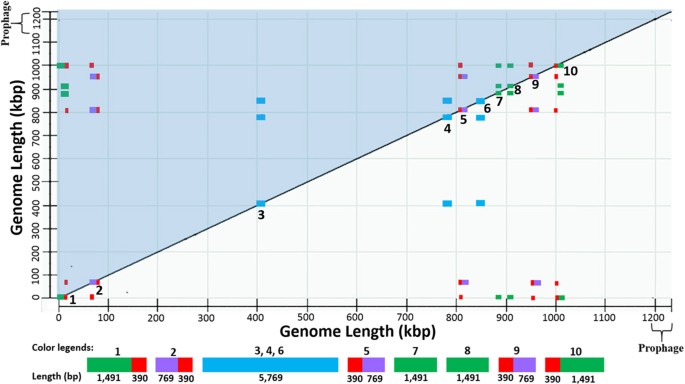
Unusual five copies and dual forms of nrdb in “candidatus liberibacter asiaticus”: biological implications and pcr detection application
- Select a language for the TTS:
- UK English Female
- UK English Male
- US English Female
- US English Male
- Australian Female
- Australian Male
- Language selected: (auto detect) - EN
Play all audios:

ABSTRACT “_Candidatus_ Liberibacter asiaticus” (CLas), a non-culturable α-proteobacterium, is associated with citrus Huanglongbing (HLB, yellow shoot disease) currently threatening citrus
production worldwide. Here, the whole genome sequence of CLas strain A4 from Guangdong of China was analyzed. Five copies of _nrdB_, encoding β-subunit of ribonucleotide reductase (RNR), a
critical enzyme involving bacterial proliferation, were found. Three _nrdB_ copies were in long form (_nrdB__L_, 1,059 bp) and two were in short form (_nrdB__S_, 378 bp). _nrdB__S_ shared
>99% identity to 3′ end of _nrdB__L_ and had no active site. Sequences of CLas _nrdB_ genes formed a distinct monophyletic lineage among eubacteria. To make use of the high copy number
feature, a _nrdB_-based primer set RNRf/RNRr was designed and evaluated using real-time PCR with 262 HLB samples collected from China and USA. Compared to the current standard primer set
HLBas/HLBr derived from the 16S rRNA gene, RNRf/RNRr had Ct value reductions of 1.68 (SYBR Green PCR) and 1.77 (TaqMan PCR), thus increasing the detection sensitivity three-fold. Meanwhile,
RNRf/RNRr was more than twice the stability of primer set LJ900f/LJ900r derived from multi-copy prophage. The _nrdB_-based PCR thereby provides a sensitive and reliable CLas detection with
broad application, especially for the early diagnosis of HLB. SIMILAR CONTENT BEING VIEWED BY OTHERS WHOLE GENOME SEQUENCES OF 135 “_CANDIDATUS_ LIBERIBACTER ASIATICUS” STRAINS FROM CHINA
Article Open access 19 September 2024 LOOP-MEDIATED ISOTHERMAL AMPLIFICATION (LAMP) ASSAY FOR SPECIFIC AND RAPID DETECTION OF _DICKEYA FANGZHONGDAI_ TARGETING A UNIQUE GENOMIC REGION Article
Open access 10 November 2022 SCAFFOLD-LEVEL GENOME ASSEMBLY OF _FUSARIUM NEOCOSMOSPORIELLUM_ STRAIN CA18-1 Article Open access 08 May 2025 INTRODUCTION “_Candidatus_ Liberibacter asiaticus”
(CLas), a phloem-limited α-proteobacterium, is associated with citrus Huanglongbing (HLB, yellow shoot disease, also known as citrus greening disease) that is devastating citrus production
worldwide1,2. No effective cure for HLB is currently available. Management of HLB depends on excluding CLas from citrus-producing regions though use of regional quarantines, pathogen-free
nursery stocks, removal of infected trees, and control of vectors, e.g. the Asian citrus psyllid (ACP, _Diaphorina citri_). Knowledge about CLas biology plays critical roles for development
of novel, effective HLB control strategies. Yet, study of this bacterium has been difficult due to the inability to culture it _in vitro_. Recent developments in bacterial whole genome
sequencing through next generation sequence (NGS) technology have opened a new venue for research in non-culturable plant pathogenic bacteria. We recently sequenced the whole genome of CLas
strain A4 from Guangdong, China where HLB was first described3,4. Analyses of genome sequence of the A4 strain has led to discovery of a CRISPR/cas system and dominant single prophage
phenomenon in CLas strains in China5. We also observed several large (>300 bp) DNA duplications in the strain A4 chromosome. One of them was identified as ribonucleotide reductase (RNR)
β-subunit gene, _nrdB_. RNR is a key enzyme for converting ribonucleotides to deoxyribonucleotides, the precursors of DNA synthesis and repair, which is under strict regulation during cell
proliferation6,7,8. RNR is also an important target for development of antibacterial drugs8. There have been extensive studies on RNR and its genes in model bacteria6,7,8. A database
dedicated for RNR research has been established9. Currently, no information about CLas RNR has been published, except for a brief mention of a partial RNR gene sequence in PCR detection10.
