor PBT germ cells into the recipient gonads. Moreover, we confirmed that ELT can
also incorporate germ cells isolated from prepubertal 1 year-old PBT testes (Supplementary Fig. 1). However, ELT exhibited a poor survival rate during the larval stage due to multiple
mortality-related factors that are prevalent in scombrids, such as floating death, sinking death, and cannibalistic behaviour16,17. Consequently, the survival rate of transplanted ELT
recipients until the maturation period was frequently <1%, making it difficult to apply germ cell transplantation technology in this species. PRODUCTION OF INTERSPECIFIC HYBRIDS OF ELT
Although we had succeeded in inducing triploidy in ELT to be used as recipients, mortality was markedly high in triploid compared with diploid ELT in the juvenile stage because of selective
cannibalism by diploid siblings17. In contrast, we previously found that an interspecific hybrid of female blue mackerel (_Scomber australasicus_) × male chub mackerel possesses a germ
cell-less sterile gonad18 and exhibits a higher survival rate than parental species due to heterosis. Thus, this study attempted to generate interspecific hybrids of ELT for improvements in
sterility and survival. Most scombrids do not have established techniques for inducing spawning and collecting unfertilized eggs, so we employed a strategy that produces hybrids by
artificial insemination of fresh eggs of ELT reared in our facility with cryopreserved sperm of various wild species. We captured mature males of four related species across the three
genera: skipjack tuna (_Katsuwonus pelamis_), bullet tuna (_Auxis rochei_), longtail tuna (_Thunnus tonggol_), and PBT, all of which are distributed in Japan’s coastal waters (Supplementary
Fig. 2), and immediately cryopreserved their sperm upon capture. Insemination tests revealed that cryopreserved sperm of all four species could fertilize ELT eggs and initiated cleavage
(Fig. 1a, b, Supplementary Table 1). The embryo development of skipjack tuna and bullet tuna hybrids was arrested at the epiboly stage (Supplementary Fig. 3), whereas longtail tuna and PBT
hybrids reached hatching (Fig. 1a, b, Supplementary Table 1). However, both longtail tuna and PBT hybrid larvae did not ingest rotifers used as the initial diet even at 3 days after hatching
(DAH), when feeding onset occurred in nonhybrid ELT (Fig. 1c), resulting in stagnant growth, and no hybrid larvae survived beyond 6 DAH (Fig. 1d). Thus, all tested intergenus hybrids
exhibited lethality. To overcome this, we sought to generate an intragenus hybrid by utilizing fish species belonging to the genus _Euthynnus_. In this study, in vitro fertilization was
performed using cryopreserved sperm of the Atlantic little tuna _E. alletteratus_ (ALT) and eggs of ELT and revealed that the resulting embryos (hereafter called hybrid little tuna, HLT)
started normal development and eventually reached hatching (Fig. 1a, b). Furthermore, HLT larvae ingested rotifers at 3 DAH similar to those of nonhybrid ELT (Fig. 1c), indicating that the
feeding onset of HLT occurred normally. Accordingly, the subsequent growth until 6 DAH did not show any significant differences between HLT and nonhybrid ELT (Fig. 1d). PCR analysis using
species-specific primers confirmed that all tested HLTs possessed nuclear DNA derived from maternal ELT and paternal ALT, as well as mitochondrial DNA derived from maternal ELT (_n_ = 10;
Fig. 1e), demonstrating that no HLTs showed signs of parthenogenesis. Additionally, the genome-wide SNP analysis found 4,238 SNPs carrying both parental species alleles in the HLT genome
without biased distribution (Supplementary Data 1). Supplementary Fig. 4 presents typical examples of these SNPs. Moreover, analyses of cellular DNA content using flow cytometry revealed
that all tested HLTs exhibited the same peaks for DNA contents observed in diploid ELT, and none showed signs of aneuploidy (_n_ = 10; Fig. 1f). These results confirmed that the HLT is
diploid hybrid inherited female ELT and male ALT genomes. SUITABILITY OF HLT AS SURROGATE RECIPIENTS The survival and growth of HLT were compared with those of nonhybrid ELT during three
developmental stages, which were classified based on the feeding scheme as follows: 0–9 DAH (yolk-sac stage to rotifer feeding stage), 9–14 DAH (larva feeding stage), and 14–18 DAH (larva
feeding with cannibalistic behaviour stage) (Fig. 2a). The observation of external morphology indicated that HLT showed no particular deformation (Fig. 2b). At 14 and 18 DAH, the standard
length of HLTs was significantly higher than that of nonhybrid ELTs (Fig. 2c). Notably, the survival test revealed that no significant differences were observed during 0–9 and 14–18 DAH
between HLT and nonhybrid ELT, whereas the survival rate during 9–14 DAH of HLT was 2.6-fold significantly higher than that of nonhybrid ELT (Fig. 2d). Since germ cell transplantation is
performed at ~9 DAH in HLT, this result implies that the recipient will have high survival after transplantation, which is an important characteristic of the recipient. HLTs were reared
until the maturation period to assess their fertility. One-year-old HLT showed a similar external appearance to nonhybrid ELT (Fig. 2e). The fork length (FL) and BW of 1 year-old fish did
not show any significant differences between HLT and nonhybrid ELT (Fig. 2f). Additionally, the gonadal somatic index of 1 year-old HLT did not differ significantly from that of nonhybrid
ELT of either sex, indicating that HLT of both sexes show fertility (Fig. 2g). Although HLT did not show hybrid sterility, they exhibited rapid growth and high survival during the larval
stage. Because ELT demonstrated quite low early survival, the improved survival of HLT was a meaningful advance in their use as recipients for PBT germ cell transplantation. SURROGATE
PRODUCTION OF DONOR-DERIVED PBT SPERM PBT testicular germ cells containing a large number of type A spermatogonia (Fig. 3a, b; Supplementary Fig. 5) were intraperitoneally transplanted into
a total of 6,300 HLT larvae at 8–10 DAH in three independent trials (Fig. 3c, Supplementary Table 2). Seven days after transplantation, the incorporation of transplanted PBT germ cells into
HLT recipient gonads was assessed by immunohistochemistry (IHC) using an anti-DEAD-box helicase 4 (Ddx4) polyclonal antibody as a marker for germ cells of both the donor and recipient and
monoclonal antibody No. 149, which specifically recognizes PBT germ cells in the recipient gonad19,20. No antibody No. 149-positive germ cells were detected in the gonads of nontransplanted
HLT (Fig. 3d, left column), whereas antibody No. 149- and Ddx4-double positive PBT germ cells were clearly detected in the gonads of transplanted HLT recipients (Fig. 3d, right column). The
frequency of occurrence of HLT recipients incorporating donor-derived PBT germ cells was 68.0% (_n_ = 25, Supplementary Table 2). The mean number of incorporated PBT germ cells found in the
HLT recipients was 2.5 ± 0.4 (_n_ = 25, range from 1 to 8, Supplementary Table 2). Note that host individuals were observed to retain an average of 10.8 ± 0.6 endogenous germ cells at this
juncture (_n_ = 5). These results demonstrated that HLT gonads possessed the capability to attract and incorporate transplanted PBT germ cells. To examine whether HLT recipients produced
donor-derived PBT sperm, we generated another antibody, No. 102B, which can specifically recognize PBT sperm but not HLT sperm. Immunocytochemistry (ICC) analysis of PBT and HLT sperm smears
demonstrated that antibodies No. 14920 and No. 102B strongly recognize PBT sperm, while HLT sperm were not recognized by these antibodies (Fig. 4a). This specificity for the PBT sperm was
confirmed through the absence of No. 149- and No. 102B-positive sperm in at least 200 million HLT sperm (Supplementary Fig. 6). Furthermore, antibody No. 149 strongly recognized the cell
