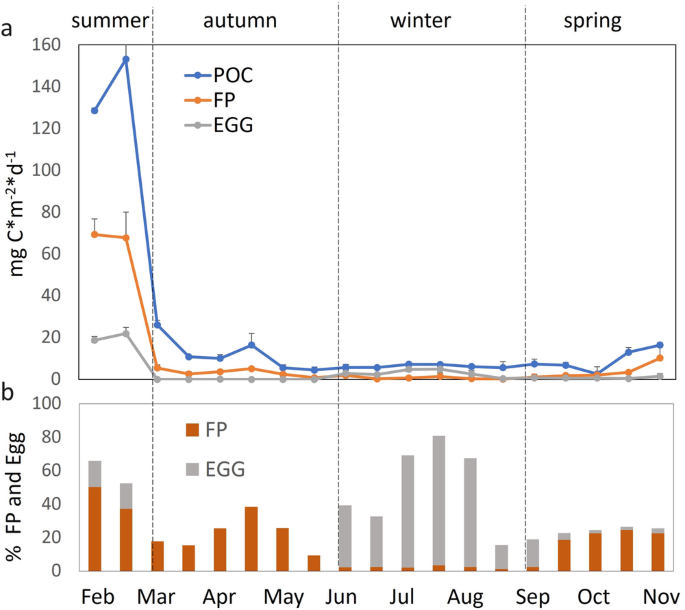
Life strategy of antarctic silverfish promote large carbon export in terra nova bay, ross sea
- Select a language for the TTS:
- UK English Female
- UK English Male
- US English Female
- US English Male
- Australian Female
- Australian Male
- Language selected: (auto detect) - EN
Play all audios:

ABSTRACT Antarctic silverfish _Pleuragramma antarcticum_ is the most abundant pelagic fish in the High Antarctic shelf waters of the Southern Ocean, where it plays a pivotal role in the
trophic web as the major link between lower and higher trophic levels. Despite the ecological importance of this species, knowledge about its role in the biogeochemical cycle is poor. We
determine the seasonal contribution of Antarctic silverfish to carbon flux in terms of faeces and eggs, from samples collected in the Ross Sea. We find that eggs and faeces production
generate a flux accounting for 41% of annual POC flux and that the variability of this flux is modulated by spawning strategy. This study shows the important role of this organism as a
vector for carbon flux. Since Antarctic silverfish are strongly dependent on sea-ice, they might be especially sensitive to climatic changes. Our results suggest that a potential decrease in
the biomass of this organism is likely to impact marine biogeochemical cycles, and this should be factored in when assessing Southern Ocean carbon budget. SIMILAR CONTENT BEING VIEWED BY
OTHERS CONTINUOUS MOULTING BY ANTARCTIC KRILL DRIVES MAJOR PULSES OF CARBON EXPORT IN THE NORTH SCOTIA SEA, SOUTHERN OCEAN Article Open access 27 November 2020 INTERANNUAL VARIABILITY IN
EARLY LIFE PHENOLOGY IS DRIVEN BY CLIMATE AND OCEANIC PROCESSES IN TWO NE ATLANTIC FLATFISHES Article Open access 11 March 2023 THE PELAGIC FOOD WEB OF THE WESTERN ADRIATIC SEA: A FOCUS ON
THE ROLE OF SMALL PELAGICS Article Open access 04 September 2023 INTRODUCTION The world’s oceans are estimated to absorb about 25% of the total CO2 emissions from the atmosphere every year1,
contributing to climate mitigation. This process is supported by the Biological Carbon Pump, the fixation of inorganic carbon (C) through photosynthesis by phytoplankton, and subsequent C
export and sequestration to deeper waters. Recent interest in the role of fish in biogeochemical cycles has highlighted the need for better parameterisations of C export generated by these
organisms2. Despite the global ecological and economic significance of fishes, observations, and process studies of their contributions to the ocean carbon export are poor3,4. However, due
to the high abundance of epipelagic fishes on coastal shelves and in upwelling regions, and the high biomass of mesopelagic fishes in the vast expanse of oligotrophic regions, their
contribution is likely to be important and has recently estimated as 16% of total carbon flux5. The Southern Ocean plays a pivotal role in the global carbon cycle and in the global C
uptake6,7,8. Rapidly sinking faecal pellets generated from zooplankton are a major contributor to the biological pump and the export of carbon9,10,11,12. However, studies from other regions
show that sinking of large faecal pellets produced by fish can reach over thousands of metres per day13,14 which is generally one-two order of magnitude higher of the sinking speed of
zooplankton faeces. Moreover, the cohesive nature of fish faecal pellets suggests that they are resistant to bacterial decomposition which combine with high sinking rates facilitate their
descent to the benthos14. The Antarctic silverfish _Pleuragramma antarcticum_ is a nototheniid species widely distributed in waters of the continental shelf in High-Antarctic regions. It is
abundant, accounting for about 98% by number of the total ichthyoplankton on Weddell Sea continental shelf and accounts for over 90% of the pelagic fish biomass in the coastal area of the
Ross Sea15,16,17,18. _P. antarcticum_ is a key species in the marine trophic web, since it plays a pivotal role in the trophic structure of the High-Antarctic coastal ecosystems by
channelling energy flow between plankton and the top predator community19,20,21. It feeds largely on zooplankton, but has shown a flexible and opportunistic trophic strategy, relying on the
wide variety of planktonic prey22. As a prey, P. antarctica provides a high energetic value23 and occurs in the stomach of predators ranging from large fishes, like the Antarctic toothfish,
to penguins, marine flying birds, and seals21,24. Among notothenioids, _P. antarcticum_ is the only known holopelagic species, living all stages of its development within the water column,
exhibiting a complex lifestyle: eggs develop under fast ice, larvae and juveniles disperse over the continental shelves and adults live in the coastal areas around the Antarctic
