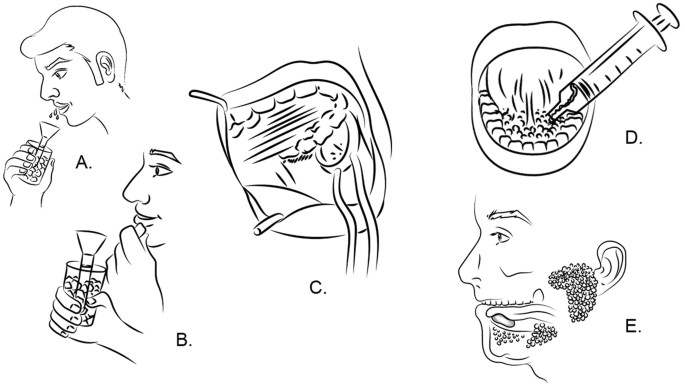
The proteomic profile of whole and glandular saliva in healthy pain-free subjects
- Select a language for the TTS:
- UK English Female
- UK English Male
- US English Female
- US English Male
- Australian Female
- Australian Male
- Language selected: (auto detect) - EN
Play all audios:

ABSTRACT Determination of the variability in the salivary proteome is a prerequisite for the development of saliva as a diagnostic and prognostic tool in particular physiological states. In
this context, it is important that technical variability induced by sample collection and processing is kept at minimum to be able to reproducibly assess variability in states of health and
disease. In the current study, the proteome profile in unstimulated and stimulated whole, parotid and sublingual saliva was investigated using two-dimensional gel electrophoresis. Saliva
samples were structurally collected from ten examined and characterized healthy individuals during the exactly same conditions. The results demonstrated that different collection methods
provide clear differences in the snapshot of the salivary proteome and also in the relative amount of specific proteins. The variable nature of the salivary proteome suggests that different
approaches may have to be adopted when studying its composition or its possible role as an indicator for particular physiological states. The results emphasize the importance of consistency
when collecting saliva samples for proteomic analysis. SIMILAR CONTENT BEING VIEWED BY OTHERS SALIVARY BIOMARKERS: NOVEL NONINVASIVE TOOLS TO DIAGNOSE CHRONIC INFLAMMATION Article Open
access 29 June 2023 SALIVARY PROTEOME OF APHTHOUS STOMATITIS REVEALS THE PARTICIPATION OF VITAMIN METABOLISM, NUTRIENTS, AND BACTERIA Article Open access 02 August 2021 IDENTIFICATION OF
EARLY BIOMARKERS IN SALIVA IN GENETICALLY ENGINEERED MOUSE MODEL C(3)1-TAG OF BREAST CANCER Article Open access 07 July 2022 INTRODUCTION Human saliva contains numerous proteins and
peptides, each of them carries several significant biological functions. These proteins are not only important in maintaining the health of the oral cavity but also may yield information
about both local and systemic disease1. Functions of the saliva are not only restricted to processing food for digestion. Saliva contains a large number of proteins, which play important
roles in the regulation of the immune defence, endocrine system and maintenance of mucosal tissue and dental health2,3. The salivary glands are also integrated into the neuroendocrine system
through complex regulatory pathways4. Some of the peptides found in saliva are also present in spinal fluid and microdialysate, where they have been implicated in a proposed neurochemical
pathogenesis of pain in rheumatoid arthritis5, temporomandibular disorders (TMD)6,7, trapezius myalgia and fibromyalgia syndrome8,9. Ultimately, saliva may contain locally expressed proteins
and other substances that can be used as indicators of diseases4,10. These components, called biomarkers, can be closely related to an individual’s health condition and can change greatly
when diseases afflict. Using saliva for biomarker detection and prognosis is not only practical and non-invasive, but it could also provide a more accurate fluid than blood10. Unlike blood
it does not clot and is easier to handle, also saliva contains a smaller quantity of proteins, therefore decreasing any potential risk of non-specific interference and hydrostatic
interactions. Within blood, the protein concentration can vary over several orders of magnitude, with protein half-life’s ranging from a few seconds to several months or longer. The protein
concentration in saliva is approximately a quarter of what is presented in blood which makes it easier to select and investigate low abundant proteins11,12. The composition of saliva is
though not as complex or varying as serum, it should therefore more accurately reflect the current condition of the body at any given time. The advantages with saliva might facilitate early
detection of many diseases, anticipate prognosis and improve clinical management13,14. Although the proteins in saliva reflects the body’s health and well-being, its use as a diagnostic
fluid has been hindered, mainly because of the lack of standardized techniques to collect saliva and comprehensive detection methods to study the protein content. Most studies using saliva
as a diagnostic medium use different collection methods and often lack to define characterization of the patients or sampling procedure4,15,16. This make it difficult to compare results from
different studies17. In general, most studies view saliva wrongly as a homogeneous body fluid. However, saliva is not a solitary fluid and cannot be viewed as such. Rather, it is a complex
mixture that comprises the secretions of three major glands (the parotid, submandibular and sublingual glands) each secreting a characteristic type of saliva, hundreds of minor salivary
glands, gingival crevicular fluid and debris. It is also not stable but constantly in change and the composition is affected among other things by sampling methodology, environment,