surface of the sperm head, whereas antibody No. 102B strongly recognized that of the sperm head and tail (Fig. 4b). In total, 126 of 6300 (survival rate = 2.0%) transplanted HLT recipients
survived until the maturation period (Supplementary Table 2). Milt was obtained from 68 male recipients and subjected to ICC with PBT-sperm specific antibodies. As shown in Fig. 4c, antibody
No. 149- and No. 102B-double-positive sperm were clearly detected in the milt of HLT recipients, indicating that HLT recipients produced donor-derived PBT sperm. These PBT sperm detected in
the recipient milt demonstrated typical sperm morphology and were indistinguishable from the control PBT sperm (Fig. 4c, Supplementary Fig. 7). PBT sperm were detected in 9 of 68 (13.2%)
milt samples of male HLT recipients (Supplementary Table 2). The ratio of PBT sperm to recipient sperm ranged from 1/5 million to 1/2.5 million (Supplementary Table 3). Notably,
donor-derived PBT sperm were produced by HLT recipients as young as 8 months old (ranging from 8 to 12 months old). This result indicated that PBT sperm, which normally required sexual
maturity at 3–5 years with ~100 kg of BW, was produced by HLT recipients at a mere of 8 months with ~1 kg of BW. These results demonstrated that HLT recipients show complete spermatogenesis
of xenogeneic PBT germ cells and produce donor-derived PBT sperm with a shorter generation time and smaller body size. Following a procedure of a previous report21, we estimated that
differentiated spermatogonia of PBT and HLT undergo eight mitosis cycles and two subsequent consecutive cycles of meiosis to reach sperm (Supplementary Table 4); thus, one donor
spermatogonium can produce up to 1,024 sperm. In the current study, the maximum number of donor-derived PBT sperm produced in the HLT recipient testes was 4.9 × 104. The incorporation number
of PBT germ cells in the gonads of HLT larvae ranged from 1 to 8 cells. These values suggest that the donor-derived germ cells may have undergone cell division from 12.6 – 15.6 times, which
was markedly larger than the predicted proliferation rate. Thus, although further detailed studies are needed to reveal whether colonized germ cells are truly germline stem cells, these
data suggest that the colonized germ cells demonstrated stem cell activity capable of self-renewal and differentiation into sperm. FUNCTIONALITY OF DONOR-DERIVED PBT SPERM Progeny tests were
conducted to assess the functionality of the PBT sperm produced by HLT recipients. Fifteen 10 month-old HLT recipients were induced to spawn by administering gonadotropin-releasing hormone
agonist (GnRHa). One day after implantation, the HLT recipients started spawning and continued to do so over the next 4 days. The fertilized eggs obtained from these HLT recipients were
incubated for 12 h to reach the embryonic-body stage, and DNA samples were then extracted from every 100 embryos and subjected to PCR analysis targeting the PBT-specific sequence of _ddx4_
in the nuclear DNA (nDNA) (Fig. 4d). As shown in Fig. 4e, a PBT-specific amplification was observed in one (sample No. 8) of the 183 samples (18,300 embryos) obtained from four spawning
days. In addition to _ddx4_, PCR analysis targeting two additional PBT-specific sequences, _dead end_ (_dnd_) and one microsatellite locus in the nDNA, further confirmed that sample No. 8
was PBT-positive (Fig. 4f). Moreover, sequencing analysis confirmed that sequences of the PCR products amplified from sample No. 8 fully matched the PBT sequence (Supplementary Fig. 8). In
contrast, the PCR analysis targeting a PBT-specific sequence of the _d-loop_ in the mitochondrial DNA (mtDNA) showed no PBT-specific amplification in sample No. 8 (Fig. 4f); that is, at
least one PBT-positive embryo in sample No. 8 had no PBT mitochondria. Since mtDNA is inherited maternally, these results strongly suggested that this embryo was generated by fertilization
of donor-derived PBT sperm and recipient-derived HLT eggs. Therefore, this progeny test demonstrated that the donor-derived PBT sperm produced in the HLT recipient was functional and could
fertilize and initiate embryonic development. DISCUSSION In this study, intraperitoneal germ cell transplantation successfully produced donor-derived functional PBT sperm in small-bodied HLT
recipients within a very short period. Previous studies reported that PBT normally requires 3–5 years to become sexually mature and reaches nearly a hundred kilograms in BW8,9,10. In
contrast, HLT recipients produced donor-derived PBT sperm at a mere 8 months of age and 1 kg of body size. Although we still have to overcome issues concerning the production of
donor-derived PBT eggs, this research represents an advance toward compact, low-cost, and time-efficient seedling production that will improve the efficiency of PBT aquaculture and stock
enhancement. Although PBT was used as the donor species in this study, it is expected that sperm from Atlantic bluefin tuna and southern bluefin tuna, which are very closely related to PBT,
can also be produced by this method. In addition, we succeeded in obtaining fertilized eggs from ELT all year-round by adjusting the water temperature and photoperiod in a 10-ton
semicirculating land-based tank16,22. Since the reproductive and rearing characteristics of ELTs and ALTs are very similar, it is expected that the use of HLT recipients will enable a
year-round supply of PBT sperm in a small land-based fish tank. We have previously reported that a hybrid between chub mackerel and blue mackerel is infertile and germ cell deficient18.
After transplanting PBT germ cells into these infertile recipients, we observed that the transplanted cells migrated to the empty gonads of the recipient18; however, we could not observe the
proliferation and differentiation of these cells. Although it was not possible to determine in this study why the HLTs used in this study were able to support spermatogenesis by
donor-derived PBT germ cells, two possibilities can be considered. The first is the phylogenetic distance: The divergence times between the donor species, genus _Thunnus_, to which bluefin
tuna belong, and each recipient species are 6.6–16.7 million years ago (Mya) for genus _Euthynnus_, to which ELT and ALT belong; 9.1–22.4 Mya for genus _Sarda_, to which striped bonito
belong; and 25.5–49.0 Mya for genus _Scomber_, to which chub mackerel belong23,24,25. The close phylogenetic distance may have played a role in sending various signals from the recipient’s
somatic cells to the donor-derived germ cells11,26. Another possibility is the rearing temperature of the recipients. Bluefin tuna are of subtropical origin and migrate to waters with
temperatures of ~25 °C for spawning27,28, so the optimum temperature for the development of juvenile tuna is ~25 °C, which is the same as that for juvenile HLT. In contrast, the optimal
rearing temperature for juvenile chub mackerel and blue mackerel is ~20 °C29,30. Therefore, it is possible that PBT-derived germ cells transplanted into the recipient mackerels failed to
proliferate and differentiate normally at ~20 °C, which was used for mackerel larvae culture, but that they developed smoothly at ~25 °C, which was used for HLT larvae culture. In this
study, we have concluded that the sperm-producing germ cells in the recipient testes behaved as germline stem cells. This result implies that even larger amounts of sperm could be produced
in the future if the somatic environment of the gonads is improved to facilitate their self-renewal and differentiation activities. Further detailed studies on these points will be an
important future task. The germ cell transplantation system established in this study can be an assisted breeding technology that can simultaneously solve many problems of PBT breeding11,12.