continent17,18. Given its abundance and ecologic role, the Antarctic silverfish has been extensively studied on many aspects of its biology and ecology17,18. Nevertheless, quantitative
knowledge on the role this organism plays in the biogeochemical cycle is absent. Here, we determine the seasonal contribution of Antarctic silverfish to the flux of carbon as faeces and eggs
from samples collected by a moored sediment trap deployed for one year on inshore area of Terra Nova Bay, the Ross Sea, in the Western sector of the Southern Ocean. This region is
considered the only nursery and hatching area of the Antarctic silverfish where huge quantities of embryonated eggs can be detected floating in huge amounts among the subsurface platelet ice
layer under sea ice18,25. Platelet ice consists of various-sized flat plate-like crystals up to above 10 cm in diameter, randomly oriented, mostly occurring under the coastal cover26,27.
Platelet ice is found as a component of fast-ice cores, at depths larger than 1 m28, making up a semi-consolidated layer ranging from a few centimetres to metres in thickness29. Due to its
prominent occurrence under the fast ice, platelet ice is considered a coastline high-latitude feature, although its real extent is still unknown20. The surface ice platelet layer is highly
porous, which facilitates nutrient exchange with seawater and supports exceptionally high algal biomass29,30. The quantifying of energetic fluxes among the biota, pack ice, platelet layer,
and free sea ice water are of scientific interest for understanding the high Antarctic carbon cycle31. This study provides new insights on the importance of the Antarctic silverfish as a
vector for C export in a region, such as the Ross Sea, that contributes significantly to global atmospheric C uptake32. We found that both eggs and faeces can significantly contribute to the
carbon export in this region. As during the entire life cycle the Antarctic silverfish appear to be strongly dependent on sea ice, this species would be especially sensitive to climatic or
oceanic changes that reduce the extent and alter the dynamic of sea-ice cover17,20. To increase confidence in forecasting the effects of climate change on the biogeochemical cycle, the
influence of a changing climate on Antarctic fish must be taken into account when assessing Southern Ocean carbon budget. RESULTS EGGS AND FAECES CONTRIBUTION TO THE POC FLUX The trend of
Particulate Organic Carbon (POC) flux showed a strong seasonal variability, with values ranging between 5.61 and 153.21 mg C *m−2 *d−1 (Fig. 1a). POC flux is at a maximum in the summer
(February), after which there is a decrease to a low winter level (June to September) until it increases again in the late spring (October onwards). The relative contribution of the
different fish components to total POC flux varies between seasons (Fig. 1b). Eggs and FPs both strongly contributed to the POC fluxes in the summer (accounting together for up to 65.3%)
while in the autumn and spring POC contribution is largely characterised only by FPs. Conversely, eggs become the dominant contributor in the winter periods (up to 77.2% of total POC flux)
(see Supplementary Table 1 for POC, FP and Egg flux data). Out of an annual total POC flux FPs make the highest contribution (25.3%), compared to eggs (15.2%). FP and egg carbon content
significant varies between the seasons (FP: _z_ = −2.8, _p_ < 0.05; Egg: _z_ = −2.1, _p_ < 0.05). In general, FP C values range between 10.9–22.5 µg C mm−3 and are at their lowest
during the autumn–winter period and highest during spring-summer. Conversely, the carbon contents of eggs present highest values during the winter (55.6 µg C egg−1) and lowest during the
summer (45.4 µg C egg−1) (Table 1). SEASONAL VARIABILITY OF EGGS AND FAECES SIZE All the fish faeces collected in the sediment trap present a light brownish colour and are shaped like long
cylindrical, most of them are in the form of fragment with different length ranging from 2.3 to 7.5 mm and were similar in width (0.9–1.3 mm) (Fig. 2a). There was a significant amount of
small FP fragments with small size (>60%, up 4.5 mm) in the spring/summer than in autumn/winter (<40%, up 7 mm) (_z_ = −2.8, _p_ < 0.05). Eggs present a spherical shape with a size
between 1.8–2.5 mm (Fig. 2b). Eggs present a significant seasonal variability with a large diameter in the summer and spring (>54%, up 2.5 mm) than in the winter (_z_ = _−2.9_, _p_ <
0.05) (see Supplementary Table 2 for fish FP and egg class size data). PRESERVATION STATE OF EGGS During the winter to the beginning of the spring period most of the eggs (up 80%) present a
good preservation state with no sign of decomposition, conversely in the summer a high percentage (100%) of eggs revel clear sign of degradation with the presence of physical decay (Fig. 3).