Detection of CLas mainly relies on PCR technologies involving the use of specifically designed primer sets based genomic DNA sequences, mostly the 16S rRNA gene. Examples are primer set
OI1/OI2c for standard PCR11 and primer set HLBas/HLBp/HLBr for TaqMan real-time PCR12. The chromosome of CLas has three copies of the 16S rRNA gene13. One strategy for further improvement of
PCR detection is to identify and target genes with >3 copies. The proof of concept has recently been achieved in PCR detection of _Spiroplasma citri_, causing citrus stubborn disease by
targeting multi-copy phage genes14. In CLas, a phage-based primer set (LJ900f/LJ900r) has been developed and tested15. However, recent investigation showed that CLas prophages and their
sequences were highly variable including the absence of prophage5,16, which could impede detection reliability or accuracy. The high copy number _nrdB_ provides an ideal target for sensitive
detection of CLas. The aims of this research were: (1) characterize _nrdB_ in CLas based on available RNR information and bacterial genome sequences and predict its possible biological
role; (2) elucidate phylogenetic relationships of CLas among eubacteria based on _nrdB_ DNA and amino acid sequences; and (3) evaluate the use of a _nrdB_-based primer set for improvement of
CLas detection, with comparisons made to existing PCR primers such as the 16S rRNA gene-based primer set HLBas/HLBr and the prophage sequence-based primer set LJ900f/LJ900r. RESULTS
IDENTIFICATION OF MULTIPLE-COPY REGIONS IN A4 GENOME As shown in Fig. 1, ten repeat regions were detected in the A4 genome by Dot Matrix analysis. Examination of the retrieved sequences
revealed that regions 3, 4 and 6 were identical DNA sequences of 5,769 bp, each containing the genes of 16S, 23S and 5S rRNAs or the _rrn_ operon (Supplementary Table S1). The other seven
regions were sequences of three different sizes: 1,881 bp for region 1 and 10, 1,059 bp for regions 2, 5, and 9, and 1,491 bp for regions 7 and 8. Results of sequence alignments showed that
regions 1, 2, 5, 9, and 10 contained a common 390-bp sequence (red in Fig. 1); regions 1, 7, 8, and 10 contained a common 1,492 bp sequence (green in Fig. 1); and regions 2, 5, and 9
contained a common 769 bp sequence (purple in Fig. 1). Genes or open reading frames (ORFs) corresponding to each region were listed in Supplementary Table S1. CHARACTERIZATION OF CLAS _NRDB_
Since the 390 bp sequence was repeated five times (the highest) in the CLas genome, the 390 bp-containing sequences, i.e. region 1, 2, 5, 9, 10 (Fig. 1) were selected for further study. In
region 1 and 10, 378 of the 390 bp formed ORFs CD16_00035 and CD16_04445, respectively (Supplementary Table S1). In region 2, 5, and 9, the whole 1,059 bp formed ORFs CD16_00300, CD16_03625,
and CD16_04230, respectively (Supplementary Table S1). All five sequences were annotated as _nrdB_ encoding the β-subunit of RNR Class Ia (EC 1.17.4.1), two (CD16_00035 and CD16_04445) in
short form (_nrdB__S_, 125 amino acids) and three (CD16_00300, CD16_03625, and CD16_04230) in long forms (_nrdB__L_, 352 amino acids) (Table 1). Note that 12 bp at the 5′ end of the 390-bp
sequence were not part of _nrdB__S_ (Fig. 2). _nrdB__S1_and _nrdB__S2_ had a SNP at position 389, part of the synonymous stop codons. Five SNPs were found among _nrdB__L1_, _nrdB__L2_, and
_nrdB__L3_ without causing frame shifts (Fig. 2). Conserved domain analysis indicated the long nrdBL protein (352-aa) contained a diiron center (ion binding site), the tyrosyl radical, a
putative radical transfer pathway and a dimer interface (polypeptide binding site) (Fig. 3). No iron binding site was identified on the short nrdBS protein (125-aa) as shown in the predicted
3-D structures (Fig. 3). BLASTn search against all published CLas genome sequences revealed that all CLas strains had the same number of nearly identical _nrdB_ genes (both _nrdB__S_ and
_nrdB__L_) (Table 2), except for the CLas strain SGCA5, which could be due to the influence of _de novo_ assembly17 that dropped out repeat sequences because reassembly using A4 sequence as
a reference showed the same five _nrdB_ genes (unpublished data). The copy number of _nrdB_ in CLas was much higher (five) than all the non-CLas Liberibacters, as well as those of other
bacterial species (Table 2). Phylogenetic trees of selected representative bacteria based on 16S rRNA gene, amino acid sequence and DNA sequence of _nrdB_ gene are shown in Fig. 4. In all
three trees, Liberibacters were clustered together. Within Liberibacters, CLas clustered together, demonstrating the monophyletic lineage of CLas based on _nrdB_ gene as that of the 16S rRNA
gene. It is, however, noted that based on 16S rRNA gene tree, _Agrobacterium_ was closely related to Liberibacters. This was not the case in the _nrdB_ gene tree. SPECIFICITY OF RNR PRIMER
SET Primer set RNRf/RNRr was designed based on the 390 bp repeats in the CLas genome (Fig. 2; Table 3). BLASTn search (word size = 16) using RNRf/RNRr primer sequences as queries against the
GenBank nr/nt database that contained >1,000 bacterial genome sequences returned hits strictly to the RNR gene of CLas. PCR of DNA samples extracted from two healthy citrus plants and
one CLas-free psyllid reared in our laboratory showed no amplification with primer set RNRf/RNRr by SYBR Green real-time PCR. The melting point of RNRf/RNRr amplicon was at 81.50 °C.