Selection using genomic information will become mainstream in genetic breeding of PBT in the future, but to promote such breeding, researchers must cross a specific female parent with a
specific male parent in a targeted manner. Since in vitro fertilization technology has not yet been developed for any bluefin tuna species, surrogate broodstock technology is expected to be
an effective method for systematic breeding. In other words, by transplanting germ cells from selected elite individuals into many recipients and mating the resulting surrogate males and
females, it is expected that the next generation will have the same genome composition as the next generation obtained by mating the target elite individuals, regardless of which surrogate
males or females are mated during group spawning. The length of the generation time for PBT is an obstacle for repeated selection and breeding, but through the use of the surrogate parents
developed in this study, it will be possible to shorten one generation to 8 months, which will substantially reduce the time needed for the entire breeding process. The key issue for the
future is to enable highly efficient production of both PBT sperm and eggs by using HLT recipients. In germ cell transplantation systems, sterilization of the recipient is important to
improve the efficiency of donor-derived gametogenesis15,31,32. In this study, sterilized ELT could not be used as recipients due to very poor early survival after triploidization or _dnd_
knockdown treatments. However, since the initial survival of HLT has been substantially improved, probably by heterosis, it may be possible to use triploids, _dnd_ gene knockdown and even
knockout using CRISPR/Cas9 in the future. METHODS BROODSTOCK MANAGEMENT All of the animal experiments in this study were performed in compliance with protocols approved by the Institutional
Animal Care and Use Committee (IACUC) of Tokyo University of Marine Science and Technology (TUMSAT). Chub mackerel (BW 600–800 g, FL 30–40 cm) were captured using hook and line and
transferred to the Tateyama Station, Field Science Center of TUMSAT, located in Chiba, Japan (34°97′ N, 139°76′ E). Chub mackerel were maintained in 10-m3 fibre-reinforced plastic (FRP)
tanks with flowthrough seawater (80 l/min) under a natural photoperiod and water temperature. Yearling ELT were caught by sea net or hook and line and were held until they were 2 years old
in a sea net‐pen located in Kushimoto, Wakayama, Japan. ELT broodstock (BW 2.0–2.5 kg, FL 40–50 cm) were transferred to Tateyama station and maintained in a 70 m3 FRP tank with flowthrough
seawater (160 l/min) under a long-day photoperiod (24 h of light) and natural water temperature. SPAWNING INDUCTION For induced spawning of chub mackerel and ELT broodstocks, GnRHa
administration was performed by implantation of sustained‐release cholesterol pellets33,34. GnRHa was prepared via a custom peptide synthesis service (Anygen, Jeollanam-do, Korea). A
cholesterol pellet containing GnRHa at a dose of 100 µg/kg BW was implanted into the abdominal cavity of chub mackerel and the dorsal muscle of the ELT. Spawning induction was conducted
during the corresponding spawning seasons of chub mackerel (April–May) and ELT (July–October), wherein the water temperatures were 18–22 °C and 25–27 °C, respectively. The striped bonito
broodstock were reared at Tokyo Sea Life Park, Tokyo, Japan. Fertilized eggs of striped bonito were obtained from spontaneous spawning of fish maintained in a doughnut-type exhibition tank
(7 m in depth, 90 m in diameter, 2200 m3). LARVAL CULTURE Up to 20,000 fertilized eggs of chub mackerel, striped bonito, ELT, and hybrid ELT were transferred to a 100‐l polycarbonate
cylindrical tank (440 mm diameter × 700 mm deep) with flowthrough seawater with a photoperiod of 14 h light (6:00–20:00) and 10 h darkness. Water temperature was maintained at 20–22 °C for
chub mackerel, 23–25 °C for striped bonito, and 25–27 °C for ELT and hybrid ELT. Air was supplied at a rate of 100–300 ml/min through an air stone placed at the centre of the bottom of the
tank. Larvae of all species were fed beginning at 2 DAH with the rotifer _Branchionus plicatilis_ sp. complex, which was fed freshwater-type Chlorella (fresh Chlorella V12; Chlorella
Industry, Tokyo, Japan). The densities of rotifers and _Nannochloropsis sp_. (Marine Fresh; ISC, Fukuoka, Japan) in the tank were maintained at 10–20 rotifers/ml and 5 × 105 cells/mL,
respectively. The chub mackerel and striped bonito were fed _Artemia_ nauplii starting at 11 DAH and 7 DAH, respectively. For increased n-3 fatty acid concentration in the live feed, the
rotifers and _Artemia_ nauplii were incubated with Hyper Gloss (Marine Tech, Aichi, Japan) for 6 to 12 h before feeding. The chub mackerel were fed an artificial diet (Otohime; Marubeni
Nisshin Feed, Tokyo, Japan) from 14 DAH. The striped bonito, ELT, and HLT were fed live hatched larvae of the Japanese whiting _Sillago japonica_ from 9–11 DAH. The ELT and HLT were fed an
artificial diet (Magokoro; Marubeni Nissin Feed) from 16–18 DAH. The ELT and HLT were reared in the 100‐l tank until they reached 40–50 mm in FL at ~25 DAH and were then transferred to 50 m3
tanks. During the rearing period in the 50 m3 tank, the water temperature was maintained at a range from 20 to 27 °C. GERM CELL TRANSPLANTATION Donor testicular cells were prepared from 3
year-old male PBT (BW ~40–60 kg) reared in net pens at Kushimoto, Wakayama, Japan, or Takashima, Nagasaki, Japan. Freshly isolated testes were minced with Weckel scissors and dissociated
with 0.4% collagenase H (Roche, Basel, Switzerland) and 0.03% dispase II (Godo Shusei, Tokyo, Japan) in L-15 medium (pH 7.6; Thermo Fisher Scientific, Waltham, MA, USA) containing 1% fetal
bovine serum (FBS; Thermo Fisher Scientific) and 0.05% DNase I (Roche) at 25 °C. The resulting cell suspension was filtered through a nylon screen with a pore size of 42 µm to eliminate
non-dissociated cell clumps and was then stored on ice until transplantation. Approximately 1.0 × 107 cells were suspended in PKH26 diluted 100 times with diluent C provided in PKH26 Red
Fluorescent Cell Linker Kit (Sigma-Aldrich, St. Louis, MO, USA) at room temperature for 5 min35. The cells were rinsed three times in L-15 and stored on ice until use. In the transplantation
into HLT larvae, PKH26 staining was not performed to minimize damage to donor testicular cells. Spermatozoa and blood corpuscles were eliminated from the whole testicular cell suspensions
by density gradient centrifugation using a Percoll gradient (Percoll Plus; GE Healthcare, Princeton, NJ, USA) that was formed by placing 2 ml of 30% Percoll/phosphate-buffered saline (PBS)
in a 15 ml centrifuge tube and overlaying 2 ml of 20% Percoll/PBS and ~1.0 × 107 to 1.5 × 107 cells/2 ml of L-15 medium. The tube was then centrifuged at 800 × g for 30 min at 4 °C.
