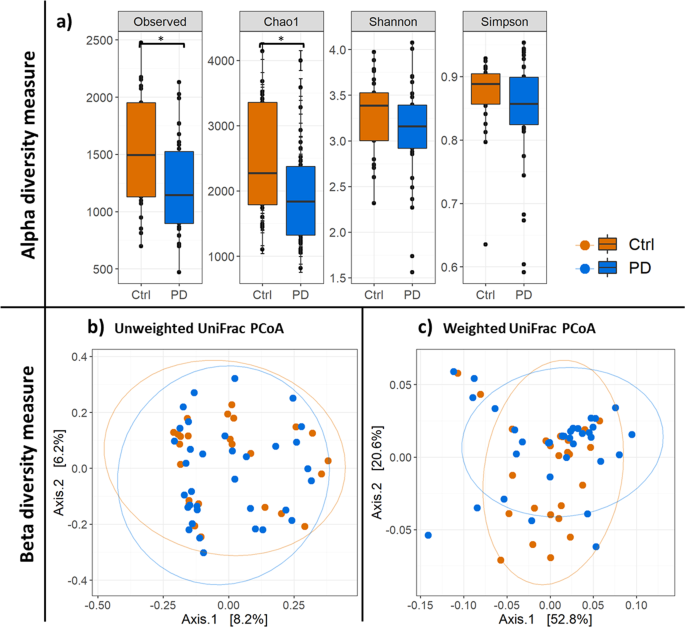
Effect of parkinson’s disease and related medications on the composition of the fecal bacterial microbiota
- Select a language for the TTS:
- UK English Female
- UK English Male
- US English Female
- US English Male
- Australian Female
- Australian Male
- Language selected: (auto detect) - EN
Play all audios:

ABSTRACT Parkinson’s disease (PD) is one of the most common neurodegenerative disorders. PD patients suffer from gastrointestinal dysfunctions and alterations of the autonomous nervous
system, especially its part in the gut wall, i.e., the enteric nervous system (ENS). Such alterations and functional gastrointestinal deficits often occur years before the classical clinical
symptoms of PD appear. Until now, only little is known about PD-associated changes in gut microbiota composition and their potential implication in PD development. In order to increase
knowledge in this field, fecal samples of 34 PD patients and 25 healthy, age-matched control persons were investigated. Here, the V4 and V5 hypervariable region of bacterial 16S rRNA genes
was PCR-amplified and sequenced using an Ion Torrent PGM platform. Within the PD group, we observed a relative decrease in bacterial taxa which are linked to health-promoting,
anti-inflammatory, neuroprotective or other beneficial effects on the epithelial barrier, such as _Faecalibacterium_ and _Fusicatenibacter_. Both taxa were lowered in PD patients with
elevated levels of the fecal inflammation marker calprotectin. In addition, we observed an increase in shares of the _Clostridiales_ family XI and their affiliated members in these samples.
Finally, we found that the relative abundances of the bacterial genera _Peptoniphilus, Finegoldia, Faecalibacterium Fusicatenibacter, Anaerococcus, Bifidobacterium, Enterococcus_, and
_Ruminococcus_ were significantly influenced by medication with L-dopa and entacapone, respectively. Our data confirm previously reported effects of COMT inhibitors on the fecal microbiota
of PD patients and suggest a possible effect of L-dopa medication on the relative abundance of several bacterial genera. SIMILAR CONTENT BEING VIEWED BY OTHERS FECAL MICROBIOME ALTERATIONS
IN TREATMENT-NAIVE DE NOVO PARKINSON’S DISEASE Article Open access 10 October 2022 ASSOCIATION BETWEEN PARKINSON’S DISEASE AND THE FAECAL EUKARYOTIC MICROBIOTA Article Open access 18
November 2021 GUT BACTERIAL TYROSINE DECARBOXYLASE ASSOCIATES WITH CLINICAL VARIABLES IN A LONGITUDINAL COHORT STUDY OF PARKINSONS DISEASE Article Open access 15 December 2021 INTRODUCTION
Parkinson’s disease (PD) is one of the most common neurodegenerative disorders. So far, there is no causal treatment for PD that is able to halt the neurodegenerative process.1 Besides motor
and cognitive symptoms, most PD patients suffer from gastrointestinal (GI) symptoms, such as constipation or prolonged intestinal transit time.2,3,4,5 These symptoms can occur several years
ahead of classical motor symptoms, which gives rise to the hypothesis that the enteric nervous system (ENS) becomes compromised before the central nervous system.6,7,8 These observations
support the hypothesis that PD may begin in the GI tract.9 The ENS is a complex of several networks of neurons, glial cells, and interconnecting fibers within the mammal GI tract. The ENS
communicates with the brain bidirectionally via the vagus nerve as the hard-wired section of the so called brain-gut axis.10 Experimental data suggest that neurons and fibers from the ENS
and vagus nerve provide a neuronal chain, that allow pathological peptides to travel between the gut and the brain in a prion-like way and modulate the course of neurological
diseases.11,12,13 Recent studies indicate, that the pathological process of PD alongside the gut-brain-axis might be modulated or even initiated by the gut microbiota.14,15 Indicators are
fecal markers of gut inflammation and permeability, which are increased in PD.16 Furthermore, bacterial metabolites, which may have an influence on the ENS, differ between PD patients and
healthy controls.16,17 Clearly, the cause-effect relationship between the intestinal microbiota composition and its metabolic capacity on the one hand and PD pathogenesis on the other hand
are still obscure. Constipation and reduced gut motility in PD may be important triggers to alter the microbiota composition.18,19 In addition, previous studies also suggested that some PD
medication may alter the microbiota composition.20,21,22 L-dopa (L-3,4-Dihydroxyphenylalanine, levodopa) application targets the striatal dopamine deficiency in PD patients and may stimulate
the dopamine transporter on the terminal nerve.23,24 Long term therapy with L-dopa is known to induce side effects like increased inflammation and oxidative stress.23
Catechol-O-methyltransferases (COMT) are able to methylate L-dopa rendering it ineffective.25,26 COMT are able to methylate a wide range of catechols and thereby eliminate biologically
active or toxic molecules27 including L-dopa.25,26,28 Entacapone is a COMT inhibitor28 preventing the degradation and increasing the plasma availability of L-dopa.28,29 To the best of our
knowledge, it is still unknown in which mechanistic way these drugs may alter gut microbiota composition and/or functionality. Increasing evidence indicates a difference in fecal microbiota
composition between PD and healthy controls, irrespective of the applied method.17,22,30,31,32 The methods used so far were quantitative real-time PCR (qPCR) to detect highly abundant
bacterial taxa,17 pyrosequencing of the V4 variable region of the 16S rRNA gene14 and Illumina MiSeq sequencing of the V1 and V2 region32 or V4 region.22 Other studies addressed the
microbial and viral gut metagenome in the early stage of PD33 in L-dopa naïve patients with shotgun sequencing methods.34 Correlations between a medication with entacapone and the (relative)
abundances of distinct bacterial taxa were previously reported,17,22,30 suggesting PD medication as an important influencing factor for the PD microbiota. However, to the best of our
knowledge no study reported medication with L-dopa as an influencing factor so far. In this study, we used next generation sequencing to screen for difference in fecal microbiota composition
between PD patients and matched controls. We analyzed the same set of samples previously detailed by Unger et al.,17 albeit with Ion Torrent-based next generation sequencing of the V4 and
V5 region of the bacterial 16S rRNA gene instead of qPCR. While qPCR is suitable for the quantitative determination of distinct bacterial taxa, it is also limited to known sequence types. We
believe that monitoring differences in fecal microbiota composition in PD patients compared to suitable controls is a first step to elucidate whether the gut microbiota might play any
functional role in PD pathogenesis. Clearly, such differences might also depend on factors such as the type of PD medication. In the long run, microorganisms which differ significantly
between healthy persons and PD patients (independent on factors such as medication) might play an indicative role in early PD diagnosis. This may increase our knowledge on the
aetiopathogenesis of PD. RESULTS SEQUENCING AND BIOINFORMATICS Four combined sequencing datasets yielded 11,752,187 partial bacterial 16S rRNA gene sequences with a mean of 199,190 sequences
per sample (min: 70,225; max: 394,784 sequences). Following exclusion of sequences that were present in less than 10% of all samples, 12,935 OTUs affiliated with 192 genera, 55 families, 28
orders, 17 classes, and 8 phyla were identified. Searching for interdependencies among the sample attributes, CramérV contingency coefficients did not show strong association (cV >
0.7)35 and no significant _Χ_² _p_-values were detected between most of the sample attributes. Only, L-dopa medication showed a significance in the _Χ_²-test (_p_ = 0.048) and a medium