In particular, in the most of the eggs present chorion damage (see Supplementary Table 3 for fish egg degradation data). DISCUSSION We found that Antarctic silverfish can strongly
contribute to the POC flux in the region of Terra Nova Bay (TNB), in the Western Ross Sea. Their contribution is mainly generated from the faecal pellets and eggs, which together, comprised
41% of the annual POC export in this study region. Hence, we suggest that the unique features of Antarctic silverfish promote the strongest carbon sink in the Southern Ocean regions where
this organism spawn (such as TNB). The potential important role of fish in promoting C flux has already been highlighted by ref. 5, who estimated based on a synthesis of passive (faecal
pellet sinking) and active (migratory) flux of fishes, a contribution of about 16% to total global carbon flux out of the euphotic zone. To the best of our knowledge this is the only study
focuses on the role that fish FPs are playing in the Southern Ocean C export. Further, no previous studies (in the Southern Ocean or elsewhere) have investigated the impact of eggs. Here we
show that eggs can provide 15% contribution to the annual POC, indicating that estimation of global fish input to the carbon cycle need also consider this component. In addition, the
relatively large contributions of Antarctic silverfish eggs to the POC in the winter (>60%), promote a relative high pulse of carbon flux in this season (always >5 mg/m2/d) which is
comparable to POC flux value observed during the productive period (i.e. spring-summer) in the Ross Sea region33,34,35. Therefore, the winter eggs flux may be an important source of
nutrition to fuel the benthos. The highest egg POC flux is observed in the summer (21 mg*m−2*d−1). Eggs size is significant higher during this period compared to winter, showing an advanced
stage of embryo development. Previous studies have suggested that spawning occurred in the winter (July–August) and hatching in spring-summer25,36. We suggest that eggs collected in the
sediment trap in this period (summer) died before reaching the hatching phase and then start to sink. In fact, most of the sunk eggs (85%) in the summer show evidence of chorion damage and
all of them show an advanced state of degradation. Further, incubation experiments show that eggs with an intact chorion could survive and remain viable at temperatures as low as −9 °C but
once the chorion is breached by ice, freezing is instantaneous37. Pelagic eggs owe their buoyancy almost exclusively (90%) to the high-water content, whereas lipid accounts for only about
10%38. Thus, chorion damage can result in a change of the water-lipid balance and lead to the loss of the eggs’ neutral balance. Conversely, in the winter, eggs are pristine and smaller,
suggesting they start to sink soon after spawning takes place. Part of the egg mass that sink in the winter might be a result of incomplete fertilisation, despite we cannot exclude that
fully fertilised eggs just die relatively soon after they are released. Other mechanisms could impact the ability of fish eggs to maintain neutral buoyancy. Some experiment shows that the
quality of fish eggs decreases with batch number39, which in turn could decrease the chance of eggs survival and increase the number of sinking eggs40. Further, we find that carbon specific
content in eggs is higher in the winter (55.6 µg*egg −1) and lower (45.4 µg*egg−1) in the summer. The seasonal variability in _P. antarcticum_ eggs carbon content agrees with previous
experiments carried out on eggs of _Gadus morhua_41. The authors show that C content of incubated eggs decrease by about 14% from the newly fertilised eggs until just before hatching since
the yolk sac is used to fuel energy for the forming larvae. The egg size after fertilisation of the Antarctic silverfish reported in literature (1.8–2.0 mm)42 is the same range of the eggs
size observed in the winter. Together, both features (relatively high C content and size range) of the eggs collected during the winter suggest that these are most likely dead-fertilised
eggs. Eggs are almost absent in the trap during autumn. This could be explained by adult Antarctic silverfish behaviour which are selecting their spawning habitat during autumn when sea ice
is also beginning to advance43. Maximum flux of FP-POC (69 mg/m2/d) is one order of magnitude lower comparing to maximum value observed in previous studies carried out on Northern anchovy
(i.e. 251 mg C m−2 d−1)14 which can be related to the behaviour of this fish to school and then aggregate in large number. Conversely Antarctic silverfish exist as loose shoals44
(unstructured aggregations), with individuals estimated to be spaced 2–4 m apart at densities of one fish per 7–43 m3, despite in the Weddell Sea, it have been occasionally observed in dense
aggregations45. We observed a significant decrease in FPs fragment size in spring and summer. This may be, in part, a result of the highest biological activities (i.e. presence of
zooplankton) in the water column during this period which could increase the FPs fragmentation and in turn remineralization process. This is also supported by the presence of large number of
zooplankton FPs observed in the same samples during spring-summer (Manno _personal observation_). Conversely the large size fragment (but low number) of FPs observed in winter reflect the
decrease of physiological activity of fish during spawning, resulting in a seasonal reduction in feeding because most of the energy is devoted to mature eggs. Moreover, in the winter a low
productivity water column allows fish FPs more likely to sink without encountering too many biological barriers. The continuous presence of Antarctic silverfish FPs over the sampled period
in the sediment trap is an agreement with the ecology of this organism which, despite being a pelagic fish, is mainly a relatively inactive species and does not form dense continuously
swimming schools17. FPs contains almost the double amount of carbon in the spring/summer compared to winter, reflecting the variability in food availability and quality throughout the
seasons9 find a similar trend in the Scotia Sea (Atlantic Sector of the Southern Ocean) where FPs produced by zooplankton present twice the amount of carbon in the productive season than in
the winter. The present study illustrates the important role that _P. antarcticum_ can play in driving the sinking flux of POC in the Southern Ocean, a region which contributes significantly
to the global C export production32. We find that Antarctic silverfish in Terra Nova Bay account for 41% of annual POC flux (in term of eggs and faeces) and that the variability of this
flux is modulated by spawning strategy. The schematic (Fig. 4) summarises the mean contribution for each season of fish egg and FP to the POC. As well as supporting the findings of other
studies regarding the important contribution of fish FPs13,14 in different regions, this study further identifies the major contributions made by sinking eggs. In addition to being a major
source of sequestration, both FP and eggs flux can also be a major driver of productivity in benthic communities, particularly in shelf regions which are their specie’s spawning area.