EVALUATIONS AMONG RNRF/RNRR, HLBAS/HLBR, AND LJ900F/LJ900R A total of 57 CLas samples collected from China and USA were selected for primer set evaluations (Fig. 5). Sensitivity comparisons
were performed simultaneously by SYBR Green real-time PCR format (all three primer sets) and TaqMan real-time PCR (RNRf/RNRr and HLBas/HLBr). As shown in Fig. 5, mean Ct values were 20.05
for RNRf/RNRr, 21.71 for HLBas/HLBr, and 23.33 for LJ900f/LJ900r. Standard deviations from RNRf/RNRr (2.22) and HLBas/HLBr (2.37) were smaller than that from LJ900f/LJ900r (4.91), suggesting
higher sequence variations of CLas prophages than those of the conserved 16S rRNA gene and _nrdB_. Mean Ct differences between RNRf/RNRr and HLBas/HLBr were significant P < 0.001 in both
SYBR Green PCR and TaqMan PCR formats, with ΔCt being −1.68 ± 0.18 for SYBR green PCR and −1.77 ± 0.18 for TaqMan PCR. These represent >3 fold increase of sensitivity based on the ΔCt
method18. Differences between RNRf/RNRr and LJ900f/LJ900r and between HLBas/HLBr and LJ900f/LJ900r were also significant at P < 0.05 level. EVALUATION ON RNRF/RNRR WITH FIELD SAMPLES FROM
CHINA AND USA A total of 262 DNA samples extracted from CLas infected plants and psyllids in seven provinces in China and three states in USA were tested with SYBR Green real-time PCR
format (Table 4). Overall, there was a significant difference between the Ct values of RNRf/RNRr and HLBas/HLBr (P < 0.0001), although variations existed from location to location in both
countries. The largest P value in China was from Guangxi Province and the largest P value in USA was from Florida. However, in all cases, P values were <0.05 and ΔCt were negative within
a range from −1.36 to −1.75 (Table 4). In addition, the RNRf/RNRr qPCR assays on three different qPCR systems (ABI system, MJ system, and CFX system) also showed the robust of RNRf/RNRf on
detection of CLas (Table S2). DISCUSSION The inability to culture CLas _in vitro_ limits the use of traditional _in vitro_ culture-based methodologies to study its biology. Genome sequence
analyses in this study provided the first insight into an RNR gene of CLas and reveal previously unknown properties of the bacterium. According to model studies, RNRs are divided into three
classes (Classes I, II, and III), largely based on their interaction with oxygen and the way in which they generate their tyrosyl racdical19. The CLas _nrdB_ described in this study belongs
to Class Ia, that is exclusively oxygen-dependent8, implying an aerobic lifestyle of CLas. This is the first report on oxygen usage status of CLas, which will benefit future efforts on _in
vitro_ cultivation of the bacterium. Typically, bacterial RNR genes are arranged in an operon. Class Ia RNR genes form _nrdAB_, where _nrdA_ encodes RNR α-subunit, and _nrdB_ encodes RNR
β-subunit. This does not seem to be the case in CLas, where both _nrdA_ and _nrdB_ are dispersed separately in the bacterial genome (Table 1). Examinations of neighboring regions of each
_nrdB_ gene revealed no RNR gene homologs with the exception of _nrdB__L2_ (Supplementary Table S3). Upstream of _nrdB__L2_ is _RibF_ (Supplementary Table S3), encoding a riboflavin
biosynthesis protein similar to that of _nrdI_ in the Class Ib operon _nrdHIEF_ where _nrdH_ encodes a glutaredoxin-like protein, _nrdI_ encodes a flavorotein, _nrdE_ encodes RNR α-subunit,
and _nrdF_ encodes RNR β-subunit20. It was also reported that in _Mycobacterium tuberculosis,_ RNR subunit genes were not arranged in an operon21. Interestingly, both CLas and _M.