periodicity, oral hygiene, psychological status and general health18. As a diagnostic medium, saliva has disadvantages that needs consideration. For instance, owing to the diurnal variations
of certain biomolecules present in saliva, it does not always reliably reflect the concentrations of these molecules in serum. Consequently, since many factors can influence on salivary
secretion and composition a precise standard for saliva collection must be established19. The aim of the current study was to in depth analyse and compare different saliva collection methods
on a protein level using two dimensional gel (2-DE) based proteomic approach in a strictly controlled and characterized healthy cohort. To the best of our knowledge, this is the first study
that structurally has analysed the salivary proteome in whole and glandular saliva. MATERIALS AND METHODS PARTICIPANTS Ten healthy participants, five men and five age-matched women, with a
mean (SD) age of 23.6 ± 2.2 years where included in this study. Inclusion criteria were good general health, age ≥18 years, not taking any medications including oral contraceptives, body
mass index (BMI) < 30 kg/m2 and no complaints of oral dryness, mucosal lesions or other oral complaints. Participants had also to be free of fever/or cold and maintain exceptional oral
hygiene on the day of collection. Participants with less than 22 teeth and extensive prosthodontics rehabilitations were excluded. If oral examination indicated poor oral hygiene,
hyposalivation, oral complaints (e.g. peritonitis, caries, tooth ache), mucosal lesions, clinical signs of ongoing periodontal diseases or dental attrition, they were directly excluded from
further involvement in the study. Participants reporting elevated levels of psychological distress were also excluded. Participants were requested not to eat, drink or brush their teeth 1 h
prior to the trial, and not consume alcoholic beverages 24 h prior to collection. The participants arrived to the clinic in the early morning after they had eaten breakfast. A brief
interview was carried out by the examiner to ensure that they had followed the instructions, which all had. Participants were then asked to fill in validated questionnaires and then a
clinical examination was carried out as described below. All participants received information regarding the objectives and procedures of the study and gave their informed written consent
before the start of the study. The study protocol was approved by the Regional Ethical Review Board in Stockholm, Sweden (2014/17–31/3) and followed the guidelines according to the
Declaration of Helsinki. QUESTIONNAIRES AND CLINICAL EXAMINATION All participants underwent a dental examination by the same examiner. During the clinical examination participants were
checked for attrition as a sign of bruxism, decayed teeth and periodontal diseases, as well as the occlusion. Participants were also evaluated by the Swedish version of the Diagnostic
Criteria for TMD (DC/TMD) axis I and II20. The evidence-based protocol was used as a screening instrument for identification of participants with TMD signs that may not be presented during
the interview and general examination. Participants showing clinical signs of TMD (except for disc displacement without reduction which was considered as normal fluctuation) were excluded
from further involvement in the study. The following brief screening instruments included in the DC/TMD axis II questionnaire were used to assess symptoms of depression, somatic symptoms,
anxiety, mental stress and jaw function: the Patient Health Questionnaire (PHQ-9 and PHQ-15), the Generalized Anxiety Disorder scale (GAD-7), the Perceived Stress Scale-10 (PSS-10) and Jaw
Functional Limitation Scale (JFLS). The PHQ is a self-administered version of the Primary Care Evaluation of Mental Disorders diagnostic instrument for common mental disorders. PHQ-9 is a
well validated instrument to estimate and determine the severity of depression21. The PHQ-9 evaluates nine symptoms of depression, and assesses the level of the depression by the frequency
of these symptoms within the last 2 weeks. Scores range between 0 and 27, and scores of 5, 10 and 20 are considered the boundaries for mild, moderate, and severe depression respectively. The
PHQ-15 is a somatic symptom subscale and inquiries about the frequency of 15 somatic symptoms or symptom clusters that account for more than 90% of the physical complaints (excluding upper
respiratory tract symptoms). In determining the PHQ-15 score, each individual symptom is coded as 0, 1, or 2, and the total score ranges from 0 to 3022. The GAD-7 was used to estimate and
determine the severity of anxiety. The GAD-7 has been shown to be a reliable and valid measurement of anxiety and it can determine how often the participant has suffered from seven problems
over the past weeks, including nervousness and worry, where the severity is evaluated by the total score of the questionnaire (range: 0–21; higher scores indicate more severe anxiety).