Further, this study adds new insight in the Antarctic silverfish reproduction strategy by providing the first year-round observation of eggs flux. Increasing water temperature in the Terra
Nova Bay (and in general in the Southern Ocean), as a result of climate change, will likely have a negative impact on fish population43. The Antarctic silverfish have evolved a suite of
specific ecological and physiological adaptations to the environmental conditions in the cold and highly seasonal Antarctic continental shelf43,46. Therefore, a sudden change in
environmental conditions driven by the current rapid climate change could negatively affect this weak equilibrium. Previous studies have already shown declines in local populations of the
Antarctic silverfish47. Our results suggest that a potential decrease in fish biomass is likely to impact not only the Antarctic food web but also the marine carbon budget. To connect
climate variability, fish population dynamic and carbon cycle is then fundamental to increase confidence in forecasting the effect of climate change. Furthermore, the role of this organism
in the biogeochemical cycle should be also taken into account (in addition to the role in the food web) to improve conservation measure and policy. We are aware that other fish species might
represent an unknown fraction of the samples found in the sediment trap. However, overall, our results highlight the relevance of pelagic fishes as an important biological vector of carbon
export in this region. Further, we assume that the large majority of the observed eggs and faeces are likely being produced by Antarctic silverfish because of contrasting feeding/spawning
behaviour of some of the most common fishes in this region (i.e. _C. hamatus, T. bernacchii_ and _T. newnesi_)42 in combination with the position of the mooring (located in the nursery and
hatching area of this organism). Future research focus on genetic analyses of eggs and faeces will be crucial to fully discriminate and quantify the individual carbon export contribution of
key fish species in the Southern Ocean. METHODS STUDY AREA TNB is located in the western sector of the Ross Sea, along the Victoria Land coast (Fig. 5). The most important feature of TNB is
a persistent coastal latent heat polynya48,49. The Terra Nova Bay polynya acts as an ice factory during winter and has a primary function in the sea-ice local dynamics50,51. The strong
katabatic winds blowing offshore form polynya as they advect the newly formed ice far from the coast52. As a result, a major environmental feature of TNB is the seasonal sea-ice cover,
bordering coastal areas for almost 9 months of the year53. The polynya of TNB is known to play a crucial role in the formation of the High Salinity Shelf Water the densest water mass of the
Southern Ocean, that directly contributes to the oceanic bottom water formation54,55. SEDIMENT TRAP SAMPLING Sediment trap samples are collected by a Hydro-Bios Multi Sediment Trap MST 24,
equipped with 24 receiving cups and a collecting area of 0.6 m2. The traps were deployed in TNB Polynya (mooring L: 74°44.6048′S, 164°8.3916′E, bottom 145 m) at 100 m depth during 1999. The
sample carousel of the sediment trap is set up to rotate at intervals of 15 days over the whole year. Each trap is fitted with a plastic baffle mounted in the opening, to prevent the
entrance of large-sized organisms. In the receiving cups, 4% buffered formalin-seawater solution is used as preservative. Upon recovery, samples are stored at ∼2–4 °C in the dark until
further analysis. The mooring line is equipped with an oceanographic instrument to monitoring currents (Aanderaa RCM7) and a conductivity/temperature/depth logger (CTD, SBE37 Microcat).