tuberculosis_ are nutritionally fastidious intracellular pathogens. The HLB associated CLas is not cultivable. The slow growing _M. tuberculosis_ causes human tuberculosis. The most
intriguing finding from this study is that CLas has five copies _nrdB_, three in a long form designated _nrdB__L_ and two in a short form designated _nrdB__S_, along with a single _nrdA_
(Table 1). As shown in Table 2, among the known Liberibacter genomes, only CLas has multiple copies of RNR genes. Although it is common to find multiple RNR classes within a single bacterial
species8, only a few cases of _nrd_ gene direct duplication have been reported. For example, _M. tuberculosis_ has a second class Ib-like subunit gene21 and _Sreptococcus pyogenes_ has two
clusters of class Ib genes, _nrdHEF_ and _nrdF*I*E*_22. In both cases, the duplicated genes show significant variations at the level of DNA sequences (<71% identity). In this study, the
sequences of three _nrdB__L_ are almost identical and the two _nrdB__S_ are nearly identical. The common regions between _nrdB__L_ and _nrdB__S_ are also identical. These indicate that the
_nrdB_ gene duplication events are recent. Duplication of RNR genes has been shown to be important for bacterial proliferation. As in the cases of _M. tuberculosis_ and _S. pyogenes_, the
two different _nrd_ genes allowed bacterial growth under different growth environments21,22. Along this direction, the _nrdB_ duplication in CLas could be related to its environmental
adaptation and likely by increasing functional dosage23. Although more evidence is needed, it will be of interest to study if this possible dosage effect could be linked to the current
dominance of CLas in HLB. In Brazil, both CLas and CLam were reported to be associated with HLB24. However, as observation continued, the population of CLas increased whereas the population
of CLam decreased25,26. It is noted that _nrdB__S_ has no active site (Fig. 4). Its biological role(s) could be an interesting topic. In early research, a strain of _Escherichi coli_ (C600)
was found to have two forms of β-subunit of RNR, one was a full length and functional β-polypeptide, the other was a truncated and non-functional β’-polypeptide27. In a model RNR structure
of α2β2, there could be two possible homodimeric β-subunits (ββ and β’β’) and one heterodimeric β-subunit (ββ’). The heterodimeric β-subunit was found to conform to a half-site reactivity,
which might be involved in regulation of enzyme activity. In this regard, we speculate that the non-functional short form _nrdB__S_ could be used at the transcriptional level to generate a
heterodimer as part of the RNR regulation in CLas proliferation. While _in silico_ genome sequence analyses of RNR genes only provide information for understanding CLas biology, the high
copy number and conserved feature of _nrdB_ was explored for CLas detection. The use of primer set HLBas/HLBr along with a hybridization probe (TaqMan PCR) has been regarded as a standard
protocol for CLas detection. However, problems arise when high Ct values, e.g. Ct = 30 or higher, are encountered. This situation is commonly encountered when testing citrus trees for the
presence of CLas, especially for symptomless or atypical symptom samples. The available RNRf/RNRr PCR detection system provides a remedy. First, as HLBas/HLBas, RNRf/RNRr was also based on
the highly conserved gene. This assured the reliability of CLas detection, in contrast to the prophage-based primer set Lj900f/LJ900r (Fig. 5). In fact, the universal presence of RNR gene
has been recommended as a key target for phylogeny research of viruses that lack ribosomal RNA genes28; and second, the RNRf/RNRr locus has five copies, higher than the three copies of the
16S rRNA gene. This means more initial targets are available for PCR leading to increased sensitivity of detection. As demonstrated in Fig. 5, RNRf/RNRr PCR is at least three times more
sensitive than HLBas/HLBr PCR in both SYBR green and TaqMan formats. In this study, the robust of RNRf/RNRrqPCR assays were also confirmed on three different real-time PCR system, although
