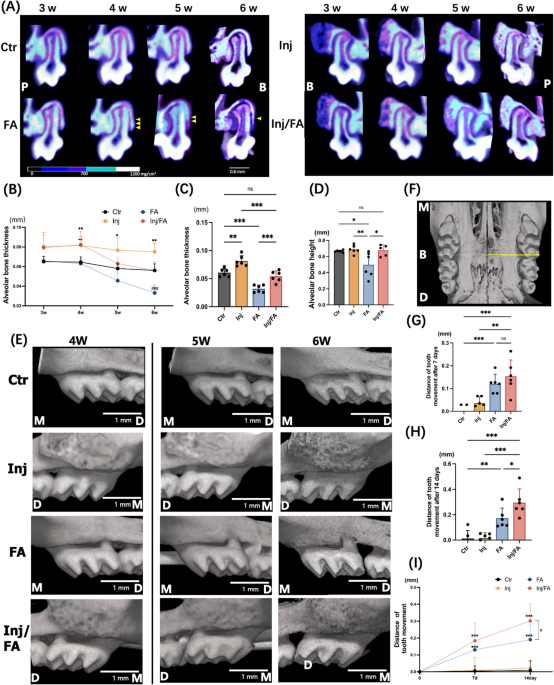
Prevention of bone dehiscence associated with orthodontic tooth movement by prophylactic injection of bone anabolic agents in mice
- Select a language for the TTS:
- UK English Female
- UK English Male
- US English Female
- US English Male
- Australian Female
- Australian Male
- Language selected: (auto detect) - EN
Play all audios:

ABSTRACT Although bone dehiscence may occur during orthodontic tooth movement into the narrow alveolar ridge, a non-invasive prevention method is yet to be fully established. We show for the
first time prevention of bone dehiscence associated with orthodontic tooth movement by prophylactic injection of bone anabolic agents in mice. In this study, we established a bone
dehiscence mouse model by applying force application and used the granular type of scaffold materials encapsulated with bone morphogenetic protein (BMP)-2 and OP3-4, the receptor activator
of NF-κB ligand (RANKL)-binding peptide, for the prophylactic injection to the alveolar bone. In vivo micro-computed tomography revealed bone dehiscence with decreased buccal alveolar bone
thickness and height after force application, whereas no bone dehiscence was observed with the prophylactic injection after force application, and alveolar bone thickness and height were
kept at similar levels as those in the control group. Bone histomorphometry analyses revealed that both bone formation and resorption parameters were significantly higher in the injection
with force application group than in the force application without the prophylactic injection group. These findings suggest that the prophylactic local delivery of bone anabolic reagents can
prevent bone dehiscence with increased bone remodelling activity. SIMILAR CONTENT BEING VIEWED BY OTHERS POLYSACHARIDE OF _AGARICUS_ _BLAZEI_ GEL MITIGATES BONE NECROSIS IN MODEL OF THE
JAWS RELATED TO BISPHOSPHONATE VIA WNT SIGNALING Article Open access 08 April 2024 BETA TRICALCIUM PHOSPHATE, EITHER ALONE OR IN COMBINATION WITH ANTIMICROBIAL PHOTODYNAMIC THERAPY OR
DOXYCYCLINE, PREVENTS MEDICATION-RELATED OSTEONECROSIS OF THE JAW Article Open access 03 October 2022 SDF-1 INVOLVEMENT IN ORTHODONTIC TOOTH MOVEMENT AFTER TOOTH EXTRACTION Article Open
access 29 February 2024 INTRODUCTION Insufficient alveolar bone volume is a common problem encountered in dental treatments, including periodontal disease treatment, prosthetic
reconstruction, tooth transplantation treatment, and orthodontic treatment1. In orthodontic treatment, the thickness of the alveolar bone defines one of the boundaries of the tooth movement.
Tooth movement in a constricted alveolar ridge, including molar buccal expansion, space closure of extraction or missing sites in an atrophic alveolar ridge (AAR), and incisor labio-lingual
movements, may cause undesirable collateral impacts such as bone dehiscence, gingival recession, root resorption and reduction in tooth movement rate2,3,4. The presence of bone dehiscence
has been proven to jeopardise the aesthetic appearance of the periodontal tissue and negatively affect the long-term stability of orthodontic treatment5,6,7,8. During dental arch expansion,
as the roots move into the thin buccal alveolar bone plate by force, there could be perforation of the cortical plate. Previous clinical studies using cone-beam computed tomography (CBCT)
reported that rapid maxillary expansion induces bone dehiscence, along with a decrease in the buccal alveolar bone thickness and height of the supporting teeth9,10. Another clinical
challenge is the space closure of edentulous regions in the AAR. AAR often manifests as a pronounced buccolingual collapse and crestal bone loss. To achieve space closure in the AAR,
reshaping of the cortical bone is required11,12. Studies using CBCT images confirmed that bone dehiscence increased after tooth movement through the AAR, mainly in the buccal plate13. Tooth
movement through the AAR also results in the loss of periodontal support14,15. Regarding the labio-lingual movements of the mandibular incisors, a decrease in alveolar bone thickness and
root perforations of the lingual cortical plates are often detected16,17. To overcome these anatomical restrictions against tooth movement, various studies have made efforts to obtain
alveolar bone augmentation and prevent bone dehiscence18,19,20,21. However, these invasive surgical procedures using bone grafting methods or osteoperforation are often associated with the
risk of harvesting healthy bone, potential infections, and postoperative pain22,23. In addition, it is also reported that clinical bone substitutes such as Bio-Oss grafts could slow down
orthodontic tooth movement24. Alternative minimally invasive approaches for alveolar bone augmentation have been actively investigated to mitigate the collateral effects of surgical
procedures. Injection of injectable growth factors using gelatin hydrogel as a carrier is considered a safe and feasible method for tissue regeneration. The injection method was shown to
reduce the operating time and minimise damage to surrounding tissue25. Bone morphogenetic protein (BMP)-2 is a strong growth factor clinically used to induce local bone formation26,27. On
the other hand, a new anabolic reagent, OP3-4 peptide, which is the receptor activator of NF-κB ligand (RANKL)-binding peptide, is known to inhibit bone resorption and enhance BMP-2-induced