Details of hydrographic conditions at the mooring site have been published in ref. 56. POC, FPS AND EGGS ANALYSIS Each sample is split accurately into a series of pseudo-replicate fractions,
for subsequent analysis, following the technique of ref. 57. Prior to splitting, ‘swimmers’, i.e. zooplanktonic organisms that can enter the receiving cups while alive, are carefully
removed. For this purpose, samples are first wet-sieved, through a 1 mm nylon mesh and the remaining swimmers are carefully removed by hand-picking under a dissecting microscope (dissecting
microscope Olympus SZX16). Replicate fractions are vacuum filtered through pre-weighed and pre-combusted (550 °C for 5 h) 25 mm glass fibre filters (GF/F, nominal pore size 0.7 μm). For POC
determination, filters are pre-treated with 2 N H3PO4 and 1 N HCl. Filters are then desalted through briefly washing with distilled water and dried at 60 °C. POC were measured by combustion
in an elemental analyser. CE Instruments NA2500 elemental analyser, was calibrated using an acetanilide calibration standard with a known % C of 71.09%. Standards are interspersed regularly
between samples to measure and correct for drift. Analytical precision is better than 1.0%34. Fish eggs and fish faeces are measured and counted using an ocular micrometre under light
microscopy (dissecting microscope Olympus SZX16 and Leica M165 stereomicroscope). Fish eggs are identified according to ref. 25. For each sample 40 fish eggs and 40 fish faeces are randomly
picked up for the calculation of carbon content by following the same protocol as for POC analyses. POC, FP and egg fluxes are expressed in mg C m−2 d−1, estimated by dividing the total mass
per sample by the time interval and the trap collection area. The carbon contribution is calculated as % of eggs and fish carbon to the total POC flux. Dead eggs are identified as
indicative of the natural mortality of eggs (i.e. caused by genetic abnormalities and incomplete fertilisation) and classified on the base of two stages of degradation (1-early
decomposition, 2-physical decay) following the description of ref. 58. STATISTICAL ANALYSIS Wilcoxon signed-rank test is used to determine whether there are any significant differences with
regards to the different flux components within the sampling periods (in terms of variability in class size and carbon content of both FPs and eggs). Differences are considered significant
where _p_ < 0.05. Benjamini-Hochberg correction was applied for multiple comparisons. All the analyses were performed using the R software version 3.4.2. REPORTING SUMMARY Further
information on research design is available in the Nature Portfolio Reporting Summary linked to this article. DATA AVAILABILITY All data are available at NERC EDS UK Polar Data Centre.
https://doi.org/10.5285/49047818-D36A-45CE-8AD1-3D459E1CCEAD and presented in the Supplementary material. REFERENCES * Le Quéré, C. et al. Trends in the sources and sinks of carbon dioxide.
_Nat. Geosci._ 2, 831–836 (2009). Article Google Scholar * Cavan, E. L. & Hill, S. L. Commercial fishery disturbance of the global ocean biological carbon sink. _Glob. Chang. Biol._
28, 1212–1221 (2022). Article PubMed Google Scholar * Kavanagh, L. & Galbraith, E. Links between fish abundance and ocean biogeochemistry as recorded in marine sediments. _Plos One_
13, e0199420 (2018). Article PubMed PubMed Central Google Scholar * Bianchi, D., Carozza, D. A., Galbraith, E. D., Guiet, J. & DeVries, T. Estimating global biomass and
biogeochemical cycling of marine fish with and without fishing. _Sci. Adv._ 7, eabd7554 (2021). Article PubMed PubMed Central Google Scholar * Saba, G. K. et al. Toward a better
understanding of fish-based contribution to ocean carbon flux. _Limnol. Oceanogr._ 66, 1639–1664 (2021). Article CAS Google Scholar * Sarmiento, J. et al. Simulated response of the ocean
carbon cycle to anthropogenic climate warming. _Nature_ 393, 245–249 (1998). Article CAS Google Scholar * Gruber, N., Landschützer, P. & Lovenduski, N. S. The variable Southern Ocean
carbon sink. _Annu. Rev. Mar. Sci._ 11, 159–186 (2019). Article Google Scholar * Hauck, J. et al. The Southern Ocean carbon cycle 1985–2018: mean, seasonal cycle, trends, and storage.
_Glob. Biogeochem. Cycles_ 37, e2023GB007848 (2023). Article CAS Google Scholar * Manno, C., Stowasser, G., Enderlein, P., Fielding, S. & Tarling, A. G. The contribution of
zooplankton faecal pellets to deep-carbon transport in the Scotia Sea (Southern Ocean). _Biogeosci. Discuss._ 11, 16105–16134 (2015). Google Scholar * Manno, C. et al. Continuous moulting
by Antarctic krill drives major pulses of carbon export in the north Scotia Sea, Southern Ocean. _Nat. Commun._ 11, 6051 (2020). Article CAS PubMed PubMed Central Google Scholar *
Belcher, A. et al. The potential role of Antarctic krill faecal pellets in efficient carbon export at the marginal ice zone of the South Orkney Islands in spring. _Polar Biol._ 40, 2001–2013
(2017). Article CAS PubMed PubMed Central Google Scholar * Belcher, A. et al. Krill faecal pellets drive hidden pulses of particulate organic carbon in the marginal ice zone. _Nat.
Commun._ 10, 889 (2019). Article CAS PubMed PubMed Central Google Scholar * Staresinic, N., Farrington, J., Gagosian, R. B., Clifford, C. H. & Hulburt, E. M. Downward transport of
particulate matter in the Peru coastal upwelling: Role of the anchoveta, _Engraulis ringens_. In: Suess, E. & Theide, J. (Eds.) Coastal Upwelling: Its Sediment Record. Part A. Responses
of the Sedimentary Regime to Present Coastal Upwelling. 225–240 (Plenum, 1983). * Saba, G. & Steinberg, D. Abundance, composition and sinking rates of fish faecal pellets in the Santa
Barbara Channel. _Sci. Rep._ 2, 716 (2012). Article PubMed PubMed Central Google Scholar * Guglielmo, L., Granata, A. & Greco, S. Distribution and abundance of post larval and
juvenile _Pleuragramma antarcticum_ (Pisces, Nototheniidae) off Terra Nova Bay (Ross Sea, Antarctica). _Polar Biol._ 19, 37–51 (1998). Article Google Scholar * Sutton, C. P. & Horn, P.