greater sensitivity of RNR primers was showed on both ABI system and MJ system rather than on CFX system (Table S2). In summary, through genome sequence analyses, we discovered that CLas had
five copies of RNR β-subunit gene _nrdB_. CLas _nrdB_ has both long and short forms that could play a role in the RNR regulation in the bacterial proliferation. Phylogenetically, all CLas
_nrdB_ genes clustered together, forming a stable evolutionary lineage, as that of the 16S rRNA gene. The high copy number and conserved feature of _nrdB_ provide a foundation for being used
in sensitive and reliable detection of CLas. Primer set RNRf/RNRr has been developed and tested. The detection system is recommended for use to resolve CLas detection issue when the primer
set HLBas/HLBr encounters border line Ct for interpretation. MATERIALS AND METHODS BACTERIAL GENOME SEQUENCES AND STRAINS The whole genome sequence of CLas strain A4 that originated from an
HLB citrus tree in Guangdong of China (CP010804)3 was used for DNA/gene copy evaluation. All bacterial genome sequences were downloaded from GenBank database (v211.0) hosted by the National
Center for Biotechnology information (NCBI) (Table 2). Field strains were collected for population study. A CLas strain was represented by DNA extracted from an infected leaf sample of
citrus (_Citrus_ sp.) or periwinkle (_Catharanthus roseus_) or an individual ACP. Samples were from seven provinces (Guangdong, Guangxi, Yunnan, Fujian, Jiangxi, Zhejiang and Hainan) in
China and three states (Florida, Texas and California) in USA (Table 4). DNA were extracted by with E. Z. N. A. HP Plant DNA Kit (OMEGA Bio-Tek Co., Guangdong, China) or DNeasy Plant Kits
(Qiagen Inc., Valencia, CA) for plant samples, and TIANamp Genomic DNA Kit (Tiangen Biotech Co., Beijing, China) or DNeasy Blood & Tissue Kit (Qiagen Inc., Valencia, CA) for individual
psyllid. IDENTIFICATION OF _NRDB_ AND _IN SILICO_ CHARACTERIZATION The CLas strain A4 genome sequence was self-compared using the BLASTn program with the word size set at 128-bp with the web
service of NCBI. The result was visualized with the Dot-Matrix option. DNA sequence regions with highest number of repeats were retrieved. The genetic nature of DNA sequences was
characterized according to genome annotation, assisted by BLAST search against the NCBI conserved domain database (CDD, v3.14). Since the identified DNA sequences were longer than the
annotated genes, only gene sequences were downloaded and used for analyses. Protein structure analyses were initially carried out with Phyre server
(http://www.sbg.bio.ic.ac.uk/~phyre2/html/page.cgi?id=index) using a profile-profile alignment algorithm29. Final 3-D structures were made using Pymol Molecular Graphics System (v1.7.6,
Schrödinger LLC). For phylogenetic studies, all published CLas and selected bacterial species representing major bacterial groups were used (Table 2). DNA and amino acid sequence of _nrdB_
were retrieved according to genome annotation or from the ribonucleotide reductase database (v0.901)9. The total number of _nrdB_ gene in each genome was directly counted from the genome
annotation and further confirmed by similarity searching the bacterial genome with the corresponding _nrdB_ sequence. DNA sequences of 16S rRNA genes were downloaded from NCBI GenBank
nucleotide database (Genbank version 211.0). Phylogenetic trees were constructed using the Neighbor-joining method with MEGA 6.030. PRIMER/PROBE DESIGNS AND PCR EXPERIMENTS CLas nrdB
sequences were aligned through the Clustal Omega software31. Common regions across all nrdB sequences were identified and used to design PCR primers and TaqMan probe sequences with Primer 3
software32 (Table 3). Primer and probe sequence specificity were checked through BLASTn against the GenBank nucleotide database (Genbank version 211.0). The TaqMan probe was synthesized by
labeling the 5′-terminal nucleotide with 6-carboxy-fluorescein (FAM) reporter dye and the 3′-terminal nucleotide with Black Hole Quencher (BHQ)-1 (Table 3) through a commercial source.