strong association with the Hoehn–Yahr stage (cV = 0.53). According to the Spearman-_Ρ_, the Hoehn–Yahr stage was significantly positively correlated with disease duration (_p_ = 0.0016; _Ρ_
= 0.5183). The numbers of samples per investigated patient or control subgroup are summarized in Table 1. Important metadata are detailed in the Supplementary Table 1. STRUCTURAL DIVERSITY
MEASURES Alpha diversity indices (Fig. 1a) revealed a significant decrease in bacterial diversity in PD patients compared to the control (Ctrl) regarding observed species (_p_Observed =
0.032) and estimated species (_p_Chao1 = 0.032). Shannon and Simpson metrics for alpha diversity did not show significant differences between PD and control (_p_Shannon = 0.197, _p_Simpson =
0.197). Non-parametric multivariate analysis of variance (ADONIS), calculated for the beta diversity of PD microbiota and the control microbiota (Fig. 1b + c), revealed that neither the
weighted (_p_weighted-UniFrac = 0.249) nor the unweighted (_p_unweighted UniFrac = 0.226) UniFrac measure were significantly different between the PD and the control group. DIFFERENCES IN
MICROBIOTA COMPOSITION One bacterial family and three genera were found to be significantly different in relative abundance between PD and controls (_p_ < 0.05). The _Clostridiales_
family XI and the genus _Peptoniphilus_ were observed at higher relative abundances in the PD dataset. The genera _Faecalibacterium_ and _Fusicatenibacter_ decreased relatively in PD (Table
2). In the control group, the family _Bifidobacteriaceae_ and the genus _Bifidobacterium_ were relatively increased within the female controls in comparison to the male controls. In the PD
group, no taxon was found to be significantly different in relative abundance between male and female patients. In the female samples, a significant increase in the relative abundance of the
_Clostridiales_ family XI and the genus _Peptoniphilus_ was observed. Furthermore, a decrease in abundance for _Faecalibacterium_ in PD was observed. Within the male samples with PD, the
family of _Bifidobacteriaceae_ was significantly increased in relative abundance. PD patients with elevated levels of fecal calprotectin16,17 showed a significant increase in the relative
abundance of the _Clostridiales_ family XI, the genera _Peptoniphilus_ and _Finegoldia_, and significantly decreased relative abundances for _Faecalibacterium_ and _Fusicatenibacter_ when
compared to calprotectin negative controls. PD patients with normal calprotectin levels showed an increased relative abundance of _Peptoniphilus_ in comparison to controls. In addition,
there was an increase in relative abundance for _Faecalibacterium_ and decreased relative abundance for the genus _Streptococcus_ and the family _Streptococcaceae_ when compared to
calprotectin positive PD patients. PD patients treated with L-dopa showed significantly higher relative abundances of _Enterococcaceae_, _Clostridiales_ family XI, and the genera
_Peptoniphilus_ and _Finegoldia_, while _Faecalibacterium_ and the _Ruminococcus gauvreauii_ group were significantly decreased when compared to the controls. However, there were no
significant differences on genus or family levels between PD patients who were not treated with L-dopa and the control group or the PD patients treated with L-dopa. PD patients treated with
entacapone showed significantly higher relative abundances of the families _Enterococcaceae, Bifidobacteriaceae_, and the _Clostridiales_ family XI, as well as of the genera _Peptoniphilus,
Anaerococcus_, the _Eubacterium brachy_ group, _Sellimonas, Bifidobacterium_, and _Enterococcus_, when compared to the controls. However, the _Ruminococcus gauvreauii_ group and the genus
_Faecalibacterium_ were significantly decreased in this relation. PD patients with a hypokinetic-rigid (HR) phenotype showed a significantly increased relative abundance of _Peptoniphilus_
and decreased relative abundance of _Faecalibacterium_ compared to the control group, while PD patients with a tremor dominant (T) phenotype showed a significant decrease in the relative
abundance of the _Ruminococcaceae_ family. Finally, PD patients were grouped according to their Hoehn–Yahr (HY) stage. The first group contained all patients with a HY stage of 1 to 2.5, and
the second all patients with a HY stage of 3 to 4. PD patients of the first group show a significantly increased relative abundance of _Peptoniphilus_ and _Faecalibacterium_ when compared
to the control group. PD patients of the second group only showed a significant increase in the relative abundance of _Peptoniphilus_. When comparing the two groups of PD patients to each
other, no significant difference were observed. FUNCTIONAL DIVERSITY MEASURES In contrast to the alpha diversity indices calculated for the relative abundances of bacterial genera, alpha
diversity measures of predicted pathway abundances using PICRUSt revealed a significant increase in alpha diversity in PD patients compared to the control regarding observed (_p_Observed =
0.0435) and estimated (_p_Chao1 = 0.0496) metrics. Shannon and Simpson metrics did not show significant differences between PD and the control group. PD patients treated with entacapone also
showed a significantly higher diversity of predicted pathways according to observed (_p_Observed = 0.0435) and estimated (_p_Chao1 = 0.0496) metrics when compared to the control group. When
compared to patients that were not treated with entacapone, the diversity of predicted pathways was significantly higher in patients that were treated with entacapone according to observed
(_p_Observed = 0.0351) and Simpson (_p_Simpson = 0.0315) metrics. PD patients treated with L-dopa showed a borderline significance (_p_Observed = 0.05) for the abundance of predicted
pathways when compared to the control group. PD patients with a Hoehn–Yahr stage of three to four showed a significant increased alpha diversity (_p_Observed = 0.0344) for the abundance of
predicted pathways when compared to the control group. Bray–Curtis analyses, calculated for beta diversity of the predicted pathways, revealed a significant difference between the PD
patients treated with entacapone when compared to the controls and patients that were not treated with entacapone. Calculated _p_-values for the alpha and beta diversity of predicted
pathways are detailed in the Supplementary Table 2. Pathways differing significantly between PD and controls and under L-dopa or entacapone treatment are displayed in the Supplementary
Tables 3–5. DISCUSSION Besides classical motor symptoms, PD patients frequently show gastrointestinal dysfunctions including impaired gastric emptying, constipation, and defecatory
dysfunction.36 In addition, it is discussed that constipation in PD might partly be dependent on changes of the intestinal microbiota composition and their metabolic products.37 The gut
microbiota is influenced by diet, medication, the immune system, intestinal transit time, and other factors. Clearly, a basic understanding of compositional changes is needed to better
understand, how the gut microbiota might influence onset and progression of PD, or is in turn influenced by the disease itself. Another point of interest is to understand how medication and
severity of the illness impact the gut microbiota. Only a few publications addressed such changes of the intestinal microbiota in PD patients, applying different methods such as qPCR,17
pyrosequencing,14,30 and Illumina MiSeq sequencing of different regions of the 16S rRNA gene.22,32 Our data indicate a significantly decreased richness of the microbiota (observed and chao1)
(Fig. 1a). This is in contrast with former studies, where no significant differences in microbial diversity measures between PD and controls were reported.30,32 However, in agreement with