Following centrifugation, three distinct cell layers containing testicular cells were visible. Cells at the interface between the 20% and 30% Percoll layers were gently aspirated and washed
three times in excess amounts of L-15 medium. Transplantation into the peritoneal cavity of chub mackerel, striped bonito, ELT, and HLT larvae was performed at 13–14, 8–10, 7–8, and 8–11
DAH, respectively, when their total length was 5–6 mm. At least 10,000 testicular cells were injected into each larva. For confirmation of the incorporation of PKH26-labelled donor germ
cells, genital ridges excised from chub mackerel (~2 cm in total length) at 14 days post-transplantation, and striped bonito and ELT recipients (~2 cm in total length) at 7 days
post-transplantation were imaged under a fluorescence microscope (BX51N-34FL, Olympus, Tokyo, Japan). The incorporation rate of donor-derived germ cells in recipient genital ridges was
calculated as [number of fish incorporating fluorescent cells in genital ridges/number of fish observed × 100]. Incorporation of donor PBT germ cells into genital ridges of HLT was analysed
by IHC as described below. IN SITU HYBRIDIZATION _ddx4-_cDNA fragment (nucleotides 934–2,028 of PBT _ddx4_36, Genbank: EU253482) was subcloned into the pGEM T-easy vector. Antisense RNA
probes were transcribed in vitro using DIG-labeled uridine triphosphate (UTP; Roche) and T7 RNA polymerase (Promega, Madison, WI, USA). Prepubertal testes of 1 year-old PBT were fixed at 4
°C for 16 h in Bouin’s solution. After dehydration in increasing concentrations of ethanol, a portion of each sample was embedded in paraffin wax and cut into 5 μm serial sections. The
paraffin sections were then mounted on Matsunami Adhesive Slides (MAS; Matsunami Glass, Osaka, Japan), dewaxed, and dehydrated by immersion in a xylene-ethanol series. The sections were
stained with hematoxylin-eosin (HE) or processed for ISH with DIG-labeled RNA probes. The sections were then permeabilized, acetylated, and incubated with a hybridization mixture of 1 μg/ml
RNA probe, 50% formamide, 2 × saline-sodium citrate (SSC) (pH 4.5), 50 μg/ml transfer RNA (tRNA), 50 μg/ml heparin, 1% sodium dodecyl sulfate (SDS), and 10% dextran sulfate. After
hybridization at 65 °C for 16 h, the sections were washed as follows: twice in 5 × SSC/50% formamide at 65 °C for 30 min, twice in 2 × SSC/50% formamide at 65 °C for 30 min, and once in 1 ×
SSC/25% formamide:1 × Tris-buffered saline containing 0.1% Tween 20 (TBST) at room temperature (RT) for 30 min. Nonspecific binding probes were digested using 20 μg/ml RNase A in order to
reduce background signals. After RNase digestion at 37 °C for 30 min, the sections were placed in NTE buffer [500 mM NaCl, 10 mM Tris–HCl pH 8.0, 1 mM ethylenediamine tetraacetic acid
(EDTA)] at 37 °C for 5 min before being washed twice in 0.5× SSC at 65 °C for 20 min and then twice 1 × TBST at RT for 5 min. Then, the sections were in blocking solution (Roche) at room
temperature for 1 h. The sections were then incubated with the Fab fragment of an anti-DIG–alkaline phosphatase-conjugated antibody (Roche), diluted to 1:500 with blocking solution, for 1 h
at room temperature. Finally, each of the sections were rinsed twice in wash buffer for 5 min. The sections were then incubated in a NTMT solution (100 mM NaCl, 100 mM Tris–HCl pH 9.5, 50 mM
MgCl2, 0.1% Tween 20, 1 mM levamisole) containing 0.0035% of nitroblue tetrazolium (NBT; Roche) and 0.0018% of 5-bromo-4-chloro-3-indolyl phosphate (BCIP; Roche) at RT in the dark. The
resulting sections were observed under a BX51N-34FL microscope (Olympus). INTERSPECIFIC HYBRIDIZATION Cryopreserved sperm of bullet tuna, skipjack tuna, longtail tuna, ALT, and PBT were
produced by the dry shipper method37. A dry shipper (VOYAGEUR 5; Air Liquide, Paris, France) that had been completely filled with liquid nitrogen 1 day before sampling was used for sperm
cryopreservation. Semen collected from the sampled fish was diluted with an ice-cold cryomedium, consisting of 90% (vol/vol) 300 mM trehalose dihydrate (Wako, Osaka, Japan) and 10% (vol/vol)
dimethyl sulfoxide (Sigma-Aldrich, St. Louis, MO, USA), at a ratio of 1:20 (vol:vol). The diluted semen was equilibrated on ice for 10 min and dispensed into a 0.5 ml straw (Fujihara Kogyo,
Tokyo, Japan), which was then sealed with straw powder (Fujihara Kogyo). Up to eight straws containing diluted semen were placed in a goblet (PA003 13 mm diameter; My Science, Tokyo,
Japan), and up to two goblets were attached to a single cryocane (C-2; My Science) and frozen in the dry shipper. Cryopreserved sperm was transferred into a liquid nitrogen storage tank and
stored until use. Ovulated eggs were obtained from female ELT by gently squeezing the abdomen, and the eggs were collected into a 2,000 ml beaker. Straws containing cryopreserved sperm were
thawed by immersion in a 1-l beaker filled with tap water at ~25 °C for 10 s. ELT eggs were divided into two to four fractions. One fraction was mixed with 0.5 ml of fresh ELT sperm (3.0 ×
109–3.5 × 1010 sperm cells), and the other with 0.5 ml of cryopreserved sperm of other species (2.2 × 108–1.3 × 109 sperm cells). Inseminated eggs were activated by adding 1,000 ml of
natural seawater (25–27 °C). Eggs that rose to the water surface within 5 min after fertilization were defined as floating eggs, and triplicate lots of 30 floating eggs were collected into 8
ml Petri dishes filled with sterile seawater and incubated at 26 °C. The number of eggs that reached the four-cell stages within 1–2 h post-fertilization (HPF) and the embryonic body stages
within 13–14 HPF were recorded as the fertilized egg count and embryonic-body stage egg count, respectively. The number of larvae that hatched within 30–31 HPF was recorded as the hatched
egg count. PCR AND PLOIDY ANALYSIS For confirmation of the successful production of diploid HLTs, total genomic DNA was extracted from 10 HLT hatching larvae and subjected to PCR analyses.
All reference sequences with GenBank accession numbers, primer sequences, and PCR conditions in this study are summarized in Supplementary Tables 5–7. PCR amplification of parental nDNA was
performed using a primer set targeting the conserved region of the _ddx4_ gene flanking the ALT-specific deletion with TaKaRa Ex Taq (TaKaRa Bio, Tokyo, Japan). Identification of maternal
origin was performed using primer sets targeting species-specific single nucleotide polymorphisms (SNPs) on the _cytochrome c oxidase subunit I_ (_co1)_ gene in mtDNA with HiDi DNA
polymerase (my POLS Biotec, Konstanz, Germany). PCR products were electrophoresed on a 2.0% agarose gel together with 1 kb Plus DNA Ladder (New England Biolabs, Ipswich, MA, USA) as a
molecular weight marker. The relative DNA content of each larva was measured by flow cytometry using CyStain PI Absolute T Kit (Partec, Munster, Germany). Hatched larvae and sperm were
treated with nuclei extraction solution in CyStain PI Absolute T Kit for 30 min at room temperature to isolate nuclei and fixed them in 70% ethanol for at least 30 min. The nucleus
suspension was filtered through a nylon screen with a pore size of 42 µm to eliminate non-dissociated cell clumps. Approximately 1 × 106 of the nuclei were stained with 60 μg/ml propidium
iodide (PI) solution containing 300 μg/ml RNase A for 1 h at room temperature. The relative DNA content of resulting cells was measured using a Guava PCA‐96 (Millipore, Billerica, MA) with a
Guava cell cycle program in a Guava Cytosoft software (ver. 5.3). Flow cytometry was performed using nuclei of 10 HLT hatching larvae, control ELT hatching larvae, an ELT milt, and an ALT
milt. ELT larva was used to represent the standard DNA content value of the different sample types. GENOME-WIDE GENOTYPING The genome-wide genotyping of HLT was performed using genotyping by
random amplicon sequencing–direct (GRAS-Di)38, which is a nontargeted PCR-based genotyping system. Library preparation, sequencing, and genotyping were performed by Bioengineering Lab. Co.,