Scores of 5, 10, and 15 are considered the cutoffs for mild, moderate, and severe anxiety23. The PSS-10 is a well document instrument to tap how unpredictable, uncontrollable and overloaded
participants find their lives. Participants rate the items on a 5-point Likert scale (0–4) where higher scores reflect greater perception of stress24. The PSS final score is obtained by
reversing the scores on the five positive items, and then summing all 10 items. The total score ranges from 0 to 40. Higher scores indicate higher perception of stress. The JFLS is designed
to assess patients jaw functional level that is both joint-specific and separated from pain-related disability. It has 20 items that address mastication, jaw mobility, and verbal and
emotional expression. Each item is rated on a numeric rating scale of 0 to 10 (where 0 indicated no limitation and 10 indicated severe limitation)25. SALIVA COLLECTION Unstimulated and
stimulated whole, parotid, and sublingual saliva was sampled (Fig. 1). Before saliva collection, participants were instructed to rinse their mouth with 10 ml of distilled deionized water for
30 seconds to remove debris and moisturise the mucosa. After 10 minutes of rest, saliva collection started. Between every sampling, participants rested for 15 minutes to neutralize salivary
flow. During the collection, participants were asked to not speak or leave the room. Further, they were instructed to keep their eyes open during salivation and not actively stimulate
salivary flow. All saliva samples were collected in the same order as presented below, in the same clinical room and at the same time, between 8:30 and 10:30 am. To prevent degradation of
sensitive proteins all samples were collected on ice in precooled polypropylene tubes. Immediately after collection a Protease Inhibitor Cocktail (Sigma Aldrich v/v 1:500) was added. All
samples where then centrifuged at 700 × g for 15 minutes at 4 °C to remove debris. The supernatant of each sample (upper 2/3) was fractionated into 100 μl tubes and frozen at −70 °C until
analyses. UNSTIMULATED WHOLE SALIVA Participants were instructed to sit upright with their head slightly titled forward. A 5 ml polypropylene tube was used to collect up to 5 ml of saliva
during passive drooling. Total drooling time was recorded, and salivary flow was measured. UNSTIMULATED PAROTID SALIVA The parotid gland secretion is voided in the oral cavity via the
Stensen’s duct at the vicinity of the parotid papilla opposite the maxillary second molar. In order to collect pure parotid saliva a modified polymethylmethacrylate Carlsson-Critten
collector was used according to authors’ description26,27. The orifices of the parotid duct was located, and the surrounding buccal mucosa dried with a gauze. The collector was placed
bilaterally on the buccal mucosa surrounding Stensen’s duct and secured with suction using a syringe. Parotid saliva were collected via 25 cm plastic tubing into a precooled tube. The first
3 drops of saliva were discarded to reduce the possibility of contamination. Total drooling time was recorded, and salivary flow was measured. UNSTIMULATED SUBLINGUAL SALIVA The
submandibular and sublingual gland secretions are voided in the oral cavity mainly via the Wharton duct and several smaller sublingual ducts which opens into the floor of the mouth. By
blocking the Stensen’s duct using Carlsson-Critten collector as described above, saliva could be collected from the floor of the mouth with a syringe every second minute. Samples from the
first 2 minutes were discarded to neutralize salivary flow. STIMULATED PAROTID SALIVA The Carlsson-Critten collector was placed bilaterally over the Stensen’s ducts orifices. Sterile aqueous
2% citric acid solution was applied bilateral on the lateral aspects of the tongue, at intervals of 30 seconds using a sterile cotton swab (max total 300 μl). Up to 1 ml parotid saliva was
collected as described above (unstimulated parotid saliva). STIMULATED SUBLINGUAL SALIVA Saliva Bio Oral Swab® (Salimetrics) consist of a polypropylene tube and contains absorbent pad made
by synthetic material. The pad was placed for around 2 minutes under the tongue while stimulating with 2% Citric acid as described above until the swab was fully covered in saliva. The fluid
was obtained by centrifugation (1500 × g, 15 min, 4 °C). Up to 2 ml saliva was extracted from the swab. STIMULATED WHOLE SALIVA Prior to collecting the last sample, participants were
instructed to again rinse their mouth with 10 ml of distilled deionized water for 30 seconds to remove any remains of the citric acid. Saliva samples were collected using paraffin gum (Orion
Diagnostica, Finland). For prestimulation, the participants were instructed to chew the gum until it was smooth and flexible. After 60 seconds of prestimulation, the participants were asked
to swallow the saliva present in the mouth. Subsequently, around 5 ml of whole saliva, stimulated by the same piece of paraffin, was collected for around 2 minutes, and salivary flow rate
was measured. PROTEIN ANALYSES The protein concentration was determined by two dimensional gel electrophoresis (2DE) using the Bio-Rad protein assay according to Bradford (Bio-Rad, Hercules,
CA, USA). Aliquots (300–400 μl) of the samples were desalted by gel filtration (PD-10 column, GE Healthcare) into 12 mM ammonium bicarbonate, pH 7.1. Proteins were lyophilized and dissolved
in 0.20 ml urea sample solution according to Görg _et al_.28. 2-DE was performed in a horizontal 2-DE setup (IPGphore and Multiphore, GE Healhcare) as described in detail previously29. The
samples (containing 50 μg protein) were applied by in-gel rehydration according to the manufacturer’s instructions for 12 h using low voltage (30 V) in pH 3–10 NL 18 cm IPGs (GE Heathcare,
Sweden). The proteins were then focused for up to 32 000 Vhs at a maximum voltage of 8000 V to assure a steady state. IPGs were immediately stored at −70 °C until analysed. The IPG gel
strips were equilibrated in SDS equilibration buffer (urea 6 M, SDS 4% (w/v), glycerol 30.5% (w/v) and Trizma-HCl 50 mM) and DTT 1% (w/v) for 15 minutes and then with iodacetamide 4.5% (w/v)
for additional 15 minutes. The second dimension (SDS-PAGE) was carried out by transferring the proteins to gradient gels (ExcelGel XL 245 × 180 × 0.5 mm, 12–14%T, 3%C) running at 20–40 mA,
up to 1000 V for about 5 h. STAINING AND IMAGE ANALYSIS For protein detection, the gels were stained with silver according to Shevchenko _et al_.30 with a detection limit of 5 ng/spot31
using a Stainer Shaker (Hoefer Processor Plus, Amersham Bioscience). The 2-DE protein patterns of the gels were visualized as digitized images using a CCD camera system, Versa Doc (Bio-Rad
Hercules, CA, USA) in combination with a computerized imaging 12-bit system designed for evaluation of 2-DE patterns (PDQuest V 8.0.1; Bio-Rad). The amount of protein in a spot was assessed
as background corrected optical density, integrated over all pixels in the spot and expressed as integrated optical density (IOD). In order to correct for differences in total silver stain
intensity between different 2-DE images, the amounts of the compared protein spots were quantified as optical density for individual spot per total protein intensity of all spots in the same
gel. Thus ppm-values (parts per million) for all proteins were generated, and were statistically evaluated for differences between collection methods. The protein spots were identified by
comparison to previously identified saliva proteins from a local database available at the laboratory29. STATISTICS Differences between males and females in the study were tested with
Mann-Whitney U-test using Statistica (StatSoft, Oklahoma, USA) since most of these variables did not show normal distribution. Repeated measurement analysis of variance (ANOVA) was used to
analyses differences in saliva collection with Bonferroni test as post hoc test when the ANOVA indicated significant differences. Correlations between variables were tested for statistical
significance with Spearman correlation test, adjusted for multiple comparisons according to Bonferroni. Descriptive data are presented as mean and standard deviation (SD) or median and
interquartile range (IQR). For all analyses, the significance level was set at P < 0.05. When investigating the multivariate correlations between the proteins and group membership.