local bone formation in vitro and in vivo28,29,30. We developed an injection technique that allowed the incorporation of BMP-2 and OP3-4 peptide into a granular type of gelatin hydrogel
carrier to achieve local alveolar bone augmentation in a mouse model31,32. However, the preventive effect of this injection method on bone dehiscence has not been investigated, since a mouse
model of bone dehiscence induced by tooth-movement has not been established yet. In this study, we established a novel mouse model of FA-induced alveolar bone dehiscence. Using this model
and bone histomorphometric measurements, we showed the preventive effect of the minimally invasive anabolic injection of BMP-2 and OP3-4 peptide on bone dehiscence induced by force
application and an increase of tooth movement in mice with high bone remodelling activities. RESULTS BONE DEHISCENCE APPEARED DURING FORCE APPLICATION Changes in the buccal alveolar bone
were tracked for six weeks following anabolic injection. In vivo micro-focal computed tomography (μCT) images of the maxillary first molar (M1) at distobuccal root (DBR) site showed that the
alveolar bone thickness and alveolar bone height of the Ctr group were at the same levels throughout the experiment, while the FA group showed continuous decrease in both alveolar bone
thickness and alveolar bone height after force application (Fig. 1A). To confirm the μCT observations, quantitative analyses were performed. Alveolar bone thickness of the Ctr group showed
no significant differences at weeks 5 and 6 compared to week 4. In the FA group, the alveolar bone at week 5 was 26.3% thinner than that at week 4. Furthermore, the alveolar bone thickness
at week 6 showed a 42.1% decrease compared with that at week 4 (Fig. 1B). At week 6, the FA group was 46% lower in alveolar bone thickness compared to the Ctr group (Fig. 1C). Quantitative
analyses of the alveolar bone height showed analogous results. Alveolar bone height in the FA group was significantly lower than that in the Ctr group at week 6 (Fig. 1D). The distance
between the cementoenamel junction (CEJ) and the alveolar crest was significantly higher in the FA group than in the other groups (Supplementary Fig. 1). The three-dimensional (3D) μCT
images of the M1s confirmed that no bone dehiscence was observed on buccal side of the alveolar bone before force application in all four groups at week 4. However, bone dehiscence was
observed in the buccal alveolar bone at the DBR site of M1 in the FA group after seven days of force application and was aggravated in the following week (Fig. 1E). Consistent with the data
from radiological analyses, Von Kossa staining showed that the FA group had 28% smaller calcified alveolar bone area and 27.8% thinner average alveolar bone than the Ctr group (Fig. 2A–C).
ANABOLIC INJECTION PREVENTED FORCE APPLICATION INDUCED BONE DEHISCENCE The injections with gelatin hydrogel alone were performed firstly to confirm no bone augmentation at the site of
injection, although we had already shown no osteoconductive effects of gelatin hydrogel itself29,31. As we expected, the injections of gelatin hydrogel alone could not induce apparent bone
formation (Supplementary Fig. 2). On the other hand, with the injection of gelatin hydrogel, BMP-2 and OP3-4 peptide, the Inj and Inj/FA groups showed significantly thicker alveolar bones
than the Ctr group at week 4 (Fig. 1A,E). The Injection groups continued to show a steady and thicker value in alveolar bone thickness compared to the other groups from weeks 4 to 6. In
addition, the mesial-distal distance of the regenerated bone in injection groups shown in the 3D μCT images (Fig. 1E) presented similar as the mesial-distal distance of the yellow area
obtained from the in vivo imaging system (IVIS) (Supplementary Fig. 3A), suggesting that the range of the scaffold delivery would be a guide for the size and the range of the regenerated
bone. After force application, the Inj/FA group showed a continuous decrease in alveolar bone thickness (Fig. 1B). At week 6, the Inj/FA group kept a similar level of alveolar bone thickness
as the Ctr group and was significantly thicker in alveolar bone thickness compared to the FA group (Fig. 1C). Additionally, in the first week after force application, the Inj/FA group
showed a similar rate of change in alveolar bone thickness as the FA group. In the second week of force application, the decrease in alveolar bone thickness was alleviated in the Inj/FA
group compared to the FA group (Supplementary Fig. 4). There were no significant differences in the alveolar bone height between the Ctr and Inj/FA groups. The Inj/FA group showed a similar
level of alveolar bone height as the Ctr group and a higher value of alveolar bone height than the FA group (Fig. 1D). In addition, no significant differences were observed in the distance
between the CEJ and alveolar crest between the Inj/FA and Ctr groups (Supplementary Fig. 1). The 3D μCT images did not show any dehiscence of the buccal alveolar bone in the Inj/FA group
throughout the study period (Fig. 1E). Similar to the μCT analyses, the Inj/FA group showed no significant difference in the average bone thickness at week 6, but had a significantly larger
calcified bone area compared to the Ctr group in von Kossa staining. The Inj/FA group also had a significantly larger calcified bone area and thicker bone than the FA group (Fig. 2A–C).