Hopfner and collegaues32 but in contrast to Scheperjans and colleagues,30 the beta diversity measures did not show significant difference between PD and control samples for the unweighted
and weighted unifrac metric (Fig. 1b+c). This hints to a very similar composition of the bacterial microbiota of PD patients and the healthy control group. Therefore, our expectation was to
find significant differences in relative abundance only for a few bacterial taxa. Calprotecin is a fecal marker for inflammation and has been shown to be elevated in PD patients compared to
control persons.16 In our study, the genera _Peptoniphilus_ and _Finegoldia_ showed a relative increase in abundance within the group of calprotectin positive PD patients. Both genera were
reported as opportunistic pathogens and being affiliated with polymicrobial infections and inflammation.38,39,40 In contrast, the genera _Faecalibacterium_ and _Fusicatenibacter_ showed a
relative decrease in abundance in the group of calprotectin positive PD patients. _Faecalibacterium_ has been reported as anti-inflammatory and health promoting, while the administration of
_Fusicatenibacter_ was reported to improve murine colitis. Both genera were reported to be decreased in inflammatory bowel disease and ulcerative colitis.41,42,43,44,45 A link between
intestinal inflammation as environmental factor and PD was previously reported.16,46 To the best of our knowledge, an association between calprotectin and the gut microbiota in PD has not
been reported yet. Since none of the subjects reported a history of acute or chronic gastrointestinal disorders, the increase of the inflammation marker calprotectin might be an indicator of
changes within the intestinal microbiota in PD that is associated with an asymptomatic, low-grade inflammation. L-dopa represents the most potent dopamine replacement agent to treat PD.47
However, gut microorganisms are able to degrade L-dopa even in the presence of decarboxylase inhibitors, thereby reducing its effectiveness.20,48,49 We found _Peptoniphilus_ and _Finegoldia_
to be relatively increased in PD patients treated with L-dopa, but not in PD patients that were treated with other dopaminergic drugs. Both genera are capable of peptone and amino acid
fermentation50,51 and might therefore play a role in the degradation of L-dopa. Previous studies found a borderline significant influence of L-dopa on the total gut microbiome or discussed
the treatment with L-dopa as a possible influencing factor.14,22,32 Furthermore, a recent study showed the important role of microbial metabolism in drug availability and degradation in the
gut.20 However, as far as we know, no study specifically addressed changes in microbial community composition in PD related to L-dopa medication in detail. Since several bacteria are capable
of metabolizing this drug, further investigations might directly target bacteria involved in this degradation process. Entacapone is the most frequently used COMT inhibitor and has been
shown to enhance the potency of L-dopa.52 When compared to the control group, patients treated with this drug showed the highest number of families and genera differing significantly in
relative abundance. Only _Peptoniphilus_ showed a difference in relative abundance also for the group of PD patients that received no entacapone treatment, when compared to the control. For
COMT inhibitors gastrointestinal side effect such as diarrhea have been reported, which may lead to changes in gut microbiota composition.52,53 Several studies reported a possible link of
COMT inhibitors to difference of abundance of some taxa.17,22,30 Especially an influence of entacapone on the abundance of _Faecalibacterium, Bifidobacterium, Lachnospiraceae, Blautia_, and
_Enterobacteriaceae_ were previously reported.17,22,30 Hill-Burns and colleagues reported the associations with PD and PD medication at OTU, genus, and family level to be robust.22 We were
able to confirm a previously reported influence of entacapone on the relative abundances of _Faecalibacterium_ and _Bifidobacterium_.17,22 In addition, we could show that _Anaerococcus_, the
_Eubacterium brachy_ group, _Sellimonas_, and _Enterococcus_ increased significantly in relative abundance only in PD patients treated with entacapone. _Faecalibacterium_ showed a relative
reduction in abundance in the PD samples compared to the controls, which corroborates several previous studies.14,17,30 Notably, in the study by Unger and colleagues,17 the identical set of
samples was investigated, albeit using qPCR. For _Faecalibacterium_, health promoting and anti-inflammatory effects have been reported.43,44,45,54 The decreased relative abundance of this
genus in conjunction with entacapone treatment, as seen in our study, is compatible with previously published findings.14,17 The potential anti-inflammatory effect of _Faecalibacterium_
matches our observation of a significantly decreased relative abundance in PD patients who showed elevated levels of calprotectin, a fecal marker for gut inflammation, but not in those
patients who showed normal values. _Faecalibacterium_ was also found to be reduced in relative abundance in PD patients who were on L-dopa treatment, but not in patients who were on other
dopaminergic treatments. Recent studies suggested L-dopa to induce inflammatory responses.55 Hence, the influence of L-dopa on anti-inflammatory bacterial genera like _Faecalibacterium_
should be examined in more detail. Given that _Faecalibacterium_ is one of the most important butyrate producers, with butyrate being the main energy source of the intestinal epithelium, a
decrease of this genus might lead to lower butyrate levels17 and thus to an impairment of the gastrointestinal mucus layer,56 rendering the enteric nervous system more susceptible to
intraluminal pathogens and inflammation. _Peptoniphilus_ is affiliated with the _Clostridiales_ family XI and was significantly increased in relative abundance in PD patients. This genus
belongs to the normal gut microbiota of humans, as well as the skin, mouth, and upper respiratory tract. Nevertheless, it has been observed in the context of polymicrobial infections,
inflammation of the upper respiratory tract, septic arthritis, and diabetes mellitus.39,40,57 The increase in relative abundance of _Peptoniphilus_ in PD patients seems to be independent of
an entacapone treatment and the inflammation marker calprotectin. However, a significant increase in abundance was observed for patients receiving L-dopa treatment, when compared to the
control group, but not for the patients with other dopaminergic treatments. So far, changes in relative abundance of this genus have not been reported before in PD. _Finegoldia_ is also
affiliated with the _Clostridiales_ family XI. Here, its abundance was found to be relatively increased in PD patients treated with L-dopa, but also in those with elevated calprotectin
levels. However, in accordance with previous studies, _Finegoldia_ was not significantly different in abundance when the control group was just compared to all PD patients.14,17,22,30,32 The
genus contains a single described species, _F. magna_, an opportunistic pathogen that can cause infections in immunocompromised hosts.38 Members of this genus were also shown to be capable
of degrading defensive proteins provided by the host.58 Many members of the _Clostridiales_ family XI are capable of peptone and amino acid fermentation, including _Peptoniphilus_ and
_Finegoldia_.50,51 It may be speculated that such organisms benefit from an altered protein expression in the colon tissue. For instance, alpha-synuclein has been shown to be pathologically
overexpressed in the colon tissue of mice with PD.59 In order to valuate this hypothesis, further studies should address the metabolic activity of these genera with respect to distinct