L. A preliminary assessment of age and growth of Antarctic silverfish. _CCAMLR Sci._ 18, 75–86 (2011). Google Scholar * La Mesa, M. & Eastman, J. T. Antarctic silverfish: life
strategies of a key species in the high-Antarctic ecosystem. _Fish. Fish._ 13, 241–266 (2012). Article Google Scholar * Vacchi, M. et al. A nursery area for the Antarctic silverfish
_Pleuragramma antarcticum_ at Terra Nova Bay (Ross Sea): first estimate of distribution and abundance of eggs and larvae under the seasonal sea-ice. _Polar Biol._ 35, 1573–1585 (2012a).
Article Google Scholar * Pinkerton, M. H. Diet and trophic ecology of adult Antarctic silverfish (_Pleuragramma antarctica_). In: Vacchi, M., Pisano, E., Ghigliotti, L. (eds) The Antarctic
silverfish: a keystone species in a changing ecosystem. 93–111 (Springer, 2017). * Vacchi, M., Koubbi, P., Ghigliotti, L. & Pisano, E. Sea-ice interactions with polar fish: focus on the
Antarctic silverfish life history. In: Adaptation and Evolution in Marine Environments, Vol 1: The Impacts of Global Change on Biodiversity, 51–73 (Springer Berlin Heidelberg, 2012b). *
Vacchi, M., Pisano, E. & Ghigliotti, L. The Antarctic Silverfish: a Keystone Species in a Changing Ecosystem (Springer International Publishing, 2017). * Carlig, E. et al. Diversified
feeding strategies of _Pleuragramma antarctica_ (Nototheniidae) in the Southern Ocean. _Polar Biol._ 42, 2045–2054 (2019). Article Google Scholar * Schaafsma, F. L. et al. Review: the
energetic value of zooplankton and nekton species of the Southern Ocean. _Mar. Biol._ 165, 129 (2018). Article PubMed PubMed Central Google Scholar * Ainley, D. G. & Siniff, D. B.
The importance of Antarctic toothfish as prey of Weddell seals in the Ross Sea. _Antarct. Sci._ 21, 317–327 (2009). Article Google Scholar * Vacchi, M., La Mesa, M., Dalù, M. &
MacDonald, J. Early life stages in the life cycle of Antarctic silverfish, _Pleuragramma antarcticum_ in Terra Nova Bay, Ross Sea. _Antarct. Sci._ 16, 299–305 (2004). Article Google Scholar
* Gow A. J., Ackley S. F. & Govoni J. W. Physical and structural properties of land-fast ice in McMurdo Sound, Antarctica. In: Jeffries, M. O. (ed) Antarctic Sea Ice: Physical
Processes, Interactions and Variability, Antarctic Research Series, 355–374 (American Geophysical Union, 1998). * Leonard, G. et al. Observations of platelet ice growth and oceanographic
conditions during the winter of 2003 in McMurdo sound, Antarctica. _J. Geophys. Res._ 111, C04012 (2006). Google Scholar * Smith, I. et al. Platelet ice and the land-fast sea ice of McMurdo
sound, Antarctica. _Ann. Glaciol._ 33, 21–27 (2001). Article Google Scholar * Hoppmann, M. et al. Platelet ice, the Southern Ocean’s hidden ice: a review. _Ann. Glaciol._ 61, 341–368
(2020). Article Google Scholar * Mangoni, O. et al. The role of platelet ice microalgae in seeding phytoplankton blooms in Terra Nova Bay (Ross Sea, Antarctica): a mesocosm experiment.
_Polar Biol._ 32, 311–323 (2009). Article Google Scholar * Granata, A. et al. Diversity in zooplankton and sympagic biota during a period of rapid sea ice change in Terra Nova Bay, Ross
Sea, Antarctica. _Diversity_ 14, 425 (2022). Article Google Scholar * Schlitzer, R. Carbon export fluxes in the Southern Ocean: results from inverse modeling and comparison with
satellite-based estimates. _Deep-Sea Res. II_ 49, 1623–1644 (2002). CAS Google Scholar * Collier, R. et al. The vertical flux of biogenic and lithogenic material in the Ross Sea: moored
sediment trap observations 1996–1998. _Deep-Sea Res. II_ 47, 3491–3520 (2000). CAS Google Scholar * Accornero, A., Manno, C., Arrigo, K., Martini, A. & Tucci, S. The vertical flux of
particulate matter in the polynya of Terra Nova Bay. Part I. Chemical constituents. _Antarct. Sci._ 15, 119–132 (2003). Article Google Scholar * Langone, L., Frignani, M., Cochran, J. K.