COMBINATION OF ANABOLIC INJECTION AND FORCE APPLICATION PROMOTED BONE TURNOVER Analysis of the 3D images revealed a continuous increase in the tooth movement distance in both the FA and
Inj/FA groups after 7 and 14 days of force application (Fig. 1G–I). When comparing the FA and Inj/FA groups, the Inj/FA group showed a significantly greater tooth movement distance than the
FA group after 14 days of force application (Fig. 1H). Further investigation was performed to clarify the bone remodelling activity in the local alveolar bone. Tartrate-resistant acid
phosphatase (TRAP)-positive multinucleated osteoclasts appeared on the compression side of the alveolar bone after force application in both the FA and Inj/FA groups (Fig. 3A,D).
Quantitative analyses of the osteoclast surface per bone surface (Oc.S/BS) and eroded surface per bone surface (ES/BS) confirmed these observations, and the FA and Inj/FA groups presented
higher values in both Oc.S/BS and ES/BS than the Ctr group (Fig. 3B,C). Furthermore, the ES/BS ratio was significantly higher in the Inj/FA group than in the FA group. Fluorescent labelling
images revealed that the mineral apposition rate (MAR), which represents osteoblast activity, was 132% higher in the Inj group and 198% higher in the Inj/FA group than in the Ctr group. The
MAR of the Inj/FA group was 54% higher than that of the FA group (Fig. 4A,B). Similarly, the bone formation rate (BFR) showed prominent bone formation activity in both the Inj and Inj/FA
groups compared to the Ctr group during the 14 days of force application (Fig. 4C). Meanwhile, no significant difference was observed in either MAR or BFR between the Inj and Inj/FA groups.
DISCUSSION In this study, we developed a mouse model of FA-induced bone dehiscence. Bone dehiscence is commonly detected after tooth movement in a narrow alveolar ridge, which jeopardises
long-term stability. In the current clinical treatment, no standard treatment methods have been developed to prevent bone dehiscence induced by tooth movement33. Therefore, it is crucial to
explore alternative approaches for preventing bone dehiscence. Additionally, assessing the alveolar ridge for bone dehiscence during dental treatment is essential. Bone dehiscence can be
defined as an increase in the distance between the CEJ and the buccal or lingual alveolar bone crest34. In this study, bone dehiscence appeared on the buccal side of M1 after force
application (Fig. 1E). The distances between the CEJ and the alveolar bone crest were significantly greater in the FA group than in the other groups (Supplementary Fig. 1). In addition, the
development of bone dehiscence is commonly related to alveolar bone height and thickness35,36. Our data showed that the alveolar bone height and thickness of the FA group were also
significantly lower (Fig. 1B–D). Our results confirmed the presence of bone dehiscence in the FA group. Thus, an FA-induced bone dehiscence mouse model was developed to elucidate the bone
response to mechanical force in a narrow alveolar ridge. A previous study showed that the bone mineral density (BMD) of the induced bone increased continuously within 8 weeks after the
anabolic injection on the palatal side32. In our study, a prophylactic injection resulted in the BMD of the induced bone increasing gradually at the buccal side during the six-week period
(Fig. 1A). Bone augmentation is achieved by injecting anabolic bone reagents into the alveolar bone, leading to thicker alveolar bone for tooth movement and expanding tooth movement
limitations. Since no osteoconductive effects of gelatin hydrogel was shown (Supplementary Fig. 2), it was also confirmed that the outcome of this study is caused by the effect of BMP-2 and
OP3-4 peptide, not by the gelatin hydrogel scaffold. It is known that the rate of tooth movement decreases when the tooth is moved into a region with reduced alveolar bone37. Thus, it is
possible that preliminary bone formation after injecting anabolic reagents in the alveolar ridge with anatomic restrictions allows tooth movement without delay. On the other hand, no bone
dehiscence was observed in the Inj/FA group until the end of force application, and both the alveolar bone thickness and alveolar bone height in the Inj/FA group remained at a level similar
to that in the Ctr group after applying force. Surprisingly, in the Inj/FA group, the rate of change in alveolar bone thickness began to decrease at week 5, whereas that in the FA group
increased continuously (Supplementary Fig. 4). These results suggest that the injection of anabolic bone reagents can protect the narrow alveolar ridge from the effects of force application.