proteins and L-dopa, as well as their interactions with the colonic wall and ENS. Another member of the _Clostridiales_ which was decreased relatively in PD, is _Fusicatenibacter_. This
genus has been shown to be decreased in organ-specific autoimmune diseases and inflammatory diseases like ulcerative colitis.42,60 _Fusicatenibacter_ was correlated to fecal secondary bile
acids and its numbers decreased under high cholesterol levels.61 For secondary bile acids, there is evidence supporting a neuroprotective role in a diverse spectrum of age-related
neurodegenerative disorders, including PD.62 The family _Bifidobacteriaceae_ and the genus _Bifidobacterium_ were reported as significantly increased in abundance in PD.17,22 In our study,
the family _Bifidobacteriaceae_ did not show significant differences in the direct comparison of all PD patients to controls. However, its relative abundance was significantly higher in the
male PD group and the PD patients treated with entacapone. Bifidobacteria are generally regarded as health promoting, as they have stimulating effects on the immune system and confer
resistance to colonization by pathogens, which is the reason why they are widely used for probiotic treatments.63 In contrast to Unger and colleagues17 and Hopfner and colleagues,32 the
family _Enterococcaceae_ was not found to be different in abundance for the overall comparison of control group and PD. However, this family showed a significant increase in relative
abundance in PD patients treated with L-dopa or entacapone. The affiliated genus _Enterococcu_s (especially _Enterococcus faecalis_) was reported to be able to induce irritable bowel
syndrome and possesses a variety of immune evasive und protein degradation functionalities.64,65,66 Recently, _Enterococcus faecalis_ was also described to convert L-dopa to dopamine in the
gut and thus may contribute to its in vivo degradation.49 In conclusion, we were able to show that several bacterial genera differed in relative abundance between PD and control samples, in
particular under the influence of drug treatments and partly in co-occurrence with the fecal inflammation marker calprotectin. Within the PD group, we observed a decrease of bacterial taxa
presumed as being health-promoting, anti-inflammatory, neuroprotective or having other beneficial effects on the epithelial barrier, such as _Faecalibacterium_ and _Fusicatenibacter_. Both
genera were decreased in PD patients with elevated calprotectin levels. In addition, we observed an increase in shares of the _Clostridiales_ family XI and their affiliated members
_Peptoniphilus_ and _Finegoldia_, which have been suspected as being opportunistic pathogens in immune compromised hosts. Furthermore, our study confirms the previously reported possible
link of COMT inhibitors, like entacapone, with differences in abundance of various microbial taxa. Finally, we provide significant evidence for an influence of L-dopa medication on the
relative abundance of several bacterial genera. Clearly, the causative links between PD, PD medication and the composition and metabolic capacity of the gut microbiota still need to be
unraveled in more detail. Gut inflammation, gut motility, and microbial metabolism of PD drugs appear as potential starting points for further investigations, which should be focused more
strongly on microbial functionalities than abundances of microbial taxa. Interestingly, prediction of pathways with PICRUSt suggested an increased diversity of biochemical pathways in our PD
patients. Especially patients treated with entacapone showed this trend. Such trends appear ideally suited to be validated with, appropriate meta-technologies.67,68 Finally, we would like
to point out that, besides technical issue (sample size and age, sequencing technology etc.), differences between the findings of our and other studies might also be based on the distinct
geographic and cultural background of our study cohort, since the human gut microbiota is regionally different.69 Thus, studies worldwide are needed in order to get a more complete picture
of potential links between gut microbiota and PD. METHODS PATIENTS AND CONTROL GROUP The cohort under investigation comprised 34 PD patients (10 female, 24 male) and 25 healthy controls (14
female, 11 male), who have been investigated previously.17 Both groups were age matched. At the time of sampling, the mean age of PD patients was 67.9 (sd = 8.6) years and 63.9 (sd = 5.8)
years for the control group, respectively. Special dietary habits or restrictions were not reported. All subjects followed an omnivorous diet. Intakes of antibiotics, probiotics, or
prebiotics over the three months prior to the fecal sampling, as well as a history of acute or chronic gastrointestinal disorders were not reported. PD diagnosis was performed according to
the UK PD Society Brain Bank Clinical Diagnostic Criteria70 by a movement disorder specialist. All PD patients were on dopaminergic drugs (see Supplementary Table 1 for details). Mean
duration of the disease was 82 months and the median of the Hoehn and Yahr stage was 2.5.71 The control group did not report any pre-existing medical conditions or any chronic or
intermittent use of medication. The study was approved by the ethics committee of the medical association of Saarland and is recorded therein with the identification number 111/12. All
enrolled subjects provided written informed consent for their participation. FECAL SAMPLE COLLECTION AND DNA ISOLATION Fecal sampling was performed in 2015. In order to collect samples at
home, subjects were provided with sterile containers (MED AUXIL fecal collector set, Süsse, Gudensberg, Germany) and introduced to the collection procedure. Samples were sent to the
Institute of Microecology in Herborn, Germany, frozen immediately and then stored at −20 °C until further analysis. Fecal calprotectin concentrations were measured by an enzyme-linked
immunosorbent assay as reported previously.16 DNA isolation was performed in 2017, using the FastDNA SPIN kit for feces (MP Biomedicals, Heidelberg, Germany), using 150 mg–300 mg of well
homogenized fecal. DNA purity and concentration after extraction were measured with an Implen NanoPhotometer P-Class 360 (Implen GmbH, Munich, Germany). LIBRARY PREPARATION AND SEQUENCING
Sequencing library preparation of the V4 and V5 region of the bacterial 16S rRNA genes were performed using the 16S-specific primers 520 F (5′-AYTGGGYDTAAAGNG-3′) and 926 R
(5′-CCGTCAATTCMTTTRAGTTT-3′)72,73 to produce amplicons of ~380 bp length which is regarded sufficient for identification at genus level.74 PCR amplification was performed at least twice per
sample. The PCR mixture consisted of 0.5 µl of each primer (10 µM), 0.6 µl of dNTP-Mix (10 mM, each), 5 µl 5× KAPA Hifi Puffer including 20 mM MgCl2 (Roche, Mannheim, Germany), 0.1 µl KAPA
Hifi Polymerase (Roche), 1 µl DNA template, and was filled up to a final volume of 25 µl with nuclease free water. PCR reactions were performed in a T100 Thermal Cycler (Bio-Rad
Laboratories, Munich, Germany) using the following thermal profile: 3 min at 95 °C for initial denaturation, 25 cycles of 30 s at 95 °C for denaturation, 30 s at 55 °C for annealing, and 45
s at 72 °C for elongation, followed by a final elongation step for 5 min at 72 °C. Water-template controls and _Escherichia coli_ DNA as positive controls were included for each set of the
PCR reaction. Success of PCRs was verified by agarose gel electrophoresis using Midori Green as DNA-dye (Biozym, Olderndorf, Germany). Replicate PCRs of the same sample were pooled and
purified with Agencourt AMPure beads (Beckman Coulter, Krefeld, Germany) into 50 µl of 10 mM Tris (pH 8.5) buffer. Subsequently, a second PCR step was performed to add unique, custom made
index barcodes with sequencing adapters to the amplicon targets (detailed in the Supplementary Table 6; Integrated DNA Technologies, Leuven, Belgium) using the same reverse primer as before.