Ltd. (Kanagawa, Japan). A genotype library was prepared for pooled DNA of ELT (_n_ = 7), pooled DNA of ALT (_n_ = 5), and each DNA of 12 HLT individuals. The library was constructed in two
sequential PCR steps. In the first PCR, 63 different primers, consisting of the Illumina Nextera adaptor sequence and 3 bp random oligomers were used. In the second PCR, primers consisting
of the Illumina multiplexing 8 bp index and P7/P5 adapter sequence were used. The first PCR was performed in a 10 µl reaction volume containing 2 µl of 5 × PrimeStar buffer (Mg2+ plus;
TaKaRa Bio), 0.8 µl of dNTP mixture (2.5 mM each), 4 µl of random primer mix (100 μM), 0.1 µl of PrimeSTAR HS DNA polymerase (2.5 U/μl; TaKaRa Bio), 1 µl of genome DNA (6 ng/μl) and 2 µl of
sterile water. The reaction conditions were: initial denaturation at 98 °C for 2 min, and 30 cycles of 98 °C for 10 s, 50 °C for 15 s and 72 °C for 20 s. The second PCR was performed in a
10-µl reaction volume containing 2 µl of 5 × PrimeStar buffer (Mg2+ plus), 0.8 µl of dNTP mixture (2.5 mM each), 1 µl of P7 primer with 8-base index (10 μM), 1 µl of P5 primer with 8-base
index (10 μM), 0.1 µl of PrimeSTAR HS DNA polymerase (2.5 U/μl), 1.5 µl of five times diluted PCR product from the first PCR and 3.6 µl of sterile water. The reaction conditions were:
initial denaturation at 95 °C for 2 min, 25 cycles of 98 °C for 15 s, 55 °C for 15 s and 72 °C for 20 s, followed by a final extension period at 72 °C for 1 min. The final PCR products were
purified using a MiniElute PCR purification Kit (Qiagen, Hilden, Germany). The library was sequenced paired-end 2 × 150 bp on DNBSEQ-T7 (MGI Tech, Shenzhen, PRC) using the DNBSEQ-T7RS
High-throughput Sequencing Set (FCL PE150: MGI Tech). Adaptor sequences were clipped using cutadapt (ver. 4.0), and low-quality reads were trimmed using Sickle (ver. 1.33). Trimmed reads
<75 bp were removed. Moreover, sequences after 76 bases were deleted using fastx_trimmer (ver. 0.0.14). single nucleotide polymorphisms (SNP) calling for each individual used the
denovo_map.pl program of Stacks (ver. 2.62) with the -m 5 option to discard SNPs with read depth <5. The populations program of Stacks (ver. 2.62) was used with the -R 0.1 option to
extract SNPs found in >10% of the individuals in the overall sample. Approximately 1,700,000 raw reads were obtained per sample (deposited in the DDBJ SRA database; accession number:
PRJDB18157). After the foregoing analysis, 49,281 reference catalog sequences were obtained (Supplementary Data 2), and 36,727 SNP loci were found (Supplementary Data 3). To confirm that the
hybrid F1 was truly an interspecific hybrid between ELT and ALT, SNPs that provided relevant evidence were extracted from Supplementary Data 3 based on these criteria: (1) SNPs that were
homozygous in both parental species and had different alleles in each species, and (2) SNPs that had both alleles of the parent species in all analysed HLT except for undetected individuals.
SURVIVAL AND GROWTH TEST For analysis of the survival and growth characteristics of HLT, a rearing experiment was performed in three developmental stages (0–9 DAH, 9–14 DAH, and 14–18 DAH),
which were classified based on the feeding scheme (Fig. 3a). Larval rearing was conducted as described above. Feeding of the rotifer and live hatched larvae began at 2 and 9 DAH,
respectively. At the embryo-forming stage, an equal number of ELT and HLT embryos (6,500–10,000 embryos) were transferred into each of the three 100-l tanks. A survival rate at 9 DAH was
estimated using a 2,000 ml beaker. The larvae were sampled together with the surrounding water at three points in each rearing tank at night with strong aeration, and the total number was
counted. The survival rate was calculated as (the number of larvae sampled using the beaker/the volume of the surrounding water sampled together [2,000 ml]/the number of embryos at the
commencement of the rearing experiment × the volume of rearing water in the tank [100 l] × 100). At 9 DAH, 300 ELT and HLT larvae were transferred into each of the three 100-l tanks, and the
number of surviving fish was visually counted at 14 DAH. Next, at 14 DAH, 50 ELT and HLT larvae were transferred into each of the three 100-l tanks, and the number of surviving fish was
visually counted at 18 DAH. The survival rate was calculated as (the number of surviving/the number of larvae at the commencement of the rearing experiment × 100). Larval standard length was
measured at 9, 14, and 18 DAH on 10 randomly selected larvae. IHC Incorporation of donor PBT germ cells into HLT recipient gonads was analysed by IHC with the previously developed antibody
No. 149, which can specifically recognize bluefin tuna germ cells in the recipient gonad19,20. Seven days after transplantation, the gonads of the transplanted HLT recipient were fixed with
Bouin’s fixative overnight at 4 °C and then embedded in paraffin wax using standard methods. The paraffin block was sliced into 6-μm serial sections, which were subsequently dewaxed and
rehydrated through a xylene-ethanol series. After rehydration, an antigen retrieval treatment was performed using HistoVT One solution (Nacalai Tesque, Kyoto, Japan) at 90 °C for 20 min. For
prevention of nonspecific antibody binding, sections were blocked by PBST (PBS containing 0.1% Tween 20 [Sigma‒Aldrich]) containing 5% (w/v) Block-Ace (DS Farmer Biomedical, Osaka, Japan)
at room temperature for 1 h, after which sections were incubated with antibody No. 149 as a first primary antibody diluted 1000-fold in PBST containing 5% Block-Ace overnight at 4 °C.
Sections were washed three times for 5 min each with PBST. After washing, the sections were incubated for 1 h at room temperature with Alexa Fluor 488 goat anti-mouse IgM (H + L) (Thermo
Fisher Scientific), which was diluted 200-fold with Can Get Signal immunostain solution A (Toyobo), as the secondary antibody solution against antibody No. 149. Then, sections were washed
three times for 5 min each with PBST and incubated with anti-Ddx4 antibody as a second primary antibody diluted 100-fold with Can Get Signal immunostain solution B (Toyobo) at room
temperature for 1 h. Sections were washed three times for 5 min each with PBST. After washing, the sections were incubated for 1 h at room temperature with Alexa Fluor 555 goat anti-rabbit
IgG (H + L) (Thermo Fisher Scientific), which was diluted 200-fold with Can Get Signal immunostain solution A, as the secondary antibody solution against the anti-Ddx4 antibody. Following
immunoreactions, the sections were washed three times with PBST for 5 min each and then observed under a fluorescence microscope (BX51N-34FL; Olympus). The incorporation rate of
donor-derived PBT germ cells in recipient genital ridges was calculated as (number of fish incorporating antibodies No. 149 and anti-Ddx4 double-positive germ cells in gonad/number of fish
observed × 100). Following observation, the slides were counterstained with haematoxylin and eosin. Type A spermatogonia in the donor PBT testis was detected by IHC using anti-Ddx4 antibody
and the previously developed No. 152 antibody19. The donor PBT testes were fixed in 4% paraformaldehyde/PBS for 12–14 h at 4 °C. IHC was performed as described above. No. 152 and anti-Ddx4
antibodies, diluted 200-fold and 100-fold with Can Get Signal immunostain solution B, respectively, were used as the primary antibodies. Fluor 488 goat anti-mouse IgG (H + L) (Thermo Fisher
Scientific) and Alexa Fluor 555 goat anti-rabbit IgG (H + L), both diluted 200-fold with Can Get Signal immunostain solution A, were used as the secondary antibodies. Following IHC, the
sections were observed under a confocal laser-scanning microscope (FV 10i; Olympus). GENERATION OF PBT-SPERM-SPECIFIC ANTIBODIES In addition to antibody No. 149, we generated another
monoclonal antibody for the specific detection of donor PBT sperm in HLT recipient milt. To generate monoclonal antibodies, PBT sperm were inoculated into six female BALB/c mice19,20,39.