Orthogonal Partial least squares discriminant analysis (OPLS-DA) was applied using SIMCA-P+ v.13.0 (UMETRICS, Umeå, Sweden)8,32,33. Principal component analysis (PCA), also available in
SIMCA-P+ was used to identify multivariate outliers. No multivariate outliers were identified. The variable influence on projection (VIP) indicates the relevance of each X-variable pooled
over all dimensions and the Y-variables – the group of variables that best explains Y. VIP > 1.2 was considered significant. RESULTS DATA OVERVIEW Anthropometric data of participants in
the study are presented in Table 1. Small differences in body mass and number of erupted teeth were observed between males and females in the cohort. Females in the cohort reported overall
higher levels of anxiety and somatic symptoms compared to the male equivalent (p = 0.032), however all psychological and psychosocial parameters were normal according to standard values.
Otherwise there were no statically significant differences regarding demographical, psychological and salivary parameters between males and females (Mann-Whitney U-test). Salivary flow
differed significantly between collection methods (F = 68.852; P = 0.000; Repeated measurement ANOVA) and was not affected by gender (F = 1.304; P = 0.305; Repeated measurement ANOVA). As
expected, whole paraffin stimulated saliva showed higher flow rate (2.2 ± 1.2 ml/min) compared to other collection methods (Repeated measurement ANOVA with Bonferroni post hoc test; P <
0.0001) (Table 2). The intra and inter subject coefficient of variation (CV) was calculated to show the extent of variability in relation to the mean value in the protein concentration. For
all six collection approaches the CV varied from 35% to 92%. Lowest CV was observed for stimulated whole saliva and the highest CV was observed for unstimulated whole saliva. The protein
concentration between individuals displayed CV between 28% up to 86% and reflects the intra-subject variability that can be expected in cross sectional studies. Contrary, no significant
differences in protein concentration was found between unstimulated and stimulated salivary collection approaches (F = 1.899; P = 1.304: Repeated Measures ANOVA). The protein concentration
ranged from 776 to 12 906 μg/ml (Table 2). There was no correlation observed between the protein concentrations of the different collection methods before and after desalting (P > 0.05;
Spearman Rank Order Correlations). Unstimulated parotid saliva displayed as expected very slow flow rate (0.029 ± 0.026 ml/min per gland). Due to the overall slow flow rate and great
variations in salivary flow rate of parotid saliva, sufficient amount for analysis could not be collected from all participants. Thus, saliva was collected from accessible gland from seven
subjects but sufficient volume for 2DE analysis could only be obtained from three subjects. PROTEOME PATTERNS IN THE DIFFERENT SALIVA COLLECTION METHODS The 2-DE protein patterns were
visualized by silver staining and using spot detection wizards, 94 to 462 protein spots could be detected in each gel. Least number of protein spots were detected in the unstimulated and
stimulated parotid saliva. The typical protein patterns for the different saliva samples are shown in Fig. 2. The protein pattern showed great differences between different sampling methods.
The largest differences were detected in the _pI_ range of 3 to 5 and molecular weight 10 to 20 kDa as marked by a circle in Fig. 2. There were significant lower levels of
Prolactin-inducible protein and Cystatin S in unstimulated and stimulated parotid saliva compared to the other saliva samples. A typical 2DE-map illustrating the salivary protein pattern and
expression is shown in Fig. 3. The integrated optical density (IOD) of the typical salivary proteins (alpha-amylase, Cystatin S, Cystatin N and Prolactin-inducible protein) in the
individual gel from the different saliva samples are shown in the diagrams. There were no differences in the level of alpha-amylase between the different saliva samples. A great individual
variation was found for Cystatin SN in all saliva samples. The level of Prolactin-inducible protein and 3 different isoforms of Cystatin S were differentially expressed in the different
saliva samples. A total of 213 protein spots were matched. At least 50% of these were presented in each salivary sampling methods and thus was eligible for statistical validation. 25 protein
spots with VIP > 1.2 combined with jack-knifed 95% confidence intervals in the regression coefficients plot not including zero were considered significant (Table 3) and discriminated the
different saliva collection methods (Fig. 4). Most spots of the 25 significant proteins were found to be identical to those identified in unstimulated whole saliva (Fig. 3A). Four spots
(4003, 6204, 8104 and 8507) were more abundant in stimulated sublingual saliva. Spot number 1001 was found to be highly specific to stimulated whole saliva and stimulated sublingual saliva.