The index PCR reaction included 1 µl Ion-index-primer forward and 1 µl 926 R reverse primer with 1.2 µl of dNTP-Mix (10 mM each), 10 µl 5× KAPA Hifi Puffer including 20 mM MgCl2 (Roche),
0.2 µl KAPA Hifi Polymerase (Roche), 5 µl amplicon DNA, and was filled up to 50 µl with nuclease free water. PCR reactions were performed in a T100 Thermal Cycler (Bio-Rad Laboratories)
using the program detailed above, albeit with 8 cycles. With indices and linker sequences, libraries had a mean sequence length of 428 bp. Purification, quality, and quantity checks, as well
as emulsion PCR and following sequencing steps with the Ion PGM Hi-Q OT2 Kit (Thermo Fisher Scientific, Schwerte, Germany) on a Ion PGM sequencer (Thermo Fisher Scientific) were performed
following the protocol provided by Kaplan and colleagues and the manufacturer´s instruction.75 BIOINFORMATICS AND STATISTICS Sequence data were processed using QIIME 1.9.1.76 Quality cutoffs
were performed using the QIIME standard at Q ≥ 25. Minimum and maximum sequence lengths with the QIIME default of 200 bp and 1000 bp were used. The sequences of four independent runs were
merged in a single dataset. Chimeras were removed using vsearch.77 Operational taxonomic units (OTU) were chosen within 97% sequence identity. SILVA database release 128 was used to assign
taxonomy and align sequences.78 Following removal of chloroplast and mitochondrial OTUs, further statistical analyses were made with R version 3.4.3. The phyloseq package version 1.22.3 was
used for rarefaction to even sequence depth and exclusion of taxa present in less than 10% of all samples.79 Alpha diversity indices for Observed, Chao1, Shannon, and Simpson metrics, as
well as beta diversity indices for weighted and unweighted unifrac were also calculated with the phyloseq package. _P_-values were calculated with ANOVA for alpha diversity and ADONIS for
beta diversity with the package vegan, version 2.4–6.80 The package coin version 1.2–2 was used to compute differences in OTU counts between PD and controls using a two-sided
Wilcoxon–Mann–Whitney test for unpaired and non-normally distributed samples in a 10,000 fold Monte-Carlo simulation.81 Statistical analyses resulting in _p_-values were corrected using a
false discovery rate (FDR) correction for multiple testing.82 In order to compare the diversity of metabolic pathways between PD and control samples, a PicRust283
(https://github.com/picrust/picrust2/) analysis was done using the QIIME284 plugin for PicRust2 after export of the necessary files into the QIIME2 format. For the hidden-state prediction
(HSP) the method “mp” and for the maximum NSTI the value “2” (optimal for human gut) were chosen. The results file containing the predicted pathway abundances and coverages per sample, based
on predicted EC number abundances, was used for calculation of Alpha diversity (Observed, Chao, Shannon and Simpson metrics) and Beta diversity indices and according statistics by the
QIIME284 pipeline commands. Associations of categorical sample attributes were calculated with a _Χ_²-test and validated by Cramér’s V (cV) using the package DescTools version 0.99.27 for
R.85 Correlations of numeric sample attributes were calculated with a Spearman-_Ρ_.86 REPORTING SUMMARY Further information on research design is available in the Nature Research Reporting
Summary linked to this article. DATA AVAILABILITY Sequences generated and analyzed during this study are accessible at the European Nucleotide Archive (ENA) under the accession number
PRJEB30615. Subject data is included in the supplementary information files (Supplementary Table 1). Other datasets are available from the corresponding author on reasonable request. CODE
AVAILABILITY For this study, no custom functionalities were coded to generate or process the dataset. All used software and packages, their versions, relevant specification and parameters
are stated in the “Methods” section in the paragraph “Bioinformatics and Statistics”. Information that is more detailed is available from the corresponding author on reasonable request.
REFERENCES * Perez-Pardo, P. et al. The gut-brain axis in Parkinson’s disease: possibilities for food-based therapies. _Eur. J. Pharmacol._ 817, 86–95 (2017). Article CAS PubMed Google
Scholar * Fasano, A. et al. The role of small intestinal bacterial overgrowth in Parkinson’s disease. _Mov. Disord._ 28, 1241–1249 (2013). Article CAS PubMed Google Scholar * Jost, W.
H. Gastrointestinal dysfunction in Parkinson’s disease. _J. Neurol. Sci._ 289, 69–73 (2010). Article CAS PubMed Google Scholar * Pfeiffer, R. F. Gastrointestinal dysfunction in
Parkinson’s disease. _Parkinsonism Relat. Disord._ 17, 10–15 (2011). Article PubMed Google Scholar * Savica, R. et al. Medical records documentation of constipation preceding Parkinson
disease: a case-control study. _Neurology_ 73, 1752–1758 (2009). Article CAS PubMed PubMed Central Google Scholar * Chen, H. et al. Meta-analyses on prevalence of selected Parkinson’s
nonmotor symptoms before and after diagnosis. _Transl. Neurodegener._ 4, 1 (2015). Article PubMed PubMed Central Google Scholar * Abbott, R. D. et al. Frequency of bowel movements and
the future risk of Parkinson’s disease. _Neurology_ 57, 456–462 (2001). Article CAS PubMed Google Scholar * Gao, X., Chen, H., Schwarzschild, M. A. & Ascherio, A. A prospective study
of bowel movement frequency and risk of Parkinson’s disease. _Am. J. Epidemiol._ 174, 546–551 (2011). Article PubMed PubMed Central Google Scholar * Hawkes, C. H., Del Tredici, K. &
Braak, H. Parkinson’s disease: a dual-hit hypothesis. _Neuropathol. Appl. Neurobiol._ 33, 599–614 (2007). Article CAS PubMed PubMed Central Google Scholar * Cryan, J. F. & Dinan,
T. G. Mind-altering microorganisms: the impact of the gut microbiota on brain and behaviour. _Nat. Rev. Neurosci._ 13, 701–712 (2012). Article CAS PubMed Google Scholar * Braak, H. &
Del Tredici, K. Neuropathological staging of brain pathology in Sporadic Parkinson’s disease: separating the wheat from the Chaff. _J. Parkinsons Dis._ 7, S71–S85 (2017). Article PubMed
PubMed Central Google Scholar * Pan-Montojo, F. et al. Progression of Parkinson’s disease pathology is reproduced by intragastric administration of rotenone in mice. _PLoS One_ 5, e8762
(2010). Article PubMed PubMed Central CAS Google Scholar * Pan-Montojo, F. et al. Environmental toxins trigger PD-like progression via increased alpha-synuclein release from enteric
neurons in mice. _Sci. Rep._ 2, 898 (2012). Article PubMed PubMed Central CAS Google Scholar * Keshavarzian, A. et al. Colonic bacterial composition in Parkinson’s disease. _Mov.