Each mouse was injected with 100 μl of emulsion containing equal volumes of PBS and Complete Freund’s Adjuvant (Merck KGaA, Darmstadt, Germany), followed by ~5.0 × 106 cells. This injection
was performed ten times at 2 week intervals. Eight days after the final inoculation, the lymph nodes were removed from the mice and the lymph node cells were recovered. To generate
hybridomas, the lymph node cells were fused with mouse myeloma cells of the P3U1 line in the presence of 50% PEG 4000 (Merck-Millipore, Billerica, MA, USA)/RPMI medium (Merck KGaA). The
fused cells were plated on six 96-well plates and cultured with Hybridoma-SFM (Thermo Fisher Scientific) supplemented with 15% FBS (Hyclone; GE healthcare), 1% HT Supplement (Thermo Fisher
Scientific), 0.2 μg/ml of aminopterin (Merck KGaA), 1% BM-Condimed H1 (Merck KGaA), and antibiotics (100 U/ml of penicillin and 100 μg/ml of streptomycin) in a 37 °C incubator with 5% CO2.
After 3 days, the medium was changed to Hybridoma-SFM supplemented with 15% FBS (Hyclone), 1% HT Supplement (Thermo Fisher Scientific), and antibiotics (100 U/ml of penicillin and 100 μg/ml
of streptomycin). The supernatants from these wells were then subjected to screening for antibody secretion and reactivity to the PBT sperm. The selected hybridoma cells were expanded and
subclones were established by limiting dilution. The hybridoma cells were then cultured with Hybridoma-SFM supplemented with 10% FBS and antibiotics (100 U/ml of penicillin and 100 μg/ml of
streptomycin) in a 37 °C incubator with 5% CO2. As a result, we obtained 907 hybridoma clones. Screening for antibodies that could specifically recognize the PBT sperm but not HLT sperm was
performed using cell-based ELISA. Approximately 5 × 105 cells of PBT sperm or HLT sperm in 100 μl PBS containing 0.5% bovine serum albumin (BSA) and 2 mM ethylenediaminetetraacetic acid
(EDTA) were dispensed in each well of twelve 96-well plates and the supernatant was removed. Next, 50 μl of primary antibodies isolated from the hybridomas were added to each well and the
cells were incubated for 30 min at room temperature. Following immunoreaction, the cells were washed three times with PBS containing 0.5% BSA and 2 mM EDTA. Then, 50 μl of anti-IgG (H + L
chain) (Mouse) pAb-HRP (MBL, Aichi, Japan) was diluted 10000-fold in HEPES buffer (MBL), added to each well as a secondary antibody, and incubated for 30 min at room temperature. Following
immunoreaction, the cells were again washed three times with PBS containing 0.5% BSA and 2 mM EDTA, and 50 μl of 3,5,3,5-tetramethylbenzidine (TMB) peroxidase substrate (MBL) was added to
each well. The plates were then incubated for 10 min at room temperature, following which color development was stopped by adding 50 μl of 1.5 N phosphoric acid to each well. The absorbance
in each well was measured at 450 nm. As a result, we found 10 antibodies that had a strong affinity for PBT sperm cells but not for HLT sperm. Furthermore, we selected an antibody (No. 102B)
that could specifically recognize PBT sperm but not HLT sperm by secondary screening using ICC against PBT and HLT sperm as described below. ICC Milts were obtained from 3 year-old PBT (BW
~40–60 kg, _n_ = 3), nontransplanted 12 month-old HLT (FL 43.5 ± 0.7 cm, BW 1848 ± 81.9 g, GW 42 ± 9.9 g, _n_ = 3), and transplanted 8–12 month-old HLT recipients (FL 41.3 ± 0.4 cm, BW
1500.3 ± 43.1 g, GW 26.5 ± 2.8 g, _n_ = 68). Milts were cryopreserved in a cryomedium consisting of 90% FBS and 10% DMSO until ICC analysis as described above37. After thawing, 100 million
sperm were fixed with 50 µl of Tissue-Tek U-fix (Sakura Finetech, Tokyo, Japan) for 5 min at room temperature and diluted with 250 µl of PBS (-). Sperm were smeared on glass slides (Frontier
FRC-05: Matsunami Grass) using disposable cytofunnels (Epredia Double Cytofunnel: Thermo Fisher Scientific) with a Cytospin 4 Cytocentrifuge (Thermo Fisher Scientific) operated at 113 x g
for 5 min and air-dried for 30 min at room temperature. Sperm smears were washed with distilled water for 5 min and then with PBST for 5 min. Sperm smears were blocked with PBST containing
5% Block-Ace at room temperature for 1 h, followed by incubation with antibody No. 149 as a first primary antibody diluted 5000-fold in PBST containing 5% Block-Ace overnight at 4 °C. Sperm
smears were washed three times for 5 min each with PBST. After washing, the sperm smears were incubated for 1 h at room temperature with Alexa Fluor 488 goat anti-mouse IgM (H + L), which
was diluted 200-fold with Can Get Signal immunostain solution A, as the secondary antibody solution against antibody No. 149. Then, sperm smears were washed three times for 5 min each with
PBST and incubated with Alexa 594-conjugated antibody 102B as a secondary primary antibody diluted 10-fold with PBST containing 5% Block-Ace at room temperature for 1 h. After
immunoreaction, sperm smears were counterstained with 4’,6-diamidino-2-phenylindole (DAPI) and then observed under a fluorescence microscope. To compare the sperm morphology, the long and
short diameters of the sperm head were measured using the Image J software application (ver. 1.54i). ESTIMATION OF GERM CELL DIVISION NUMBER We estimated whether incorporated PBT
spermatogonia showed stem cell activity by predicting the proliferation rate. The cell division number of PBT and HLT spermatogonium to reach sperm was assessed21. Mature testes of PBT and
HLT were fixed in Bouin’s fixative, dehydrated, and embedded in paraffin wax. The specimens were then cut into 5-μm sections, and the hydrated sections were stained with haematoxylin and
eosin solution. We counted the number of spermatids at the largest diameter cyst among the serial sections and calculated the mean spermatid count in 10 cysts. For estimation of the cell
number in cysts, this mean value was then compared with the clay simulation model21. The total donor-derived PBT sperm number produced by HLT recipients was estimated by multiplying the
existence ratio of donor-derived PBT sperm, the sperm concentration of recipient milt, and the total milt volume. PROGENY TEST Fifteen 10 month-old HLT recipients were induced to spawn by
administering GnRHa as described above. Fertilized eggs were introduced into 8-l tanks and incubated with flowthrough seawater at 24–25 °C with gentle aeration for 12 h. For removal of
unfertilized eggs and dead eggs sinking in the bottom of the tank, only floating eggs that reached the embryonic body stage were collected using a hand net and stored in 70% ethanol at −20
°C until DNA extraction. These embryos were divided into microtubes every 100 embryos and mashed using a disposable homogenizer (Bio masher II, Nippi, Tokyo, Japan). Total genomic DNA was
extracted from these pooled embryos. PCR amplification was performed using PBT-specific primer sets targeting _ddx4_, _dnd_, one microsatellite locus40, and the _d-loop_ with AmpliTaq Gold
DNA polymerase (Applied Biosystems, Waltham, MA). Primer sequences and PCR conditions are listed in Supplementary Tables 6 and 7. PCR products were electrophoresed on a 2.0% agarose gel.