& Ravaioli, M. Scavenging processes and export fluxes close to a retreating seasonal ice margin (Ross Sea, Antarctica). _Water Air Soil Pollut._ 99, 705–715 (1997). Article CAS Google
Scholar * La Mesa, M., Riginella, E., Mazzoldi, C. & Ashford, J. Reproductive resilience of ice-dependent Antarctic silverfish in a rapidly changing system along the Western Antarctic
Peninsula. _Mar. Ecol._ 36, 235–245 (2015). Article Google Scholar * Cziko, P. A., Evans, C. W., Cheng, C. C. & DeVries, A. L. Freezing resistance of antifreeze-deficient larval
Antarctic fish. _J. Exp. Biol._ 209, 407–420 (2006). Article CAS PubMed Google Scholar * Craik, J. & Harvey, S. The causes of buoyancy in eggs of marine teleosts. _J. Mar. Biol.
Assoc. U. Kingd._ 67, 169–182 (1987). Article Google Scholar * Solemdal, P. et al. A comparison of egg quality and larval viability between cultured coastal cod and wild Arcto-Norwegian
cod. _Comm. Meet. int. Coun. Explor. Sea_ 41, 1–13 (1991). C.M.-ICES/F. Google Scholar * Nissling, A., Kryvi, H. & Vallin, L. Variation in egg buoyancy of Baltic cod _Gadus morhua_ and
its implications for egg survival in prevailing conditions in the Baltic Sea. _Mar. Ecol. Prog. Ser._ 110, 67–74 (1994). Article Google Scholar * Jørgensen, L. Carbon and nitrogen
utilization in developing eggs and larvae of cod (_Gadus morhua_, L.) and variation among different parents. _Fish. Res._ 3, 337–342 (1985). Article Google Scholar * Kock, K.-H. &
Kellermann, A. Reproduction in Antarctic notothenioid fish—a review. _Antarct. Sci._ 3, 125–150 (1991). Article Google Scholar * Corso, A. D., Steinberg, D. K., Stammerjohn, S. E. &
Hilton, E. J. Climate drives long-term change in Antarctic Silverfish along the western Antarctic Peninsula. _Commun. Biol._ 5, 104 (2022). Article PubMed PubMed Central Google Scholar *
Fuiman, L. A., Davis, R. W. & Williams, T. M. Behavior of midwater fishes under Antarctic ice: observations by a predator. _Mar. Biol._ 140, 815–822 (2002). Article Google Scholar *
La Mesa, M., Piepenburg, D., Pineda-Metz, Riginella, E. & Eastaman, J. T. Spatial distribution and habitat preferences of demersal fish assemblages in the southeastern Weddell Sea
(Southern Ocean). _Polar Biol._ 42, 1025–1040 (2019). Article Google Scholar * Evans, C. W., Williams, D. E., Vacchi, M., Brimble, M. A. & DeVries, A.-L. Metabolic and behavioural
adaptations during early development of the Antarctic silverfish, _Pleuragramma antarcticum_. _Polar Biol._ 35, 891–898 (2012). Article Google Scholar * Moline, M. A., Claustre, H.,
Frazer, T. K., Schofield, O. & Vernet, M. Alteration of the food web along the Antarctic Peninsula in response to a regional warming trend. _Glob. Change Biol._ 10, 1973–1980 (2004).
Article Google Scholar * Nitsche, F. & Davey, F. Ross Sea bathymetry grid (2005) based on Fred Davey’s bathymetry map (2004). Interdisciplinary Earth Data Alliance (IEDA).
https://doi.org/10.1594/IEDA/100405 (2013). * Cassano, J. J. et al. Wintertime observations of an Antarctic polynya with unmanned aircraft systems. _Eos_ 91, 245–246 (2010). Article Google
Scholar * Mohrmann, M., Heuze, C. & Swart, S. Southern Ocean polynyas in CMIP6 models. _Cryosphere_ 15, 4281–4313 (2021). Article Google Scholar * Drucker, R., Martin, S., &
Moritz, R. Observations of ice thickness and frazil ice in the St. Lawrence Island polynya from satellite imagery, upward looking sonar, and salinity/temperature moorings. _J. Geophys. Res_.
108. https://doi.org/10.1029/2001JC001213 (2003). * Bromwich, D. H. & Kurtz, D. D. Experiences of Scott’s Northern Party: evidence for a relationship between winter katabatic winds and
the Terra Nova Bay polynya. _Polar Rec._ 21, 137–146 (1982). Article Google Scholar * Faranda, F. M., Guglielmo, L. & Ianora, A. The Italian Oceanographic Cruises in the Ross Sea
(1987–95): strategy, general considerations and description of the sampling sites. In: Ross Sea Ecology: Italiantartide Expeditions (1987–1995), 1–13 (Springer Berlin Heidelberg, 2000). *
Jacobs, S. S., Fairbanks, R. G. & Horibe, Y. Origin and evolution of water masses near the Antarctic continental margin: evidence from H 218 O/ H 216O ratios in seawater. _Antarct. Res.