Disord._ 30, 1351–1360 (2015). Article CAS PubMed Google Scholar * Forsyth, C. B. et al. Increased intestinal permeability correlates with sigmoid mucosa alpha-synuclein staining and
endotoxin exposure markers in early Parkinson’s disease. _PLoS ONE_ 6, e28032 (2011). Article CAS PubMed PubMed Central Google Scholar * Schwiertz, A. et al. Fecal markers of intestinal
inflammation and intestinal permeability are elevated in Parkinson’s disease. _Parkinsonism Relat. Disord_. https://doi.org/10.1016/j.parkreldis.2018.02.022 (2018). Article Google Scholar
* Unger, M. M. et al. Short chain fatty acids and gut microbiota differ between patients with Parkinson’s disease and age-matched controls. _Parkinsonism Relat. Disord._ 32, 66–72 (2016).
Article PubMed Google Scholar * Ge, X. et al. Potential role of fecal microbiota from patients with slow transit constipation in the regulation of gastrointestinal motility. _Sci. Rep._
7, 441 (2017). Article PubMed PubMed Central CAS Google Scholar * Zhu, L. et al. Structural changes in the gut microbiome of constipated patients. _Physiological genomics_ 46, 679–686,
https://doi.org/10.1152/physiolgenomics.00082.2014 (2014). Article CAS PubMed Google Scholar * van Kessel, S. P. et al. Gut bacterial tyrosine decarboxylases restrict levels of levodopa
in the treatment of Parkinson’s disease. _Nat. Commun._ 10, 310 (2019). Article PubMed PubMed Central CAS Google Scholar * van de Steeg, E. et al. An ex vivo fermentation screening
platform to study drug metabolism by human gut microbiota. _Drug Metab. Disposition_ 46, 1596–1607 (2018). Article CAS Google Scholar * Hill-Burns, E. M. et al. Parkinson’s disease and
Parkinson’s disease medications have distinct signatures of the gut microbiome. _Mov. Disord._ 32, 739–749 (2017). Article CAS PubMed PubMed Central Google Scholar * Dorszewska, J.,
Prendecki, M., Lianeri, M. & Kozubski, W. Molecular effects of l-dopa therapy in Parkinson’s disease. _Curr. Genomics_ 15, 11–17 (2014). Article CAS PubMed PubMed Central Google
Scholar * Koller, W. C. & Rueda, M. G. Mechanism of action of dopaminergic agents in Parkinson’s disease. _Neurology_ 50, S11–S114 (1998). discussion S44-8. Article CAS PubMed Google
Scholar * Männistö, P. T. & Kaakkola, S. New selective COMT inhibitors: useful adjuncts for Parkinson’s disease? _Trends Pharmacol. Sci._ 10, 54–56 (1989). Article PubMed Google
Scholar * Sharpless, N. S. & McCann, D. S. Dopa and 3-O-methyldopa in cerebrospinal fluid of Parkinsonism patients during treatment with oral L-dopa. _Clin. Chim. Acta_ 31, 155–169
(1971). Article CAS PubMed Google Scholar * Guldberg, H. C. & Marsden, C. A. Catechol-O-methyl transferase: pharmacological aspects and physiological role. _Pharmacol. Rev._ 27,
135–206 (1975). CAS PubMed Google Scholar * Bonifácio, M. J., Palma, P. N., Almeida, L. & Soares-da-Silva, P. Catechol-O-methyltransferase and its inhibitors in Parkinson’s disease.
_CNS Drug Rev._ 13, 352–379 (2007). Article PubMed PubMed Central Google Scholar * Nissinen, E. et al. Inhibition of catechol-O-methyltransferase activity by two novel disubstituted
catechols in the rat. _Eur. J. Pharmacol._ 153, 263–269 (1988). Article CAS PubMed Google Scholar * Scheperjans, F. et al. Gut microbiota are related to Parkinson’s disease and clinical
phenotype. _Mov. Disord._ 30, 350–358 (2015). Article PubMed Google Scholar * Li, W. et al. Structural changes of gut microbiota in Parkinson’s disease and its correlation with clinical
features. _Sci. China Life Sci._ 60, 1223–1233 (2017). Article PubMed Google Scholar * Hopfner, F. et al. Gut microbiota in Parkinson disease in a northern German cohort. _Brain Res._
1667, 41–45 (2017). Article CAS PubMed Google Scholar * Bedarf, J. R. et al. Functional implications of microbial and viral gut metagenome changes in early stage L-DOPA-naïve Parkinson’s
disease patients. _Genome Med._ 9, 39 (2017). Article CAS PubMed PubMed Central Google Scholar * Tetz, G., Brown, S. M., Hao, Y. & Tetz, V. Parkinson’s disease and bacteriophages
as its overlooked contributors. _Sci. Rep._ 8, 10812 (2018). Article PubMed PubMed Central CAS Google Scholar * Smithson, M. _Confidence Intervals_. (Sage Publ, Thousand Oaks, 2006).
Google Scholar * Cersosimo, M. G. & Benarroch, E. E. Pathological correlates of gastrointestinal dysfunction in Parkinson’s disease. _Neurobiol. Dis._ 46, 559–564 (2012). Article
PubMed Google Scholar * Zhao, Y. & Yu, Y.-B. Intestinal microbiota and chronic constipation. _Springerplus_ 5, 1130 (2016). Article PubMed PubMed Central Google Scholar * Higaki,
S. & Morohashi, M. Characteristics of anaerobes from skin specimens. _Drugs Exp. Clin. Res._ 29, 153–155 (2003). CAS PubMed Google Scholar * Jung, M. Y. et al. Peptoniphilus
rhinitidis sp. nov., isolated from specimens of chronic rhinosinusitis. _Anaerobe_ 30, 30–34 (2014). Article PubMed Google Scholar * Cobo, F., Rodríguez-Granger, J., Sampedro, A. &
Navarro-Marí, J. M. Peritoneal infection due to Peptoniphilus harei in a patient with intestinal occlusion. _Anaerobe_ 44, 126–127 (2017). Article PubMed Google Scholar * Rapozo, D. C.
M., Bernardazzi, C. & Souza, H. S. Pde Diet and microbiota in inflammatory bowel disease: The gut in disharmony. _World J. Gastroenterol._ 23, 2124–2140 (2017). Article CAS PubMed
PubMed Central Google Scholar * Takeshita, K. et al. A single species of Clostridium Subcluster XIVa decreased in ulcerative colitis patients. _Inflamm. Bowel Dis._ 22, 2802–2810 (2016).