Primers of HLBas/HLBr and HLBp and LJ900f/LJ900r were synthesized according to the original publication12,15. Both SYBR Green and TaqMan real-time PCR formats were used in this study. The
SYBR Green real-time PCR assays were performed in three different real-time PCR systems. In the USA, MJ Research DNA Engine opticon 2 system (MJ; MJ Research Inc), and Applied Biosystems
StepOnePlus™ Real-Time PCR Systems (ABI; Applied Biosystems, Foster City, CA, US) were used. In China, the CFX Connect Real-Time System (Bio-Rad, Hercules, CA, USA) was used. The TaqMan
real-time PCR assays were only performed in the Applied Biosystems StepOnePlus™ Real-Time PCR Systems. Real-time PCR procedures were essentially referenced to that of Li _et al_.12. For SYBR
Green real-time PCR, the reaction mixture contained 10 μl of iQ™ SYBR® Green Supermix (Bio-Rad) or Fast SYBR® Green Master Mix (Applied Biosystems) or Bestar® qPCR Master Mix (DBI®
Bioscience), 1 μl of DNA template (~25 ng), 0.5 μl of each forward and reverse primer (10 μM) in a final volume of 20 μl with the following procedure: 95 °C for 3 min (MJ and CFX) or 95 °C
for 20 s (ABI), followed by 40 cycles at 95 °C for 10 s (MJ) or 95 °C for 3 s (ABI) or 95 °C for 10 s (CFX, Bio-Rad) and 60 °C for 30 s (MJ and CFX) or 60 °C for 3 s (ABI). The fluorescence
signal was captured at the end of each 60 °C step, followed by a melting point analysis. For TaqMan® real-time PCR, the reaction mixture contained 10 μl of TaqMan® Fast Universal PCR Master
Mix (2X) (Applied Biosystems), 1 μl of DNA template (~25 ng), 0.2 μl of TaqMan® probe (5 μM), 0.4 μl of each forward and reverse primer (10 μM) in a final volume of 20 μl with the following
procedure: 50 °C for 2 min, then 95 °C for 20 s, followed by 40 cycles at 95 °C for 3 s and 60 °C for 30 s. The fluorescence signal was captured at the end of each 60 °C step. The data were
analyzed using Opticon Monitor™ software (MJ Research), StepOnePlus™ Software v2.3 (Applied Biosystems) and Bio-Rad CFX Manager 2.1 software with automated baseline settings and a manually
set threshold at 0.1. Amplicons were quantified using standard curves established based on ten-fold serial dilutions of the CLas-infected citrus plant total DNA in triplicate. For evaluation
of differences among primer sets of RNRf/RNRr, HLBas/HLBr, and LJ900f/LJ900r, 34 CLas samples from China and 10 CLas samples from USA were used (Table 3). The SYBR green real-time PCR
format was used to for primer set evaluations. Since HLBas/HLBr-HLBp (TaqMan real-time PCR format) was popularly used, RNRf/RNRr-RNRp was also used. To substantiate the evaluation results, a
total of 262 CLas samples collected from China and USA (Table 4) were tested with SYBR green format. STATISTICAL ANALYSIS PCR results (Ct values) among different primer sets were evaluated
by independent-sample T test. All tests were performed using the SPSS Statistic package (v19.0, IBM, Armonk, New York, U.S.). Sensitivity increase (R) between RNRf/RNRr and HLBas/HLBr was
calculated through the ΔCt method18, i.e. R = 2−ΔCt, ΔCt = Ct (RNRf/RNRr)–Ct (HLBas/HLBr). ADDITIONAL INFORMATION HOW TO CITE THIS ARTICLE: Zheng, Z. _et al_. Unusual Five Copies and Dual
Forms of _nrdB_ in “_Candidatus_ Liberibacter asiaticus”: Biological Implications and PCR Detection Application. _Sci. Rep._ 6, 39020; doi: 10.1038/srep39020 (2016). PUBLISHER’S NOTE:
Springer Nature remains neutral with regard to jurisdictional claims in published maps and institutional affiliations. REFERENCES * Bové, J. M. Huanglongbing: a destructive, newly-emerging,
century-old disease of citrus. J Plant Pathol. 88, 7–37 (2006). Google Scholar * Jagoueix, S., Bové, J. M. & Garnier, M. The phloem-limited bacterium of greening disease of citrus is a
member of the α subdivision of the Proteobacteria. Int J Syst Bacteriol. 44, 379–386 (1994). Article CAS PubMed Google Scholar * Zheng, Z., Deng, X. & Chen, J. Whole-genome sequence
of “_Candidatus_ Liberibacter asiaticus” from Guangdong, China. Genome Announc. 2, e00273–14 (2014). PubMed PubMed Central Google Scholar * Lin, K. H. Observations on yellow shoot of
citrus. Acta Phytopathol. Sin. 2, 1–11 (1956). Google Scholar * Zheng, Z., Bao, M., Wu, F., Chen, J. & Deng, X. Predominance of single prophage carrying a CRISPR/cas system in
“_Candidatus_ Liberibacter asiaticus” strains in southern China. PloS One. 11, e0146422 (2016). Article PubMed PubMed Central Google Scholar * Eklund, H., Uhlin, U., Färnegårdh, M.,
Logan, D. T. & Nordlund, P. Structure and function of the radical enzyme ribonucleotide reductase. Prog Biophys Mol Biol. 77, 177–268 (2001). Article CAS PubMed Google Scholar *