STATISTICAL ANALYSIS All data are presented as the mean value ± standard error of the mean (SEM). Statistical significance was determined using two-sided Student’s _t_ test for comparisons
between two groups and one-way ANOVA for multiple group comparisons. All analyses were carried out using GraphPad Prism version 5.0 (GraphPad Software, CA, USA). REPORTING SUMMARY Further
information on research design is available in the Nature Portfolio Reporting Summary linked to this article. DATA AVAILABILITY The main data supporting the results of this study are
available within the paper and supplementary information. The raw sequence data generated by GRAS-Di have been deposited in the the DDBJ BioProject database under BioProject accession number
PRJDB18157. The GenBank accession numbers of reference sequences for generating primers are listed in Supplementary Table 5. All unique materials used are available from the authors on
reasonable request. Source data are provided with this paper. REFERENCES * Collette, B. B. et al. High value and long life-double jeopardy for tunas and billfishes. _Science_ 333, 291–292
(2011). Article ADS CAS PubMed Google Scholar * Juan-Jordá, M. J., Mosqueira, I., Cooper, A. B., Freire, J. & Dulvy, N. K. Global population trajectories of tunas and their
relatives. _Proc. Natl Acad. Sci. USA_ 108, 20650–20655 (2011). Article ADS PubMed PubMed Central Google Scholar * ISC. _Report of the Pacific Bluefin Tuna Working Group. The Annex 13
of 15 ISC Final Report. Stock Assessment of Pacific Bluefin Tuna in the Pacific Ocean in 2022._ https://isc.fra.go.jp/pdf/ISC22/ISC22_ANNEX13_Stock_Assessment_for_Pacific_Bluefin_Tuna
(2022). * Sawada, Y., Okada, T., Miyashita, S., Murata, O. & Kumai, H. Completion of the Pacific bluefin tuna _Thunnus orientalis_ (Temminck et Schlegel) life cycle. _Aquac. Res._ 36,
413–421 (2005). Article Google Scholar * Ottolenghi, F. Capture-based aquaculture of bluefin tuna. In: _Capture-Based Aquaculture. Global Overview_. (eds Lovatelli, A. & Holthus P. F.)
169–182 (FAO, 2008). * Metian, M., Pouil, S., Boustany, A. & Troell, M. Farming of bluefin tuna–reconsidering global estimates and sustainability concerns. _Rev. Fish. Sci. Aquac._ 22,
184–192 (2014). Article Google Scholar * Benetti, D. D., Partridge, G. J. & Stieglitz, J. Overview on status and technological advances in tuna aquaculture around the world. In
_Advances in Tuna Aquaculture_ (eds Benetti, D. D., Partridge, G. J. & Buentello, A.) 1–19 (Academic Press, 2016). * Seoka, M. et al. Gonadal maturation of Pacific bluefin tuna _Thunnus
orientalis_ in captivity. _Aquac. Sci._ 55, 289–292 (2007). Google Scholar * Masuma, S., Miyahita, S., Yamamoto, H. & Kumai, H. Status of bluefin tuna farming, broodstock management,
breeding and fingerling production in Japan. _Rev. Fish. Sci._ 16, 385–390 (2008). Article Google Scholar * Masuma, S., Takebe, T. & Sakakura, Y. A review of the broodstock management
and larviculture of the Pacific northern bluefin tuna in Japan. _Aquaculture_ 315, 2–8 (2011). Article Google Scholar * Yoshizaki, G. & Yazawa, R. Application of surrogate broodstock
technology in aquaculture. _Fish. Sci._ 85, 429–437 (2019). Article CAS Google Scholar * Houston, R. D. et al. Harnessing genomics to fast-track genetic improvement in aquaculture. _Nat.
Rev. Genet._ 21, 389–409 (2020). Article CAS PubMed Google Scholar * Okutsu, T., Suzuki, K., Takeuchi, Y., Takeuchi, T. & Yoshizaki, G. Testicular germ cells can colonize sexually
undifferentiated embryonic gonad and produce functional eggs in fish. _Proc. Natl Acad. Sci. USA_ 103, 2725–2729 (2006). Article ADS CAS PubMed PubMed Central Google Scholar *
Takeuchi, Y., Yoshizaki, G. & Takeuchi, T. Surrogate broodstock produces salmonids. _Nature_ 430, 629–630 (2004). Article ADS CAS PubMed Google Scholar * Okutsu, T., Shikina, S.,
Kanno, M., Takeuchi, Y. & Yoshizaki, G. Production of trout offspring from triploid salmon parents. _Science_ 317, 1517 (2007). Article ADS CAS PubMed Google Scholar * Yazawa, R. et
al. Eastern little tuna, _Euthynnus affinis_ (Cantor, 1849) mature and reproduce within 1 year of rearing in land-based tanks. _Aquac. Res._ 47, 3800–3810 (2016). Article CAS Google
Scholar * Yazawa, R. et al. Production of triploid eastern little tuna, _Euthynnus affinis_ (Cantor, 1849). _Aquac. Res._ 50, 1422–1430 (2019). Article CAS Google Scholar * Kawamura, W.
et al. Suitability of hybrid mackerel (_Scomber australasicus_ x _S. japonicus_) with germ cell-less sterile gonads as a recipient for transplantation of bluefin tuna germ cells. _Gen. Comp.
Endocrinol._ 295, 113525 (2020). Article CAS PubMed Google Scholar * Ichida, K. et al. Specific visualization of live type A spermatogonia of Pacific bluefin tuna using fluorescent
dye-conjugated antibodies. _Biol. Reprod._ 100, 1637–1647 (2019). Article PubMed Google Scholar * Yazawa, R. et al. Establishment of a tracing technique for transplanted bluefin tuna germ
cells in recipient’s gonads using monoclonal antibodies specifically recognizing bluefin tuna spermatogenic cells. _Fish. Sci._ 87, 105–112 (2021). Article CAS Google Scholar * Ando, N.,
Miura, T., Nader, M., Miura, C. & Yamauchi, K. A method for estimating the number of mitotic divisions in fish testes. _Fish. Sci._ 66, 299–303 (2000). Article CAS Google Scholar *
Yazawa, R. et al. Spawning induction and seed production of eastern little tuna, _Euthynnus affinis_ (Cantor, 1849), in the post- and pre-spawning seasons by hormonal treatment in a
semi-closed recirculation system with elevated temperature. _Aquac. Res._ 48, 3472–3481 (2017). Article CAS Google Scholar * Miya, M. et al. Evolutionary origin of the Scombridae (tunas
and mackerels): members of a paleogene adaptive radiation with 14 other pelagic fish families. _PLoS One_ 8, e73535 (2013). Article ADS CAS PubMed PubMed Central Google Scholar *
Diaz-Arce, N., Arrizabalaga, H., Murua, H., Irigoien, X. & Rodriguez-Ezpeleta, N. RAD-seq derived genome-wide nuclear markers resolve the phylogeny of tunas. _Mol. Phyl. Evol._ 102,
202–207 (2016). Article Google Scholar * Graham, J. B. & Dickson, K. A. The evolution of thunniform locomotion and heat conservation in scombrid fishes: New insights based on the
morphology of _Allothunnus fallai_. _J. Linn. Soc. Lond., Zool._ 129, 419–466 (2000). Article Google Scholar * Gonzalez, R. & Dobrinski, I. Beyond the mouse monopoly: studying the male
germ line in domestic animal models. _ILAR J._ 56, 83–98 (2015). Article CAS PubMed PubMed Central Google Scholar * Ashida, H., Suzuki, N., Tanabe, T., Suzuki, N. & Aonuma, Y.
Reproductive condition, batch fecundity, and spawning fraction of large Pacific bluefin tuna _Thunnus orientalis_ landed at Ishigaki Island, Okinawa, Japan. _Environ. Biol. Fish._ 98,
1173–1183 (2014). Article Google Scholar * Okochi, Y., Abe, O., Tanaka, S., Ishihara, Y. & Shimizu, A. Reproductive biology of female Pacific bluefin tuna, _Thunnus orientalis_, in the
Sea of Japan. _Fish. Res._ 174, 30–39 (2016). Article Google Scholar * Yoneda, M. et al. Maternal spawning experience and thermal effects on offspring viability of chub mackerel and their
influence on reproductive success. _Front. Mar. Sci._ 9, 2701 (2022). Article Google Scholar * Neira, F. J. & Keane, J. P. Ichthyoplankton-based spawning dynamics of blue mackerel
(Scomber australasicus) in south-eastern Australia: links to the East Australian current. _Fish. Oceanogr._ 17, 281–298 (2008). Article Google Scholar * Yoshizaki, G. et al. Production of
germ cell-deficient salmonids by dead end gene knockdown, and their use as recipients for germ cell transplantation. _Mol. Reprod. Dev._ 83, 298–311 (2016). Article CAS PubMed Google
Scholar * Franěk, R. et al. Who is the best surrogate for germ stem cell transplantation in fish? _Aquaculture_ 549, 737759 (2022). Article Google Scholar * Yazawa, R. et al.