Article PubMed Google Scholar * Laval, L. et al. Lactobacillus rhamnosus CNCM I-3690 and the commensal bacterium Faecalibacterium prausnitzii A2-165 exhibit similar protective effects to
induced barrier hyper-permeability in mice. _Gut Microbes_ 6, 1–9 (2015). Article CAS PubMed PubMed Central Google Scholar * Martín, R. et al. Faecalibacterium prausnitzii prevents
physiological damages in a chronic low-grade inflammation murine model. _BMC Microbiol._ 15, 67 (2015). Article PubMed PubMed Central Google Scholar * Maier, E., Anderson, R. C. &
Roy, N. C. Live Faecalibacterium prausnitzii Does Not Enhance Epithelial Barrier Integrity in an Apical Anaerobic Co-Culture Model of the Large Intestine. _Nutrients_ 9;
https://doi.org/10.3390/nu9121349 (2017). Article PubMed Central CAS Google Scholar * Becker, A., Faßbender, K., Oertel, W. H. & Unger, M. M. A punch in the gut-Intestinal
inflammation links environmental factors to neurodegeneration in Parkinson’s disease. _Parkinsonism Relat. Disord_. https://doi.org/10.1016/j.parkreldis.2018.09.032 (2018). Article Google
Scholar * Salat, D. & Tolosa, E. Levodopa in the treatment of Parkinson’s disease: current status and new developments. _J. Parkinsons Dis._ 3, 255–269 (2013). CAS PubMed Google
Scholar * Goldin, B. R., Peppercorn, M. A. & Goldman, P. Contributions of host and intestinal microflora in the metabolism of L-dopa by the rat. _J. Pharmacol. Exp. therapeutics_ 186,
160–166 (1973). CAS Google Scholar * Maini Rekdal, V., Bess, E. N., Bisanz, J. E., Turnbaugh, P. J. & Balskus, E. P. Discovery and inhibition of an interspecies gut bacterial pathway
for Levodopa metabolism. _Science_ 364; https://doi.org/10.1126/science.aau6323 (2019). Article PubMed Google Scholar * Ezaki, T. et al. Proposal of the genera Anaerococcus gen. nov.,
Peptoniphilus gen. nov. and Gallicola gen. nov. for members of the genus Peptostreptococcus. _Int. J. Syst. Evol. Microbiol._ 51, 1521–1528 (2001). Article CAS PubMed Google Scholar *
Murphy, E. C. & Frick, I.-M. Gram-positive anaerobic cocci–commensals and opportunistic pathogens. _FEMS Microbiol. Rev._ 37, 520–553 (2013). Article CAS PubMed Google Scholar *
Gordin, A., Kaakkola, S. & Teräväinen, H. Clinical advantages of COMT inhibition with entacapone-a review. _J Neural Transm_ 111, (1343–1363 (2004). Google Scholar * Kaakkola, S.
Clinical pharmacology, therapeutic use and potential of COMT inhibitors in Parkinson’s disease. _Drugs_ 59, 1233–1250 (2000). Article CAS PubMed Google Scholar * Chen, J. et al. Multiple
sclerosis patients have a distinct gut microbiota compared to healthy controls. _Sci. Rep._ 6, 28484 (2016). Article CAS PubMed PubMed Central Google Scholar * Pisanu, A. et al.
Neuroinflammation in L-DOPA-induced dyskinesia: beyond the immune function. _J Neural Transm_ 125, (1287–1297 (2018). Google Scholar * Wrzosek, L. et al. Bacteroides thetaiotaomicron and
Faecalibacterium prausnitzii influence the production of mucus glycans and the development of goblet cells in the colonic epithelium of a gnotobiotic model rodent. _BMC Biol._ 11, 61 (2013).
Article PubMed PubMed Central CAS Google Scholar * Verma, R., Morrad, S. & Wirtz, J. J. Peptoniphilus asaccharolyticus-associated septic arthritis and osteomyelitis in a woman with
osteoarthritis and diabetes mellitus. _BMJ Case Reports_ 2017, https://doi.org/10.1136/bcr-2017-219969 (2017). * Frick, I.-M. et al. Constitutive and inflammation-dependent antimicrobial
peptides produced by epithelium are differentially processed and inactivated by the commensal Finegoldia magna and the pathogen Streptococcus pyogenes. _J. Immunol._ 187, 4300–4309 (2011).
Article CAS PubMed Google Scholar * Kelly, L. P. et al. Progression of intestinal permeability changes and alpha-synuclein expression in a mouse model of Parkinson’s disease. _Mov.
Disord._ 29, 999–1009 (2014). Article CAS PubMed Google Scholar * Zhao, F. et al. Alterations of the gut microbiota in Hashimoto’s thyroiditis patients. _Thyroid_ 28, 175–186 (2018).
Article CAS PubMed Google Scholar * Prieto, I. et al. Influence of a diet enriched with virgin olive oil or butter on mouse gut microbiota and its correlation to physiological and
biochemical parameters related to metabolic syndrome. _PLoS ONE_ 13, e0190368 (2018). Article PubMed PubMed Central CAS Google Scholar * Ackerman, H. D. & Gerhard, G. S. Bile acids
in neurodegenerative disorders. _Front. Aging Neurosci._ 8, 263 (2016). Article PubMed PubMed Central CAS Google Scholar * Picard, C. et al. Review article: bifidobacteria as probiotic
agents–physiological effects and clinical benefits. _Alimentary Pharmacol. Therapeutics_ 22, 495–512 (2005). Article CAS Google Scholar * Balish, E. & Warner, T. Enterococcus faecalis
induces inflammatory bowel disease in interleukin-10 knockout mice. _Am. J. Pathol._ 160, 2253–2257 (2002). Article CAS PubMed PubMed Central Google Scholar * Golińska, E. et al.
Virulence factors of Enterococcus strains isolated from patients with inflammatory bowel disease. _World J. Gastroenterol._ 19, 3562–3572 (2013). Article PubMed PubMed Central CAS Google
Scholar * Giridhara Upadhyaya, P. M., Ravikumar, K. L. & Umapathy, B. L. Review of virulence factors of enterococcus: an emerging nosocomial pathogen. _Indian J. Med. Microbiol._ 27,
301–305 (2009). Article CAS PubMed Google Scholar * Petriz, B. A. & Franco, O. L. Metaproteomics as a complementary approach to gut microbiota in health and disease. _Front. Chem._
5, 4 (2017). Article PubMed PubMed Central CAS Google Scholar * Mondot, S. & Lepage, P. The human gut microbiome and its dysfunctions through the meta-omics prism. _Ann. NY Acad.
Sci._ 1372, 9–19 (2016). Article PubMed Google Scholar * He, Y. et al. Regional variation limits applications of healthy gut microbiome reference ranges and disease models. _Nat. Med._
24, 1532–1535 (2018). Article CAS PubMed Google Scholar * Massano, J. & Bhatia, K. P. Clinical approach to Parkinson’s disease: features, diagnosis, and principles of management.
_Cold Spring Harb. Perspect. Med._ 2, a008870 (2012). Article PubMed PubMed Central Google Scholar * Hoehn, M. M. & Yahr, M. D. Parkinsonism: onset, progression and mortality.
_Neurology_ 17, 427–442 (1967). Article CAS PubMed Google Scholar * Claesson, M. J. et al. Comparative analysis of pyrosequencing and a phylogenetic microarray for exploring microbial
community structures in the human distal intestine. _PLoS ONE_ 4, e6669 (2009). Article PubMed PubMed Central CAS Google Scholar * Engelbrektson, A. et al. Experimental factors
affecting PCR-based estimates of microbial species richness and evenness. _ISME J._ 4, 642–647 (2010). Article CAS PubMed Google Scholar * Ding, L.-J., Su, J.-Q., Xu, H.-J., Jia, Z.-J.