Spot number 2002 was detected in almost all gels (n = 9) in stimulated whole saliva samples and only in 4 gels from unstimulated whole saliva and stimulated sublingual saliva and in 3 gels
from unstimulated sublingual saliva. Unstimulated and stimulated parotid saliva gels lack the spot 2002. DISCUSSION Determination of the variability in the salivary proteome is a
prerequisite for the development of saliva as a diagnostic and prognostic tool for biomarker discovery. In this context, it is important that technical variability induced by sample
collection and processing is kept at minimum to be able to reproducibly assess inter-subject variability in states of health and disease15. In the current study, the effect of unstimulated
and stimulated saliva collection methods on the proteome profile was investigated. Using a small cohort and multiple samples allowed to detect major changes and reduced the influence of
individual differences and external factors34. The participants were sex matched and closely related in age in order to minimize the influence of these factors on the flow rate and protein
expression, since it has been described in earlier studies35. Salivary flow showed great differences in accordance with previous reported levels36,37,38 and was directly associated with the
method for saliva collection. The relative contributions of the different glands to whole saliva vary depending upon the method of collection, the degree of stimulation, age and even the
time of the day39. The variable nature of saliva secretion suggest that different approaches may have to be adopted when studying its composition or its possible role as an indicator for
particular physiological states. There is now a considerable body of literature on the diagnostic possibilities of saliva, but there are still no standardized techniques for saliva sample
collection. In different studies, different sampling methods are often used and many articles provide no or little description about the preparation of the patient or sampling procedure10.
Additionally, the characterization of the participants are often insufficient without a proper clinical examination. Most papers in saliva proteomics focus exclusively on studying whole
saliva1,15,29 because it can be obtained very easily by spitting into a test tube or allowing it to dribble from the mouth. Very few have focused on ductal saliva obtained from the different
salivary glands16,17,40. And, as far as we know, this is the first study focusing on structurally comparing the protein expression of both whole and glandular saliva in a carefully
characterized and clinically examined cohort. The results demonstrated that different collection methods provided clear differences in the snapshot of the salivary proteome. Waltz and
co-authors described differences in the protein expression between whole, parotid and sublingual-submandibular saliva17. Using a similar approach, but a much smaller cohort (n = 4), they
reported less protein spots in glandular saliva compared to the present study. Though, the authors chose to filter saliva prior to desalting with the purpose of removing large insoluble
material and bacterial contamination. This could consequently lead to loss of proteins and should not be needed if the participants are examined clinically for exclusion of oral disease and
neglected oral hygiene prior to collection17. In another more recent study, also in a small uncharacterized cohort (n = 6), significantly different proteoform profiles were resolved with
high reproducibility between stimulated parotid and submandibular/sublingual secretion16. As indicated in earlier studies, samples collected by the same method displayed closer correlation
than those obtained from different methods36. Our results revealed close correlation between the protein patterns of stimulated parotid saliva and unstimulated parotid saliva as well as
unstimulated sublingual saliva and unstimulated whole saliva. The parotid gland’s main function is carbohydrate digestion and formation of the food bolus, consequently serous cells
predominate, making the gland secrete mainly serous secretory product high in salivary alpha-amylase and electrolyte content. This may explain the somehow low level of specific protein spots
and high level of salivary alpha-amylase in parotid saliva. The main functions of sublingual saliva are to lubricate the oral cavity and protect against chemical and mechanical influence.
The saliva is dominated by mucous cell secretion which makes it rich in mucins and other glycoproteins. The glycoproteins (e.g. mucins) are high molecular weight polypeptides that stick
together and have very low solubility, and cannot migrate through the polyacrylamide gel resulting in their aggregation41,42. This may in turn contribute to the somehow lower level of
specific protein spots. The close relationship between unstimulated sublingual saliva and unstimulated whole saliva may be due to the high content of sublingual saliva in the unstimulated
whole saliva. The major component of unstimulated saliva is derived from the submandibular glands (60%), followed by the parotid glands (20%), minor salivary glands (15%), and sublingual
glands (5%)43. The submandibular glands are responsible for producing high concentration of glycoproteins which consequently makes unstimulated whole saliva and unstimulated sublingual
saliva viscous and difficult to technically process. The protein pattern of stimulated whole saliva and stimulated sublingual saliva, however, differed significantly. This finding may be
explained by the great differences in salivary quality and stimulation method. Stimulated sublingual saliva was collected with an absorbent material under the tongue while chemically
stimulating with 2% citric acid on the lateral aspect of the tongue, while stimulated whole saliva was collected through mechanical stimulation by chewing on paraffin gum. The parotid gland
normally responds to stimulation and proprioceptive activity, via masticatory muscles and periodontal ligaments, and accordingly contribute mostly to the stimulated whole saliva (60%)37,41.