The rate of tooth movement in a narrow alveolar ridge is inhibited compared to when the teeth are moved to a location with sufficient bone4. Given that in our study force application led to
bone dehiscence (Fig. 1E), the alveolar ridge to which the tooth moved due to force application was thought to be narrow, and the speed of tooth movement in the FA group should have been
inhibited. In contrast, a tooth with sufficient alveolar bone width moves faster than a tooth with a thin alveolar bone38. Due to the augmentation of the alveolar bone achieved by anabolic
injection in the Inj/FA group (Fig. 1A), the shortening of the tooth movement period was supposed to have taken place. In this study, our results confirmed the above-mentioned hypothesis,
revealing that the tooth movement distance was greater in the Inj/FA group within two weeks of force application than in the FA group (Fig. 1H). Shortening the duration of tooth movement
treatment has been reported to be associated with a decrease in certain side effects, such as oral hygiene-related problems, gingival recession, and root resorption39. So far, several
approaches have been utilised to shorten tooth movement treatment. Recently, a minimally invasive injection method using platelet-rich plasma (PRP) was reported to shorten the treatment
period40. A study by Alomari et al. showed that bone dehiscence and fenestrations were not prevented by orthodontic treatment with PRP injection41. However, our findings suggested that the
use of the anabolic injection could shorten the tooth movement period without causing bone dehiscence during tooth movement. We then clarified the morphological and biological changes in the
alveolar bone after applying force to the area where bone augmentation occurred. Bone histomorphometric analyses showed higher bone remodelling activity in the Inj/FA group than in the FA
group (Figs. 3 and 4). ES/BS, a bone resorption parameter indicating osteoclast activity, was significantly higher in the Inj/FA group than in the FA group. As for the bone formation
activities, dynamic bone histomorphometric analyses using fluorescent-labelled images showed a higher value of MAR and BFR in the Inj/FA group compared to the FA group. This implied that the
combination of anabolic injection and force application led to a higher level of bone formation. These results suggested that a higher bone remodelling activity was induced by anabolic
injection in the local alveolar bone. This could explain the faster tooth movement in the Inj/FA group than in the FA group. Our study investigated a minimally invasive injection method of
BMP-2 and OP3-4 peptide, and showed that the anabolic injection method could achieve alveolar ridge augmentation and expand the range of tooth movement. Additionally, the combination of
anabolic injection and force application led to further tooth movement without evident bone dehiscence. In conclusion, we developed the first FA-induced bone dehiscence mouse model and
showed that a minimally invasive anabolic injection method could prevent bone dehiscence induced by force application. Thus, this method has a high potential to facilitate orthodontic
treatment for patients with anatomic limitations and might be useful in treating periodontal disease, prosthetic treatment with dentures, dental implants, and tooth transplantation
accompanied by missing teeth. METHODS ANIMALS Twelve seven-week-old C57BL/6J male mice bred by NIPPON CLEA (Tokyo, Japan) were used. All mice were given a week to adapt to a 12-h dark/light
cycle under constant temperature (22 ± 1 °C) with ad libitum access to food and water. This study was approved by the Institutional Animal Care and Use Committee of Tokyo Medical and Dental
University (TMDU) (approval number: 2021-259C, 2021-135C). We can confirm that all methods were performed in accordance with the relevant guidelines and regulations. All animal experiments
were conducted in strict accordance with the Animal Research Reporting of In Vivo Experiments (ARRIVE) guidelines and regulations for care and use of laboratory animals. EXPERIMENTAL DESIGN
AND SURGICAL PROCEDURE Three mice were used in a preliminary study to investigate the effects of gelatin hydrogel itself on bone formation (granule size of gelatin hydrogel: 50 µm-diameter
and 250 µm-length, the Japan Wool Textile Co., Ltd., Osaka, Japan). These three mice received an injection of gelatin hydrogel alone on the left side of the maxilla bone and were sacrificed
at four weeks after the injections. Micro CT images were obtained at the end of the experiment. Then a mixture of gelatin hydrogel fibre scaffolds, BMP-2 (Bioventus LLC, Durham, NC, USA),
and anabolic peptide (OP3-4; ABclonal Biotechnology, Woburn, MA, USA) was prepared for injection. The ratio of BMP-2 and OP3-4 was at a ratio described in a previous study31, BMP-2 (1 μg in
1 μl LF6 buffer) was mixed with gelatin hydrogel (0.6 mg) with OP3-4 (0.66 mg, dissolved in 5 μl dimethyl sulfoxide), and the mixture was incubated at room temperature for 4 h. A total of 12
eight-week-old male C57BL/6J mice were subcutaneously anaesthetized as previously described32. All the mice received a 2-μl submucosal injection on the buccal side of the right maxillary M1
using a 26-gauge, 15° tip Hamilton needle attached to a 25-μl Hamilton syringe (Fig. 5A). Due to the delicate nature of the mucosal tissue, the injection site where the needle was inserted
had to be placed approximately 2.0 mm proximal from bone formation site, namely the buccal alveolar bone of M1. Additionally, six mice were injected with gelatin hydrogel impregnated with
alizarin on the right buccal side of maxillary first molar, and the left side was injected with gelatin hydrogel without alizarin. The maxillae were excised and then obtained a fluorescent
image using in vivo imaging system (IVIS) (IVIS Lumina XRMS Series III, Revvity Inc., Waltham, MA, USA) to evaluate the distribution of the gelatin hydrogel injected to maxilla at 4 h and 3
days after the injections. Excitation and emission filters was 560/620 nm. Then undecalcified sections were made (Supplementary Fig. 5). Four weeks after the injection, a U-shaped tooth
movement appliance was applied to 50% of the randomly selected mice. The appliance was made with a nickel-titanium round alloy wire of 0.2 mm diameter and 10 mm length (Furukawa Electric Co.
Ltd., Tokyo, Japan). The wire was bent and adjusted using a dial tension gauge (DTN-10; TECLOCK, Tokyo, Japan) and bonded to the palatal surfaces of the right and left maxillary M1s using a
light-curing composite resin (G-FIX; GC, Tokyo, Japan) to exert a 10 cN reciprocal force toward the buccal side (Fig. 5. Direction shown as yellow arrow). All appliances were checked daily
throughout the period of force application. Since the applied force was still active until day 14, we did not reactivate the wire (Supplementary Fig. 6). Then, the mice were randomly divided
into four groups (n = 6 for each group): the control (Ctr) group , the injection (Inj) group , the force application (FA) group, and the injected group with force application (Inj/FA) group
(Fig. 5B). The sample size was determined using power analysis based on data from our previous pilot studies. On days 27 and 37, the mice were subcutaneously injected with the following
fluorescent reagents: calcein (20 mg/kg; Sigma-Aldrich Co., Ltd., St. Louis, MO, USA) and alizarin complexone (20 mg/kg; Dojindo, Kumamoto, Japan), and euthanised by cervical dislocation
under anaesthesia at week 6. The cranial bones were then dissected and fixed in phosphate-buffered saline (PBS)-based formaldehyde solution fixative (pH 7.4) for 18 h at 4 °C under constant
shaking motion. All the samples were washed and stored in PBS for radiological and histological analyses. RADIOLOGICAL ASSESSMENT After the second week after injection, in vivo μCT (R_mCT2
SPMD, Rigaku, Tokyo, Japan) imaging was performed weekly (90 kV, 160 μA, 10-mm field of view). 3D reconstruction images of the maxillae were captured and reconstructed using image analysis
software (TRI/3D-BON; Ratoc System Engineering, Tokyo, Japan), and arranged such that the occlusal plane was parallel to the floor and balanced between the left and right planes.