& Zhu, Y.-G. Long-term nitrogen fertilization of paddy soil shifts iron-reducing microbial community revealed by RNA-(13)C-acetate probing coupled with pyrosequencing. _ISME J._ 9,
721–734 (2015). Article CAS PubMed Google Scholar * Kaplan, H., Ratering, S., Felix-Henningsen, P. & Schnell, S. Stability of in situ immobilization of trace metals with different
amendments revealed by microbial 13C-labelled wheat root decomposition and efflux-mediated metal resistance of soil bacteria. _Sci. Total Environ._ 659, 1082–1089 (2019). Article CAS
PubMed Google Scholar * Caporaso, J. G. et al. QIIME allows analysis of high-throughput community sequencing data. _Nat. Methods_ 7, 335–336 (2010). Article CAS PubMed PubMed Central
Google Scholar * Rognes, T., Flouri, T., Nichols, B., Quince, C. & Mahé, F. VSEARCH: a versatile open source tool for metagenomics. _PeerJ_ 4, e2584 (2016). Article PubMed PubMed
Central Google Scholar * Pruesse, E. et al. SILVA: a comprehensive online resource for quality checked and aligned ribosomal RNA sequence data compatible with ARB. _Nucleic Acids Res._ 35,
7188–7196 (2007). Article CAS PubMed PubMed Central Google Scholar * McMurdie, P. J. & Holmes, S. phyloseq: an R package for reproducible interactive analysis and graphics of
microbiome census data. _PLoS ONE_ 8, e61217 (2013). Article CAS PubMed PubMed Central Google Scholar * Oksanen, J. et al. _vegan: Community Ecology Package. R package version 2.4-6_.
https://CRAN.R-project.org/package=vegan (2018). * Hothorn, T., Hornik, K., van de Wiel, Mark, A. & Zeileis, A. A lego system for conditional inference. _Am. Stat._ 60, 257–263 (2006).
Article Google Scholar * Benjamini, Y. & Hochberg, Y. Controlling the false discovery rate-a practical and powerful approach to multiple testing. _J. R. Stat. Soc. Ser. A Method_. 57,
289–300 (1995). * Langille, M. G. I. et al. Predictive functional profiling of microbial communities using 16S rRNA marker gene sequences. _Nat. Biotechnol._ 31, 814–821 (2013). Article CAS
PubMed PubMed Central Google Scholar * Bolyen, E. et al. _QIIME 2: reproducible, interactive, scalable, and extensible microbiome data science._ (2018). * Signorell, Andri et mult. et
al. _DescTools: tools for descriptive statistics_. R package version 0.99.27. https://cran.r-project.org/package=DescTools (2019). * Hollander, M., Wolfe, D. A. & Chicken, E.
_Nonparametric Statistical Methods_ (John Wiley & Sons Inc, Hoboken, New Jersey 2014). Download references ACKNOWLEDGEMENTS The authors wish to thank Massimiliano Cardinale and Bellinda
Schneider (Giessen, Germany), as well as Manuela Jakobi and Pamela Passauer (Herborn, Germany) for their expertize, and technical assistance with the Ion Torrent sequencer. Funding was
provided by the German Federal Ministry of Education and Research (BMBF, Project immENS, grant number 03FH036PB5). AUTHOR INFORMATION AUTHORS AND AFFILIATIONS * Faculty of Medical and Life
Sciences, Institute of Precision Medicine, Microbiology and Hygiene Group, Furtwangen University, Villingen-Schwenningen, Germany Severin Weis & Markus Egert * MVZ Institute of
Microecology, Herborn, Germany Andreas Schwiertz * Department of Neurology, Saarland University, Homburg, Germany Marcus M. Unger, Anouck Becker & Klaus Faßbender * Institute of Applied
Microbiology, Justus-Liebig-University, Giessen, Germany Stefan Ratering & Sylvia Schnell * Faculty of Medical and Life Sciences, Institute of Precision Medicine, Group for Statistics in
Biology and Medicine, Furtwangen University, Villingen-Schwenningen, Germany Matthias Kohl * Department of Biotechnology, ENS Working Group, University of Applied Sciences Kaiserslautern,
Zweibrücken, Germany Karl-Herbert Schäfer Authors * Severin Weis View author publications You can also search for this author inPubMed Google Scholar * Andreas Schwiertz View author
publications You can also search for this author inPubMed Google Scholar * Marcus M. Unger View author publications You can also search for this author inPubMed Google Scholar * Anouck
Becker View author publications You can also search for this author inPubMed Google Scholar * Klaus Faßbender View author publications You can also search for this author inPubMed Google
Scholar * Stefan Ratering View author publications You can also search for this author inPubMed Google Scholar * Matthias Kohl View author publications You can also search for this author
inPubMed Google Scholar * Sylvia Schnell View author publications You can also search for this author inPubMed Google Scholar * Karl-Herbert Schäfer View author publications You can also
search for this author inPubMed Google Scholar * Markus Egert View author publications You can also search for this author inPubMed Google Scholar CONTRIBUTIONS A.S., M.U., A.B. and K.F.
conceived the clinical setup and executed the sampling, the storage, and the distribution of the samples. S.W. performed the DNA isolation, quality controls, and sequencing. S.W., S.R., and
M.K. set up and performed the bioinformatics procedures. S.W., S.R., S.S., A.S., M.U., K.S. and M.E. contributed to the paper. All authors approved the final version of this article.
CORRESPONDING AUTHOR Correspondence to Markus Egert. ETHICS DECLARATIONS COMPETING INTERESTS The authors declare that this research was conducted in the absence of commercial or financial
relationships that could be construed as potential conflicts of interest. ADDITIONAL INFORMATION PUBLISHER’S NOTE Springer Nature remains neutral with regard to jurisdictional claims in
published maps and institutional affiliations. SUPPLEMENTARY INFORMATION SUPPLEMENTARY TABLES REPORTING SUMMARY CHECKLIST RIGHTS AND PERMISSIONS OPEN ACCESS This article is licensed under a
Creative Commons Attribution 4.0 International License, which permits use, sharing, adaptation, distribution and reproduction in any medium or format, as long as you give appropriate credit
to the original author(s) and the source, provide a link to the Creative Commons license, and indicate if changes were made. The images or other third party material in this article are
included in the article’s Creative Commons license, unless indicated otherwise in a credit line to the material. If material is not included in the article’s Creative Commons license and
your intended use is not permitted by statutory regulation or exceeds the permitted use, you will need to obtain permission directly from the copyright holder. To view a copy of this
license, visit http://creativecommons.org/licenses/by/4.0/. Reprints and permissions ABOUT THIS ARTICLE CITE THIS ARTICLE Weis, S., Schwiertz, A., Unger, M.M. _et al._ Effect of Parkinson’s
disease and related medications on the composition of the fecal bacterial microbiota. _npj Parkinsons Dis._ 5, 28 (2019). https://doi.org/10.1038/s41531-019-0100-x Download citation *
Received: 22 March 2019 * Accepted: 29 October 2019 * Published: 29 November 2019 * DOI: https://doi.org/10.1038/s41531-019-0100-x SHARE THIS ARTICLE Anyone you share the following link with
will be able to read this content: Get shareable link Sorry, a shareable link is not currently available for this article. Copy to clipboard Provided by the Springer Nature SharedIt
content-sharing initiative