Kolberg, M., Strand, K. R., Graff, P. & Andersson, K. K. Structure, function, and mechanism of ribonucleotide reductases. Biochim Biophys Acta. 1699, 1–34 (2004). Article CAS PubMed
Google Scholar * Torrents, E. Ribonucleotide reductases: essential enzymes for bacterial life. Front Cell Infect Microbiol. 4, 52 (2014). Article PubMed PubMed Central Google Scholar *
Lundin, D., Torrents, E., Poole, A. M. & Sjöberg, B. M. RNRdb, a curated database of the universal enzyme family ribonucleotide reductase, reveals a high level of misannotation in
sequences deposited to Genbank. BMC Genomics 10, 589 (2009). Article PubMed PubMed Central Google Scholar * Razi, M. F. et al. Detection of citrus huanglongbing-associated ‘_Candidatus_
Liberibacter asiaticus’ in citrus and _Diaphorina citri_ in Pakistan, seasonal variability, and implications for disease management. Phytopathology 104, 257–68 (2014). Article CAS PubMed
Google Scholar * Jagoueix, S., Bové, J. M. & Garnier, M. PCR detection of the two ‘_Candidatus_’ Liberobacter species associated with greening disease of citrus. Mol Cell Probes 10,
43–50 (1996). Article CAS PubMed Google Scholar * Li, W. B., Hartung, J. S. & Levy, L. Quantitative real-time PCR for detection and identification of “_Candidatus_ Liberibacter”
species associated with citrus Huanglongbing. J. Microbiol. Meth. 66, 104–115 (2006). Article CAS Google Scholar * Duan, Y. et al. Complete genome sequence of citrus Huanglongbing
bacterium, ‘_Candidatus_ Liberibacter asiaticus’ obtained through metagenomics. Mol Plant-Microbe Interact. 22, 1011–1020 (2009). Article CAS PubMed Google Scholar * Wang, X.,
Doddapaneni, H., Chen, J. & Yokomi, R. K. Improved real-time PCR diagnosis of citrus stubborn disease by targeting prophage genes of _Spiroplasma citri_. Plant Disease 99, 149–154
(2014). Article Google Scholar * Morgan, J. K. et al. Improved real-time PCR detection of ‘_Candidatus_ Liberibacter asiaticus’ from citrus and psyllid hosts by targeting the intragenic
tandem-repeats of its prophage genes. Mol Cell Probes 26, 90–98 (2012). Article CAS PubMed Google Scholar * Katoh, H., Miyata, S., Inoue, H. & Iwanami, T. Unique features of a
Japanese ‘_Candidatus_ Liberibacter asiaticus’ strain revealed by whole Genome Sequencing. PloS One 9, e106109 (2014). Article ADS PubMed PubMed Central Google Scholar * Wu, F. et al.
Draft Genome Sequence of “_Candidatus_ Liberibacter asiaticus” from a citrus tree in San Gabriel, California. Genome Announc. 3, e01508–15 (2015). PubMed PubMed Central Google Scholar *
Wittwer, C. T., Herrmann, M. G., Gundry, C. N. & Elenitoba-Johnson, K. S. Real-time multiplex PCR assays. Methods 25, 430–442 (2001). Article CAS PubMed Google Scholar * Nordlund, P.
& Reichard, P. Ribonucleotide reductases. Annu Rev Biochem. 75, 681–706 (2006). Article CAS PubMed Google Scholar * Jordan, A., Aragall, E., Gibert, I. & Barbe, J. Promoter
identification and expression analysis of _Salmonella typhimurium_ and _Escherichia coli_ nrdEF operons encoding one of two class I ribonucleotide reductases present in both bacteria. Mol.
Microbiol. 19, 777–790 (1996). Article CAS PubMed Google Scholar * Yang, F. et al. Characterization of two genes encoding the _Mycobacterium tuberculosis_ ribonucleotide reductase small
subunit. J. Bacteriol. 179, 6408–6415 (1997). Article CAS PubMed PubMed Central Google Scholar * Roca, I., Torrents, E., Sahlin, M., Gibert, I. & Sjoberg, B. M. NrdI essentiality
for class Ib ribonucleotide reduction in _Streptococcus pyogenes_. J. Bacteriol. 190, 4849–4858 (2008). Article CAS PubMed PubMed Central Google Scholar * Anderson, R. P. & Roth, J.
R. Tandem genetic duplications in phage and bacteria. Annu Rev Microbiol. 31, 473–505 (1977). Article CAS PubMed Google Scholar * Teixeira, D. C. et al. ‘_Candidatus_ Liberibacter
americanus’, associated with citrus Huanglongbing (greening disease) in São Paulo State, Brazil. Int J Syst Evol Microbiol. 55, 1857–62 (2005). Article CAS PubMed Google Scholar * Lopes,
S. A. et al. Graft transmission efficiencies and multiplication of ‘_Candidatus_ Liberibacter americanus’ and ‘_Ca_. Liberibacter asiaticus’ in citrus plants. Phytopathology 99, 301–306
(2009). Article CAS PubMed Google Scholar * Lopes, S. A. et al. Liberibacters associated with citrus huanglongbing in Brazil: ‘_Candidatus_ Liberibacter asiaticus’ is heat tolerant,
‘_Ca_. L. americanus’ is heat sensitive. Plant Dis. 93, 257–262 (2009). Article CAS PubMed Google Scholar * Sjoberg, B. M., Karlsson, K. & Jornvall, H. Half-site Reactivity of the
tyrosyl radical of ribonucleotide reductase from _Escherichia coli_. J. Biological Chemistry. 262, 9736–9743 (1987). CAS Google Scholar * Dwivedi, B., Xue, B., Lundin, D., Edwards, R. A.