GnRHa-induced spawning of the Eastern little tuna (_Euthynnus affinis_) in a 70-m3 land-based tank. _Aquaculture_ 442, 58–68 (2015). Article CAS Google Scholar * Amezawa, K., Yazawa, R.,
Takeuchi, Y. & Yoshizaki, G. Spawning induction of blue mackerel _Scomber australasicus_ and eastern little tuna _Euthynnus affinis_ by oral administration of a crude
gonadotropin-releasing hormone analogue. _Fish. Sci._ 84, 495–504 (2018). Article CAS Google Scholar * Takeuchi, Y., Higuchi, K., Yatabe, T., Miwa, M. & Yoshizaki, G. Development of
spermatogonial cell transplantation in Nibe croaker, _Nibea mitsukurii_ (Perciformes, Sciaenidae). _Biol. Reprod._ 81, 1055–1063 (2009). Article CAS PubMed Google Scholar * Nagasawa, K.
et al. cDNA cloning and expression analysis of a _vasa_-like gene in Pacific bluefin tuna _Thunnus orientalis_. _Fish. Sci._ 75, 71–79 (2008). Article Google Scholar * Kawamura, W. et al.
Development of a simple method for sperm cryopreservation of Scombridae fishes in outdoor environments. _Aquac. Res._ 51, 3376–3383 (2020). Article CAS Google Scholar * Hosoya, S. et al.
Random PCR-based genotyping by sequencing technology GRAS-Di (genotyping by random amplicon sequencing, direct) reveals genetic structure of mangrove fishes. _Mol. Ecol. Resour._ 19,
1153–1163 (2019). Article CAS PubMed Google Scholar * Hayashi, M. et al. Establishment of novel monoclonal antibodies for identification of type A spermatogonia in teleosts. _Biol.
Reprod._ 101, 478–491 (2019). Article PubMed Google Scholar * Appleyard, S., Grewe, P., Innes, B. & Ward, R. Population structure of yellowfin tuna (_Thunnus albacares_) in the
western Pacific ocean, inferred from microsatellite loci. _Mar. Biol._ 139, 383–393 (2001). Article CAS Google Scholar Download references ACKNOWLEDGEMENTS This work was partly supported
by a Grant-in-Aid for Scientific Research (KAKENHI) on Innovative Areas, Establishment of gamete integrity (18H05545) and Sophistication of technologies to secure living marine resources
(JPMXD0511102331) from MEXT (to G.Y.). AUTHOR INFORMATION Author notes * These authors contributed equally: Wataru Kawamura, Ryosuke Yazawa, Yutaka Takeuchi. AUTHORS AND AFFILIATIONS *
Institute for Reproductive Biotechnology for Aquatic Species, Tokyo University of Marine Science and Technology, 4-5-7 Konan, Minato-ku, Tokyo, 108-8477, Japan Wataru Kawamura, Ryosuke
Yazawa, Kensuke Ichida, Tetsuro Morita, Makoto Hayashi & Goro Yoshizaki * Department of Marine Biosciences, Tokyo University of Marine Science and Technology, 4-5-7 Konan, Minato-ku,
Tokyo, 108-8477, Japan Ryosuke Yazawa, Shigeharu Kamio & Goro Yoshizaki * Faculty of Biological Science and Technology, Kanazawa University, Kakuma, Kanazawa, Ishikawa, 920-1192, Japan
Yutaka Takeuchi * Salmonid Experimental Station at Campos do Jordão, Unidade de Pesquisa e Desenvolvimento-Campos do Jordão, Agência Paulista de Tecnologia dos Agronegócios, Secretaria de
Agricultura, São Paulo, 12460-000, Brazil Ricardo Shohei Hattori Authors * Wataru Kawamura View author publications You can also search for this author inPubMed Google Scholar * Ryosuke
Yazawa View author publications You can also search for this author inPubMed Google Scholar * Yutaka Takeuchi View author publications You can also search for this author inPubMed Google
Scholar * Shigeharu Kamio View author publications You can also search for this author inPubMed Google Scholar * Kensuke Ichida View author publications You can also search for this author
inPubMed Google Scholar * Ricardo Shohei Hattori View author publications You can also search for this author inPubMed Google Scholar * Tetsuro Morita View author publications You can also
search for this author inPubMed Google Scholar * Makoto Hayashi View author publications You can also search for this author inPubMed Google Scholar * Goro Yoshizaki View author publications
You can also search for this author inPubMed Google Scholar CONTRIBUTIONS W.K., R.Y., Y.T., S.K., K.I., R.S.H., and M.H. conducted experiments and analysed the data. W.K., R.Y., Y.T., T.M.,
and G.Y. designed the research. W.K., R.Y. and G.Y. conceived the study and wrote the manuscript. All authors critically revised the report, commented on drafts of the manuscript, and
approved the final report. CORRESPONDING AUTHOR Correspondence to Goro Yoshizaki. ETHICS DECLARATIONS COMPETING INTERESTS The authors declare no competing interests. PEER REVIEW PEER REVIEW
INFORMATION _Nature Communications_ thanks the anonymous reviewers for their contribution to the peer review of this work. A peer review file is available. ADDITIONAL INFORMATION PUBLISHER’S
NOTE Springer Nature remains neutral with regard to jurisdictional claims in published maps and institutional affiliations. SUPPLEMENTARY INFORMATION SUPPLEMENTARY INFORMATION PEER REVIEW
FILE DESCRIPTION OF ADDITIONAL SUPPLEMENTARY FILES SUPPLEMENTARY DATA 1 SUPPLEMENTARY DATA 2 SUPPLEMENTARY DATA 3 REPORTING SUMMARY SOURCE DATA SOURCE DATA RIGHTS AND PERMISSIONS OPEN ACCESS
This article is licensed under a Creative Commons Attribution-NonCommercial-NoDerivatives 4.0 International License, which permits any non-commercial use, sharing, distribution and
reproduction in any medium or format, as long as you give appropriate credit to the original author(s) and the source, provide a link to the Creative Commons licence, and indicate if you
modified the licensed material. You do not have permission under this licence to share adapted material derived from this article or parts of it. The images or other third party material in
this article are included in the article’s Creative Commons licence, unless indicated otherwise in a credit line to the material. If material is not included in the article’s Creative
Commons licence and your intended use is not permitted by statutory regulation or exceeds the permitted use, you will need to obtain permission directly from the copyright holder. To view a
copy of this licence, visit http://creativecommons.org/licenses/by-nc-nd/4.0/. Reprints and permissions ABOUT THIS ARTICLE CITE THIS ARTICLE Kawamura, W., Yazawa, R., Takeuchi, Y. _et al._
Bluefin tuna sperm production is hastened by surrogacy in small _Euthynnus_. _Nat Commun_ 15, 8128 (2024). https://doi.org/10.1038/s41467-024-52393-4 Download citation * Received: 15 January
2024 * Accepted: 04 September 2024 * Published: 03 October 2024 * DOI: https://doi.org/10.1038/s41467-024-52393-4 SHARE THIS ARTICLE Anyone you share the following link with will be able to
read this content: Get shareable link Sorry, a shareable link is not currently available for this article. Copy to clipboard Provided by the Springer Nature SharedIt content-sharing
initiative