Two-dimensional images of the sagittal planes were first defined as the plane passing through the centre of the mesial root canal and the DBR canal, and then the coronal views were defined
as the plane passing through the DBR canal (Supplementary Fig. 7). Coronal sections were used to observe BMD changes (Fig. 1A). Then the same coronal views of the image data were blinded,
evaluated to measure the buccal alveolar bone thickness at the DBR site, region of interest (ROI) of 500 × 500 µm was set from the CEJ. Alveolar bone thickness was analysed using ImageJ
software (version 1.50 i; NIH, Bethesda, MD, USA). The change in alveolar bone thickness was calculated by subtracting the thickness at week 4 from week 5, and week 5 from week 6. Then, the
weekly thickness changes were divided into seven days to calculate the rate of change after force application. In the same coronal view, the buccal alveolar bone height was measured from the
most lingual aspect of the buccal bone at the level of the apex to the beginning of the high BMD (> 690 mg/cm3). The distance of tooth movement was calculated by subtracting the distance
between the midpalatal suture and the distobuccal cusp of M1 (Fig. 1F) at the start of force application (week 4) from 7-day force application (week 5) to 14-day force application (week 6)
after force application. HISTOLOGICAL ASSESSMENT AND BONE HISTOMORPHOMETRY The maxillae were cut just anterior to the M1 under a constant flow of water. The samples were then embedded in
SCEM compound (Section-Lab Co. Ltd, Hiroshima, Japan) and frozen into blocks at − 100 °C in a freezing system (UT2000F; Leica Microsystems, Wetzlar, Germany). Two- and 5-μm-thickness slices
of undecalcified frozen sections at DBR sites were prepared using a cryomicrotome (CM3050sIV; Leica Biosystems, Nussloch, Germany) according to Kawamoto's method with adhesive film
(Cryofilm type 2C(9), Section-Lab Co. Ltd), as described elsewhere42. Two-μm slices were stained according to the von Kossa staining method to detect the calcified tissue. Osteoclasts were
identified using TRAP staining with methyl green counterstaining32. Bone histomorphometry was performed at an ROI of 500 × 500 µm from the crest of the alveolar bone at the DBR section.
Osteoclasts were defined as TRAP-positive multinucleated cells with at least two nuclei on the bone surface, which was measured as the root-facing surface. Oc. S/BS and ES/BS were measured
and calculated. Fluorescence-labelled images were used to analyse bone formation activity in the alveolar bone. All sections (5-μm) were blinded, and viewed under a fluorescence microscope
(FSX100; Olympus, Tokyo, Japan). The MAR was calculated by measuring the inter-labelled distance between a calcein-labelled line (green) and an alizarin-labelled line (red) at 10-day
intervals. The area reference (BFR) was calculated by multiplying the mineralising surface (MS) by the MAR and then dividing by the bone volume43,44. Bone histomorphometric analyses were
performed using ImageJ software. STATISTICAL ANALYSIS All mice were used for the measurements, and no mice were excluded from data analysis. All statistical analyses were performed using the
SPSS ver. 25.0. Multiple intergroup comparisons were performed using one-way analysis of variance (ANOVA), followed by Tukey’s post-hoc test. Normality was analysed using the Shapiro–Wilk
test. When a significant F ratio was identified, the groups were compared using non-parametric analyses using the Kruskal–Walli’s test. The calculated _p_-values were adjusted using the
Bonferroni correction. Statistical significance was set at _p_ < 0.05 indicating statistical significance. All data are presented as means ± standard deviation (SD). DATA AVAILABILITY All
data associated with this study are provided within the main manuscript or a supplementary information file. REFERENCES * Freitas, R. M. _et al._ Alveolar ridge and maxillary sinus
augmentation using rhBMP-2: A systematic review. _Clin. Implant Dent. Relat. Res._ 17(Suppl 1), e192–e201. https://doi.org/10.1111/cid.12156 (2015). Article MathSciNet PubMed Google
Scholar * Fuhrmann, R. A. W. Three-dimensional evaluation of periodontal remodeling during orthodontic treatment. _Semin. Orthod._ 8, 23–28. https://doi.org/10.1053/sodo.2002.28168 (2002).
Article Google Scholar * Garib, D. G., Yatabe, M. S., Ozawa, T. O. & Silva Filho, O. G. D. S. Alveolar bone morphology under the perspective of the computed tomography: Defining the
biological limits of tooth movement. _Dental Press J. Orthod._ 15, 192–205. https://doi.org/10.1590/S2176-94512010000500023 (2010). Article Google Scholar * Lee, K. H., Cheon Lee, S., Jung
Kim, H., Kang, Y. G. & Kim, S. J. Effect of locally delivered protein complex-loaded nanoparticles on bone remodelling of atrophic alveolar ridge in beagles. _Orthod. Craniofac. Res._
25, 55–63. https://doi.org/10.1111/ocr.12487 (2022). Article PubMed Google Scholar * Löst, C. Depth of alveolar bone dehiscences in relation to gingival recessions. _J. Clin.