& Breitbart, M. A bioinformatic analysis of ribonucleotide reductase genes in phage genomes and metagenomes. BMC Evol Biol. 13, 33 (2013). Article CAS PubMed PubMed Central Google
Scholar * Kelley, L. A., Mezulis, S., Yates, C. M., Wass, M. N. & Sternberg, M. J. The Phyre2 web portal for protein modeling, prediction and analysis. Nat Protoc. 10, 845–58 (2015).
Article CAS PubMed PubMed Central Google Scholar * Tamura, K., Stecher, G., Peterson, D., Filipski, A. & Kumar, S. MEGA6: Molecular evolutionary genetics analysis version 6.0.
Molecular Biology and Evolution. 30, 2725–2729 (2013). Article CAS PubMed PubMed Central Google Scholar * Sievers, F. et al. Fast, scalable generation of high-quality protein multiple
sequence alignments using Clustal Omega. Molecular Systems Biology 7, 539 (2011). Article PubMed PubMed Central Google Scholar * Untergrasser, A. et al. Primer3 - new capabilities and
interfaces. Nucleic Acids Research 40, e115 (2012). Article Google Scholar Download references ACKNOWLEDGEMENTS We thank C. Wallis and C. Ledbetter for critical editing of this manuscript
and X. Sun and L. Kumagai for DNA samples. This study was funded by Chinese Modern Agricultural Technology Systems (CARS-27), the Special Fund for Three-high Agriculture in Guangdong
Province, China (F15070), and California Citrus Research Board. Mention of trade names or commercial products in this publication is solely for the purpose of providing specific information
and does not imply recommendation or endorsement by the U.S. Department of Agriculture. USDA is an equal opportunity provider and employer. AUTHOR INFORMATION AUTHORS AND AFFILIATIONS *
Guangdong Province Key Laboratory of Microbial Signals and Disease Control, Citrus Huanglongbing Research Laboratory, South China Agricultural University, Guangzhou, The People's
Republic of China Zheng Zheng, Meirong Xu, Minli Bao, Fengnian Wu & Xiaoling Deng * United States Department of Agriculture–Agricultural Research Service, San Joaquin Valley Agricultural
Sciences Center, Parlier, California, United States of America Zheng Zheng, Fengnian Wu & Jianchi Chen Authors * Zheng Zheng View author publications You can also search for this author
inPubMed Google Scholar * Meirong Xu View author publications You can also search for this author inPubMed Google Scholar * Minli Bao View author publications You can also search for this
author inPubMed Google Scholar * Fengnian Wu View author publications You can also search for this author inPubMed Google Scholar * Jianchi Chen View author publications You can also search
for this author inPubMed Google Scholar * Xiaoling Deng View author publications You can also search for this author inPubMed Google Scholar CONTRIBUTIONS Z.Z., J.C. and X.D. were
responsible for the conception and design of the study; Z.Z., M.X., M.B. and F.W. performed the experiments. Z.Z., M.X., M.B., F.W., J.C. and X.D. analyzed the data. Z.Z., J.C. and X.D.
drafted the paper. All authors reviewed the manuscript. ETHICS DECLARATIONS COMPETING INTERESTS The authors declare no competing financial interests. ELECTRONIC SUPPLEMENTARY MATERIAL
SUPPLEMENTARY INFORMATION RIGHTS AND PERMISSIONS This work is licensed under a Creative Commons Attribution 4.0 International License. The images or other third party material in this
article are included in the article’s Creative Commons license, unless indicated otherwise in the credit line; if the material is not included under the Creative Commons license, users will
need to obtain permission from the license holder to reproduce the material. To view a copy of this license, visit http://creativecommons.org/licenses/by/4.0/ Reprints and permissions ABOUT
THIS ARTICLE CITE THIS ARTICLE Zheng, Z., Xu, M., Bao, M. _et al._ Unusual Five Copies and Dual Forms of _nrdB_ in “_Candidatus_ Liberibacter asiaticus”: Biological Implications and PCR
Detection Application. _Sci Rep_ 6, 39020 (2016). https://doi.org/10.1038/srep39020 Download citation * Received: 04 July 2016 * Accepted: 16 November 2016 * Published: 13 December 2016 *
DOI: https://doi.org/10.1038/srep39020 SHARE THIS ARTICLE Anyone you share the following link with will be able to read this content: Get shareable link Sorry, a shareable link is not
currently available for this article. Copy to clipboard Provided by the Springer Nature SharedIt content-sharing initiative