Ser._ 43, 59–85 (1985). Article Google Scholar * Budillon, G., Castagno, P., Aliani, S., Spezie, G. & Padman, L. Thermohaline variability and Antarctic Bottom Water formation at the
Ross Sea shelf break. _Deep-Sea Res. I_ 58, 1002–1018 (2011). Article Google Scholar * Cappelletti, A., Picco, P. & Peluso, T. Upper ocean layer dynamics and response to atmospheric
forcing in the Terra Nova Bay polynya, Antarctica. _Antarct. Sci._ 3, 319–329 (2010). Article Google Scholar * Heussener, S., Ratti, C. & Carbonne, J. The PPS3 time-series sediment
trap and the trap sample processing techniques used during the ECOMARGE experiment. _Cont. Shelf Res._ 10, 943–958 (1990). Article Google Scholar * Iseki, K. & Kiymoto, Y. Distribution
and settling of Japanese anchovy (Engraulis494 japonicus eggs at the spawning ground off Changjiang River in the East China Sea. _Fish. Oceanogr._ 6, 205–2010,
https://doi.org/10.1046/j.1365-2419.1997.00040.x (1997). Article Google Scholar Download references ACKNOWLEDGEMENTS The study was performed in the framework of MORSea project is supported
by the Italian National Antarctic Research Programme (PNRA), grant number OSS-13 - PNRA14_00070. Thanks to CEFREM, University of Perpignan, France to provide the laboratory support for
sediment trap analyses. We gratefully acknowledge M. Capello (DIPTERIS, University of Genova, Italy) and the crew of the RV Italica, for the deployment/recovery of the mooring. CM discloses
support for publication of this work from UKRI-FLF project CUPIDO (MR/T020962/1). AUTHOR INFORMATION AUTHORS AND AFFILIATIONS * British Antarctic Survey, Natural Environment Research
Council, NERC, Cambridge, UK Clara Manno * National Research Council (CNR) of Italy, Institute for the study of the Anthropic impacts and the Sustainability of the marine environment (IAS),
Genoa, Italy Erica Carlig * Department of Life and Environmental Sciences, Marche Polytechnic University, Ancona, Italy Pier Paolo Falco * Department of Mathematics and Computer Sciences,
Physical Sciences and Earth Sciences (MIFT), University of Messina, Messina, Italy Pasquale Castagno * Department of Science and Technology, University of Naples “Parthenope”, Naples, Italy
Giorgio Budillon Authors * Clara Manno View author publications You can also search for this author inPubMed Google Scholar * Erica Carlig View author publications You can also search for
this author inPubMed Google Scholar * Pier Paolo Falco View author publications You can also search for this author inPubMed Google Scholar * Pasquale Castagno View author publications You
can also search for this author inPubMed Google Scholar * Giorgio Budillon View author publications You can also search for this author inPubMed Google Scholar CONTRIBUTIONS C.M.
conceptualised the project, analysed the samples, prepared the figures, and wrote the manuscript with the assistance of all authors. P.F. and P.C. carried out the fieldwork. GB carried out
the funding acquisition. E.C. provided overviews on fish ecology and data interpretation. CORRESPONDING AUTHOR Correspondence to Clara Manno. ETHICS DECLARATIONS COMPETING INTERESTS The
authors declare no competing interests. PEER REVIEW PEER REVIEW INFORMATION _Communications Biology_ thanks Mario la Mesa and the other, anonymous, reviewer(s) for their contribution to the
peer review of this work. Primary Handling Editors: Quan-Xing Liu and Luke R. Grinham. ADDITIONAL INFORMATION PUBLISHER’S NOTE Springer Nature remains neutral with regard to jurisdictional
claims in published maps and institutional affiliations. SUPPLEMENTARY INFORMATION SUPPLEMENTARY INFORMATION REPORTING SUMMARY RIGHTS AND PERMISSIONS OPEN ACCESS This article is licensed
under a Creative Commons Attribution 4.0 International License, which permits use, sharing, adaptation, distribution and reproduction in any medium or format, as long as you give appropriate
credit to the original author(s) and the source, provide a link to the Creative Commons licence, and indicate if changes were made. The images or other third party material in this article
are included in the article’s Creative Commons licence, unless indicated otherwise in a credit line to the material. If material is not included in the article’s Creative Commons licence and
your intended use is not permitted by statutory regulation or exceeds the permitted use, you will need to obtain permission directly from the copyright holder. To view a copy of this
licence, visit http://creativecommons.org/licenses/by/4.0/. Reprints and permissions ABOUT THIS ARTICLE CITE THIS ARTICLE Manno, C., Carlig, E., Falco, P.P. _et al._ Life strategy of
Antarctic silverfish promote large carbon export in Terra Nova Bay, Ross Sea. _Commun Biol_ 7, 450 (2024). https://doi.org/10.1038/s42003-024-06122-8 Download citation * Received: 03 October
2023 * Accepted: 28 March 2024 * Published: 11 April 2024 * DOI: https://doi.org/10.1038/s42003-024-06122-8 SHARE THIS ARTICLE Anyone you share the following link with will be able to read
this content: Get shareable link Sorry, a shareable link is not currently available for this article. Copy to clipboard Provided by the Springer Nature SharedIt content-sharing initiative