Periodontol._ 11, 583–589. https://doi.org/10.1111/j.1600-051x.1984.tb00911.x (1984). Article PubMed Google Scholar * Buyuk, S. K., Ercan, E., Celikoglu, M., Sekerci, A. E. &
Hatipoglu, M. Evaluation of dehiscence and fenestration in adolescent patients affected by unilateral cleft lip and palate: A retrospective cone beam computed tomography study. _Angle
Orthod._ 86, 431–436. https://doi.org/10.2319/042715-289.1 (2016). Article PubMed PubMed Central Google Scholar * Artun, J. & Urbye, K. S. The effect of orthodontic treatment on
periodontal bone support in patients with advanced loss of marginal periodontium. _Am. J. Orthod. Dentofac. Orthop._ 93, 143–148. https://doi.org/10.1016/0889-5406(88)90292-2 (1988). Article
CAS Google Scholar * Wennström, J. L., Stokland, B. L., Nyman, S. & Thilander, B. Periodontal tissue response to orthodontic movement of teeth with infrabony pockets. _Am. J. Orthod.
Dentofac. Orthop._ 103, 313–319. https://doi.org/10.1016/0889-5406(93)70011-C (1993). Article Google Scholar * Garib, D. G., Henriques, J. F., Janson, G., de Freitas, M. R. &
Fernandes, A. Y. Periodontal effects of rapid maxillary expansion with tooth-tissue-borne and tooth-borne expanders: A computed tomography evaluation. _Am. J. Orthod. Dentofac. Orthop._ 129,
749–758. https://doi.org/10.1016/j.ajodo.2006.02.021 (2006). Article Google Scholar * Baysal, A. _et al._ Evaluation of alveolar bone loss following rapid maxillary expansion using
cone-beam computed tomography. _Korean J. Orthod._ 43, 83–95. https://doi.org/10.4041/kjod.2013.43.2.83 (2013). Article PubMed PubMed Central Google Scholar * Taner, T. U., Germec, D.,
Er, N. & Tulunoglu, I. Interdisciplinary treatment of an adult patient with old extraction sites. _Angle Orthod._ 76, 1066–1073. https://doi.org/10.2319/082505-301 (2006). Article
PubMed Google Scholar * Tan, W. L., Wong, T. L., Wong, M. C. & Lang, N. P. Systematic review of post-extractional alveolar hard and soft tissue dimensional changes in humans. _Clin.
Oral Implants Res._ 23(Suppl 5), 1–21. https://doi.org/10.1111/j.1600-0501.2011.02375.x (2012). Article PubMed Google Scholar * Ramos, A. L., Dos Santos, M. C., de Almeida, M. R. &
Mir, C. F. Bone dehiscence formation during orthodontic tooth movement through atrophic alveolar ridges. _Angle Orthod._ 90, 321–329. https://doi.org/10.2319/063019-443.1 (2020). Article
PubMed Google Scholar * Kessler, M. Interrelationships between orthodontics and periodontics. _Am. J. Orthod._ 70, 154–172. https://doi.org/10.1016/s0002-9416(76)90316-x (1976). Article
CAS PubMed Google Scholar * Santos, P. B. D. D. _et al._ Movement of mandibular molar into edentulous alveolar ridge: A cone-beam computed tomography study. _Am. J. Orthod. Dentofac.
Orthop._ 151, 907–913. https://doi.org/10.1016/j.ajodo.2016.10.024 (2017). Article Google Scholar * Sarikaya, S., Haydar, B., Ciğer, S. & Ariyürek, M. Changes in alveolar bone
thickness due to retraction of anterior teeth. _Am. J. Orthod. Dentofac. Orthop._ 122, 15–26. https://doi.org/10.1067/mod.2002.119804 (2002). Article Google Scholar * Wainwright, W. M.
Faciolingual tooth movement its influence on the root and cortical plate. _Am. J. Orthod._ 64, 278–302. https://doi.org/10.1016/0002-9416(73)90021-3 (1973). Article CAS PubMed Google
Scholar * Ma, H. _et al._ Augmented corticotomy-assisted presurgical orthodontic treatment to prevent alveolar bone loss in patients with skeletal Class III malocclusion. _Am. J. Orthod.
Dentofac. Orthop._ 163, 210–221. https://doi.org/10.1016/j.ajodo.2021.10.021 (2023). Article Google Scholar * Araújo, M. G., Carmagnola, D., Berglundh, T., Thilander, B. & Lindhe, J.
Orthodontic movement in bone defects augmented with Bio-Oss: An experimental study in dogs. _J. Clin. Periodontol._ 28, 73–80. https://doi.org/10.1034/j.1600-051x.2001.280111.x (2001).
Article PubMed Google Scholar * Wilcko, M. T., Wilcko, W. M., Pulver, J. J., Bissada, N. F. & Bouquot, J. E. Accelerated osteogenic orthodontics technique: A 1-stage surgically
facilitated rapid orthodontic technique with alveolar augmentation. _J. Oral Maxillofac. Surg._ 67, 2149–2159. https://doi.org/10.1016/j.joms.2009.04.095 (2009). Article PubMed Google
Scholar * Zhang, D. _et al._ Orthodontic tooth movement in alveolar cleft repaired with a tissue engineering bone: An experimental study in dogs. _Tissue Eng. Part A_ 17, 1313–1325.
https://doi.org/10.1089/ten.TEA.2010.0490 (2011). Article CAS PubMed Google Scholar * Arrington, E. D., Smith, W. J., Chambers, H. G., Bucknell, A. L. & Davino, N. A. Complications
of iliac crest bone graft harvesting. _Clin. Orthop. Relat. Res._ 329, 300–309. https://doi.org/10.1097/00003086-199608000-00037 (1996). Article Google Scholar * Yamada, Y. _et al._
Injectable bone tissue engineering using expanded mesenchymal stem cells. _Stem Cells_ 31, 572–580. https://doi.org/10.1002/stem.1300 (2013). Article CAS PubMed Google Scholar * Ru, N.