In addition to the differences in the proteomic profile, the relative amount of expressed proteins differed between salivary methods. The strength of electrophoreses based proteomics is that
it allow us to separate and study different protein isoforms34. The finding that the same protein, e.g. Cystatin S and Prolactin-inducible protein, was identified in different spots in
different saliva types suggests the presence of several isoforms. The amount of each isoforms also showed different expression in different saliva collection approaches. A typical example is
the spot number 104 with the highest VIP-value (Table 3) that was highly present in unstimulated whole saliva and sublingual saliva but not in parotid saliva. The next spot number with high
VIP-value, 105, was highly expressed only in unstimulated whole saliva and stimulated sublingual saliva. Both protein spots were identified as Prolactin-inducible protein. This protein is a
common saliva protein that is secreted as glycosylated and non-glycosylated protein and has a roll in innate immunity and mucosal defence44,45. Accordingly, when using saliva as diagnostic
or prognostic fluid, scientists need to carefully consider the saliva collection approach. This is highly important when studying the salivary proteome profile for particular physiological
states in order to find biological markers. Although superficially it may appear that the collection of saliva is a simple non-invasive procedure, there are limitations with the different
collection methods that may not be obvious. The parotid glands are known to secrete several organic and inorganic substances. The saliva composition is highly viscous and easy to process.
The collection is however somehow time-consuming and require special devices e.g. parotid cups26. Unstimulated parotid saliva presents very low flow-rate and is consequently not suitable
when larger sample volumes are needed. This collection method is thus not practical in large cross-sectional studies or clinical studies involving patients with serious health conditions.
The submandibular and sublingual glands are closely situated and can sometimes share the same ducts. Therefore, it is difficult to separate the saliva from these glands untraumatically and
with certainty. This is the reason why saliva were sampled from both glands together in the current study46. The use of absorbent materials to collect saliva is common in saliva research but
could be problematic when the expected volume of saliva is small, and can negatively affect the validity of the assays when the saliva is filtered through a cotton/pad. The collection of
unstimulated whole saliva, by simply allowing saliva to accumulate in the mouth and then allowing it to dribble out through a funnel or straw in a tube, is subject to considerable variation
as the participants tries, to varying extents, speed up the collection by tongue and cheek movements. This could partly explain the large variability in saliva secretion and protein
concentration between subjects. In order to diminish that risk it is recommended that researchers give clear instructions to which participants must adhere before and during saliva
collection. Paraffin-stimulated whole saliva could therefore be recommended due to its simplicity. This collection method also resulted in the least variability (CV 35%), the highest volume
of saliva in this study and low levels of glycoproteins. In spite of these limitations, there are compelling reasons for exploring saliva as a diagnostic tool in proteomics to identify
specific protein biomarkers for diseases. In conclusion the results of this study demonstrated that different saliva collection methods provide clear differences in the snapshot of the
salivary proteome. Based on the comparison of unstimulated and stimulated salivary collection approaches, it could be suggested that stimulated whole saliva may be a preferable collection
method based on the simplicity of the collection method and low variability. The protein expression showed thus great variability between methods. The results emphases the importance of
consistency when collecting saliva samples, which should be more important than the collection approach itself. ADDITIONAL INFORMATION HOW TO CITE THIS ARTICLE: Jasim, H. _et al_. The
proteomic profile of whole and glandular saliva in healthy pain-free subjects. _Sci. Rep._ 6, 39073; doi: 10.1038/srep39073 (2016). PUBLISHER'S NOTE: Springer Nature remains neutral
with regard to jurisdictional claims in published maps and institutional affiliations. REFERENCES * Hu, S., Loo, J. A. & Wong, D. T. Human saliva proteome analysis. Annals of the New
York Academy of Sciences 1098, 323–329, doi: 10.1196/annals.1384.015 (2007). Article CAS ADS PubMed Google Scholar * Dodds, M., Roland, S., Edgar, M. & Thornhill, M. Saliva A review
of its role in maintaining oral health and preventing dental disease. Bdj Team 2, 15123, doi: 10.1038/bdjteam.2015.123 (2015). Article Google Scholar * Fabian, T. K., Hermann, P., Beck,
A., Fejerdy, P. & Fabian, G. Salivary defense proteins: their network and role in innate and acquired oral immunity. International journal of molecular sciences 13, 4295–4320, doi:
10.3390/ijms13044295 (2012). Article CAS PubMed PubMed Central Google Scholar * Fischer, H. P., Eich, W. & Russell, I. J. A possible role for saliva as a diagnostic fluid in
patients with chronic pain. Seminars in arthritis and rheumatism 27, 348–359 (1998). Article CAS Google Scholar * Levine, J. D., Goetzl, E. J. & Basbaum, A. I. Contribution of the
nervous system to the pathophysiology of rheumatoid arthritis and other polyarthritides. Rheumatic diseases clinics of North America 13, 369–383 (1987). CAS PubMed Google Scholar *
Ernberg, M., Hedenberg-Magnusson, B., Alstergren, P. & Kopp, S. The level of serotonin in the superficial masseter muscle in relation to local pain and allodynia. Life sciences 65,
313–325 (1999). Article CAS Google Scholar * Castrillon, E. E. et al. Interstitial glutamate concentration is elevated in the masseter muscle of myofascial temporomandibular disorder
patients. Journal of orofacial pain 24, 350–360 (2010). PubMed Google Scholar * Olausson, P. et al. Protein alterations in women with chronic widespread pain–An explorative proteomic study
of the trapezius muscle. Scientific reports 5, 11894, doi: 10.1038/srep11894 (2015). Article ADS PubMed PubMed Central Google Scholar * Gerdle, B., Ghafouri, B., Ernberg, M. &
Larsson, B. Chronic musculoskeletal pain: review of mechanisms and biochemical biomarkers as assessed by the microdialysis technique. Journal of pain research 7, 313–326, doi:
10.2147/jpr.s59144 (2014). Article PubMed PubMed Central Google Scholar * Hu, S., Loo, J. A. & Wong, D. T. Human saliva proteome analysis and disease biomarker discovery. Expert
review of proteomics 4, 531–538, doi: 10.1586/14789450.4.4.531 (2007). Article CAS PubMed Google Scholar * Lee, Y. H. & Wong, D. T. Saliva: an emerging biofluid for early detection
of diseases. American journal of dentistry 22, 241–248 (2009). PubMed PubMed Central Google Scholar * Pfaffe, T., Cooper-White, J., Beyerlein, P., Kostner, K. & Punyadeera, C.