_et al._ BoneCeramic graft regenerates alveolar defects but slows orthodontic tooth movement with less root resorption. _Am. J. Orthod. Dentofac. Orthop._ 149, 523–532.
https://doi.org/10.1016/j.ajodo.2015.09.027 (2016). Article Google Scholar * Kuroda, Y., Kawai, T., Goto, K. & Matsuda, S. Clinical application of injectable growth factor for bone
regeneration: A systematic review. _Inflamm. Regen._ 39, 20. https://doi.org/10.1186/s41232-019-0109-x (2019). Article CAS PubMed PubMed Central Google Scholar * Notodihardjo, F. Z.,
Kakudo, N., Kushida, S., Suzuki, K. & Kusumoto, K. Bone regeneration with BMP-2 and hydroxyapatite in critical-size calvarial defects in rats. _J. Craniomaxillofac. Surg._ 40, 287–291.
https://doi.org/10.1016/j.jcms.2011.04.008 (2012). Article PubMed Google Scholar * Başçıl, S., Turhan İyidir, Ö., Bayraktar, N., Ertörer, M. E. & Başçıl Tütüncü, N. Severe chronic
periodontitis is not common in Acromegaly: Potential protective role of gingival BMP-2. _Turk. J. Med. Sci._ 51, 1172–1178. https://doi.org/10.3906/sag-2006-93 (2021). Article CAS PubMed
PubMed Central Google Scholar * Arai, Y. _et al._ Peptide-induced de novo bone formation after tooth extraction prevents alveolar bone loss in a murine tooth extraction model. _Eur. J.
Pharmacol._ 782, 89–97. https://doi.org/10.1016/j.ejphar.2016.04.049 (2016). Article CAS PubMed Google Scholar * Sugamori, Y. _et al._ Peptide drugs accelerate BMP-2-induced calvarial
bone regeneration and stimulate osteoblast differentiation through mTORC1 signaling. _BioEssays_ 38, 717–725. https://doi.org/10.1002/bies.201600104 (2016). Article CAS PubMed PubMed
Central Google Scholar * Rashed, F. _et al._ The effects of receptor activator of NF-κB ligand-binding peptides on bone resorption and bone formation. _Front. Cell Dev. Biol._ 9, 648084.
https://doi.org/10.3389/fcell.2021.648084 (2021). Article PubMed PubMed Central Google Scholar * Uehara, T. _et al._ Delivery of RANKL-binding peptide OP3-4 promotes BMP-2-induced
maxillary bone regeneration. _J. Dent. Res._ 95, 665–672. https://doi.org/10.1177/0022034516633170 (2016). Article CAS PubMed Google Scholar * Keo, P. _et al._ A pilot study to
investigate the histomorphometric changes of murine maxillary bone around the site of mini-screw insertion in regenerated bone induced by anabolic reagents. _Eur. J. Orthod._ 43, 86–93.
https://doi.org/10.1093/ejo/cjaa018 (2021). Article PubMed Google Scholar * Zeitounlouian, T. S., Zeno, K. G., Brad, B. A. & Haddad, R. A. Three-dimensional evaluation of the effects
of injectable platelet rich fibrin (i-PRF) on alveolar bone and root length during orthodontic treatment: A randomized split mouth trial. _BMC Oral Health_ 21, 92.
https://doi.org/10.1186/s12903-021-01456-9 (2021). Article CAS PubMed PubMed Central Google Scholar * Evangelista, K. _et al._ Dehiscence and fenestration in patients with Class I and
Class II Division 1 malocclusion assessed with cone-beam computed tomography. _Am. J. Orthod. Dentofac. Orthop._ 138, 133.e1–7. https://doi.org/10.1016/j.ajodo.2010.02.021 (2010) (DISCUSSION
133). Article Google Scholar * Little, R. M. Stability and relapse of mandibular anterior alignment: University of Washington studies. _Semin. Orthod._ 5, 191–204.
https://doi.org/10.1016/s1073-8746(99)80010-3 (1999). Article CAS PubMed Google Scholar * Enhos, S. _et al._ Dehiscence and fenestration in patients with different vertical growth
patterns assessed with cone-beam computed tomography. _Angle Orthod._ 82, 868–874. https://doi.org/10.2319/111211-702.1 (2012). Article PubMed PubMed Central Google Scholar * Reichert,
C., Götz, W., Smeets, R., Wenghöfer, M. & Jäger, A. The impact of nonautogenous bone graft on orthodontic treatment. _Quintessence Int._ 41, 665–672 (2010). PubMed Google Scholar *
Ritwiroon, N., Suzuki, B. & Suzuki, E. Y. Effects of alveolar bone width and density on the rate of orthodontic tooth movement. _J. Dent. Assoc. Thai._ 71, 53–63 (2021). Google Scholar
* Huang, H., Williams, R. C. & Kyrkanides, S. Accelerated orthodontic tooth movement: Molecular mechanisms. _Am. J. Orthod. Dentofac. Orthop._ 146, 620–632.
https://doi.org/10.1016/j.ajodo.2014.07.007 (2014). Article Google Scholar * Alaa, S., Fouda, A. M., Grawish, M. E. & Abdelnaby, Y. L. The effect of submucosal injection of
platelet-rich fibrin vs. platelet-rich plasma on orthodontic tooth movement in rabbits; 28 days follow-up. _Int. Orthod._ 21, 100715. https://doi.org/10.1016/j.ortho.2022.100715 (2023).