Diagnostic potential of saliva: current state and future applications. Clinical chemistry 57, 675–687, doi: 10.1373/clinchem.2010.153767 (2011). Article CAS PubMed Google Scholar * Liu,
J. & Duan, Y. Saliva: a potential media for disease diagnostics and monitoring. Oral oncology 48, 569–577, doi: 10.1016/j.oraloncology.2012.01.021 (2012). Article PubMed Google Scholar
* Messana, I., Inzitari, R., Fanali, C., Cabras, T. & Castagnola, M. Facts and artifacts in proteomics of body fluids. What proteomics of saliva is telling us? Journal of separation
science 31, 1948–1963, doi: 10.1002/jssc.200800100 (2008). Article CAS PubMed Google Scholar * Al Kawas, S., Rahim, Z. H. & Ferguson, D. B. Potential uses of human salivary protein
and peptide analysis in the diagnosis of disease. Archives of oral biology 57, 1–9, doi: 10.1016/j.archoralbio.2011.06.013 (2012). Article CAS PubMed Google Scholar * Wu, S. et al.
Quantitative analysis of human salivary gland-derived intact proteome using top-down mass spectrometry. Proteomics 14, 1211–1222, doi: 10.1002/pmic.201300378 (2014). Article CAS PubMed
Google Scholar * Walz, A. et al. Proteome analysis of glandular parotid and submandibular-sublingual saliva in comparison to whole human saliva by two-dimensional gel electrophoresis.
Proteomics 6, 1631–1639, doi: 10.1002/pmic.200500125 (2006). Article CAS PubMed Google Scholar * de Almeida Pdel, V., Gregio, A. M., Machado, M. A., de Lima, A. A. & Azevedo, L. R.
Saliva composition and functions: a comprehensive review. The journal of contemporary dental practice 9, 72–80 (2008). Article Google Scholar * Castagnola, M. et al. The human salivary
proteome: a critical overview of the results obtained by different proteomic platforms. Expert review of proteomics 9, 33–46, doi: 10.1586/epr.11.77 (2012). Article CAS PubMed Google
Scholar * Schiffman, E. et al. Diagnostic Criteria for Temporomandibular Disorders (DC/TMD) for Clinical and Research Applications: recommendations of the International RDC/TMD Consortium
Network* and Orofacial Pain Special Interest Groupdagger. Journal of oral & facial pain and headache 28, 6–27, doi: 10.11607/jop.1151 (2014). Article Google Scholar * Kroenke, K.,
Spitzer, R. L. & Williams, J. B. The PHQ-9: validity of a brief depression severity measure. Journal of general internal medicine 16, 606–613 (2001). Article CAS Google Scholar *
Kroenke, K., Spitzer, R. L. & Williams, J. B. The PHQ-15: validity of a new measure for evaluating the severity of somatic symptoms. Psychosomatic medicine 64, 258–266 (2002). Article
Google Scholar * Lowe, B. et al. Validation and standardization of the Generalized Anxiety Disorder Screener (GAD-7) in the general population. Medical care 46, 266–274, doi:
10.1097/MLR.0b013e318160d093 (2008). Article PubMed Google Scholar * Nordin, M. & Nordin, S. Psychometric evaluation and normative data of the Swedish version of the 10-item perceived
stress scale. Scandinavian journal of psychology 54, 502–507, doi: 10.1111/sjop.12071 (2013). Article PubMed Google Scholar * Ohrbach, R., Larsson, P. & List, T. The jaw functional
limitation scale: development, reliability, and validity of 8-item and 20-item versions. Journal of orofacial pain 22, 219–230 (2008). PubMed Google Scholar * Shannon, I. L., Prigmore, J.
R. & Chauncey, H. H. Modified Carlson-Crittenden device for the collection of parotid fluid. Journal of dental research 41, 778–783 (1962). Article CAS Google Scholar * Lashley, K. S.
Reflex secretion of the human parotid gland. Journal of Experimental Psychology 1, 461–493, doi: doi.org/10.1037/h0073282 (1916). Article Google Scholar * Gorg, A. et al. The current
state of two-dimensional electrophoresis with immobilized pH gradients. Electrophoresis 21, 1037–1053, doi: 10.1002/(sici)1522-2683(20000401)21:6<1037::aid-elps1037>3.0.co;2-v
(2000). Article CAS PubMed Google Scholar * Ghafouri, B., Tagesson, C. & Lindahl, M. Mapping of proteins in human saliva using two-dimensional gel electrophoresis and peptide mass
fingerprinting. Proteomics 3, 1003–1015, doi: 10.1002/pmic.200300426 (2003). Article CAS PubMed Google Scholar * Shevchenko, A., Wilm, M., Vorm, O. & Mann, M. Mass spectrometric
sequencing of proteins silver-stained polyacrylamide gels. Analytical chemistry 68, 850–858 (1996). Article CAS Google Scholar * Swain, M. & Ross, N. W. A silver stain protocol for
proteins yielding high resolution and transparent background in sodium dodecyl sulfate-polyacrylamide gels. Electrophoresis 16, 948–951 (1995). Article CAS Google Scholar * Eriksson, L.