Article PubMed Google Scholar * Alomari, E. B. & Sultan, K. Efficacy of injectable platelet-rich plasma in reducing alveolar bone resorption following rapid maxillary expansion: A
cone-beam computed tomography assessment in a randomized split-mouth controlled trial. _Angle Orthod._ 89, 705–712. https://doi.org/10.2319/091018-661.1 (2019). Article PubMed PubMed
Central Google Scholar * Kawamoto, T. Use of a new adhesive film for the preparation of multi-purpose fresh-frozen sections from hard tissues, whole-animals, insects and plants. _Arch.
Histol. Cytol._ 66, 123–143. https://doi.org/10.1679/aohc.66.123 (2003). Article PubMed Google Scholar * Dempster, D. W. _et al._ Standardized nomenclature, symbols, and units for bone
histomorphometry: A 2012 update of the report of the ASBMR Histomorphometry Nomenclature Committee. _J. Bone Miner. Res._ 28, 2–17. https://doi.org/10.1002/jbmr.1805 (2013). Article PubMed
Google Scholar * Takagi, M. _et al._ High-turnover periprosthetic bone remodeling and immature bone formation around loose cemented total hip joints. _J. Bone Miner. Res._ 16, 79–88.
https://doi.org/10.1359/jbmr.2001.16.1.79 (2001). Article CAS PubMed Google Scholar Download references ACKNOWLEDGEMENTS We thank Eri Tagawa, Masao Oi, and Naoki Shimada (Japan Wool
Textile Co., Ltd., Osaka, Japan) for providing the gelatin hydrogel scaffolds. This work was supported by the Japan Society for the Promotion of Science (JSPS) Grants-in-Aid for Scientific
Research (KAKENHI) (grant numbers 19H01068, 20K10199, 20K10222 and 24K13171). We would like to thank Editage (http://www.editage.com) for English language editing. AUTHOR INFORMATION Author
notes * Yoshiro Matsumoto Present address: Department of Orthodontic Science, Graduate School of Medical and Dental Sciences, Tokyo Medical and Dental University (TMDU), Tokyo, Japan AUTHORS
AND AFFILIATIONS * Department of Orthodontic Science, Graduate School of Medical and Dental Sciences, Tokyo Medical and Dental University (TMDU), Tokyo, Japan Jia Qi & Takashi Ono *
Department of Basic Oral Health Engineering, Graduate School of Medical and Dental Sciences, Tokyo Medical and Dental University (TMDU), Tokyo, Japan Jia Qi, Cangyou Xie, Fatma Rashed &
Kazuhiro Aoki * Department of Oral Pathology, Graduate School of Medical and Dental Sciences, Tokyo Medical and Dental University (TMDU), Tokyo, Japan Cangyou Xie * Department of Oral
Biology, Faculty of Dentistry, Damanhour University, Damanhour, 22511, Egypt Fatma Rashed Authors * Jia Qi View author publications You can also search for this author inPubMed Google
Scholar * Yoshiro Matsumoto View author publications You can also search for this author inPubMed Google Scholar * Cangyou Xie View author publications You can also search for this author
inPubMed Google Scholar * Fatma Rashed View author publications You can also search for this author inPubMed Google Scholar * Takashi Ono View author publications You can also search for
this author inPubMed Google Scholar * Kazuhiro Aoki View author publications You can also search for this author inPubMed Google Scholar CONTRIBUTIONS J. Q. and Y. M. contributed to the
conception, design, data acquisition, and interpretation; performed all statistical analyses; and drafted and critically revised the manuscript; C. X. and F. R. contributed to data
acquisition and analysis and critically revised the manuscript; K. A. and T. O. contributed to the conception, design, data acquisition, and interpretation and critically revised the
manuscript. All authors provided their final approval and agreed to be accountable for all aspects of this study. CORRESPONDING AUTHOR Correspondence to Yoshiro Matsumoto. ETHICS
DECLARATIONS COMPETING INTERESTS The authors declare no competing interests. ADDITIONAL INFORMATION PUBLISHER'S NOTE Springer Nature remains neutral with regard to jurisdictional claims
in published maps and institutional affiliations. SUPPLEMENTARY INFORMATION SUPPLEMENTARY FIGURES. RIGHTS AND PERMISSIONS OPEN ACCESS This article is licensed under a Creative Commons
Attribution 4.0 International License, which permits use, sharing, adaptation, distribution and reproduction in any medium or format, as long as you give appropriate credit to the original
author(s) and the source, provide a link to the Creative Commons licence, and indicate if changes were made. The images or other third party material in this article are included in the
article's Creative Commons licence, unless indicated otherwise in a credit line to the material. If material is not included in the article's Creative Commons licence and your
intended use is not permitted by statutory regulation or exceeds the permitted use, you will need to obtain permission directly from the copyright holder. To view a copy of this licence,
visit http://creativecommons.org/licenses/by/4.0/. Reprints and permissions ABOUT THIS ARTICLE CITE THIS ARTICLE Qi, J., Matsumoto, Y., Xie, C. _et al._ Prevention of bone dehiscence
associated with orthodontic tooth movement by prophylactic injection of bone anabolic agents in mice. _Sci Rep_ 14, 15749 (2024). https://doi.org/10.1038/s41598-024-66617-6 Download citation
* Received: 28 March 2024 * Accepted: 02 July 2024 * Published: 08 July 2024 * DOI: https://doi.org/10.1038/s41598-024-66617-6 SHARE THIS ARTICLE Anyone you share the following link with
will be able to read this content: Get shareable link Sorry, a shareable link is not currently available for this article. Copy to clipboard Provided by the Springer Nature SharedIt
content-sharing initiative KEYWORDS * Alveolar bone loss * Bone regeneration * OP3-4 peptide * Drug delivery systems * Orthodontic tooth movement * Bone remodelling