et al. Multi- and megavariate data analysis. Part I-II, Basic principles and applications 2nd edn (Umetrics AB, 2006). * Wheelock, A. M. & Wheelock, C. E. Trials and tribulations of
‘omics data analysis: assessing quality of SIMCA-based multivariate models using examples from pulmonary medicine. Molecular bioSystems 9, 2589–2596, doi: 10.1039/c3mb70194h (2013). Article
CAS PubMed Google Scholar * Rabilloud, T. & Lelong, C. Two-dimensional gel electrophoresis in proteomics: a tutorial. Journal of proteomics 74, 1829–1841, doi:
10.1016/j.jprot.2011.05.040 (2011). Article CAS PubMed Google Scholar * Fleissig, Y. et al. Comparative proteomic analysis of human oral fluids according to gender and age. Oral diseases
16, 831–838, doi: 10.1111/j.1601-0825.2010.01696.x (2010). Article CAS PubMed Google Scholar * Golatowski, C. et al. Comparative evaluation of saliva collection methods for proteome
analysis. Clinica chimica acta; international journal of clinical chemistry 419, 42–46, doi: 10.1016/j.cca.2013.01.013 (2013). Article CAS PubMed Google Scholar * Topkas, E., Keith, P.,
Dimeski, G., Cooper-White, J. & Punyadeera, C. Evaluation of saliva collection devices for the analysis of proteins. Clinica chimica acta; international journal of clinical chemistry
413, 1066–1070, doi: 10.1016/j.cca.2012.02.020 (2012). Article CAS PubMed Google Scholar * Baum, B. J. Evaluation of stimulated parotid saliva flow rate in different age groups. Journal
of dental research 60, 1292–1296 (1981). Article CAS Google Scholar * Helmerhorst, E. J. & Oppenheim, F. G. Saliva: a dynamic proteome. Journal of dental research 86, 680–693 (2007).
Article CAS Google Scholar * Denny, P. et al. The proteomes of human parotid and submandibular/sublingual gland salivas collected as the ductal secretions. Journal of proteome research 7,
1994–2006, doi: 10.1021/pr700764j (2008). Article CAS PubMed PubMed Central Google Scholar * Frejerskov., O., Nyvad., B. & Kidd., E. Dental caries: the disease and its clinical
management 3rd edn (John Wiley Sons, 2015). * Zalewska, A., Zwierz, K., Zolkowski, K. & Gindzienski, A. Structure and biosynthesis of human salivary mucins. Acta biochimica Polonica 47,
1067–1079 (2000). CAS Google Scholar * Pedersen, A. M., Bardow, A., Jensen, S. B. & Nauntofte, B. Saliva and gastrointestinal functions of taste, mastication, swallowing and digestion.
Oral diseases 8, 117–129 (2002). Article CAS Google Scholar * Murphy, L. C., Tsuyuki, D., Myal, Y. & Shiu, R. P. Isolation and sequencing of a cDNA clone for a prolactin-inducible
protein (PIP). Regulation of PIP gene expression in the human breast cancer cell line, T-47D. The Journal of biological chemistry 262, 15236–15241 (1987). CAS PubMed Google Scholar *
Mirels, L., Hand, A. R. & Branin, H. J. Expression of gross cystic disease fluid protein-15/Prolactin-inducible protein in rat salivary glands. The journal of histochemistry and
cytochemistry: official journal of the Histochemistry Society 46, 1061–1071 (1998). Article CAS Google Scholar * Hu, S. et al. Differentially expressed protein markers in human
submandibular and sublingual secretions. International journal of oncology 25, 1423–1430 (2004). CAS PubMed Google Scholar Download references ACKNOWLEDGEMENTS We gratefully acknowledge
Professor Dan Ericson for his help with the Carlsson-Critten collector. The research was supported by the Swedish Research Council, the Stockholm County Council (ALF project and SOF),
Swedish Dental Society and Folktandvården Stockholm AB. AUTHOR INFORMATION AUTHORS AND AFFILIATIONS * Department of Dental Medicine, Section of Orofacial Pain and Jaw Function, Karolinska
Institutet, and Scandinavian Center for Orofacial Neurosciences (SCON), Huddinge, SE 14104, Sweden Hajer Jasim, Britt Hedenberg-Magnusson & Malin Ernberg * Folktandvården Stockholm AB,
Sweden Hajer Jasim & Britt Hedenberg-Magnusson * Division of Community Medicine, Department of Medical and Health Sciences, Faculty of Health Sciences, Linköping University and Pain and
Rehabilitation Center, Anaesthetics, Operations and Specialty Surgery Center, Region Östergötland Patrik Olausson & Bijar Ghafouri Authors * Hajer Jasim View author publications You can
also search for this author inPubMed Google Scholar * Patrik Olausson View author publications You can also search for this author inPubMed Google Scholar * Britt Hedenberg-Magnusson View
author publications You can also search for this author inPubMed Google Scholar * Malin Ernberg View author publications You can also search for this author inPubMed Google Scholar * Bijar
Ghafouri View author publications You can also search for this author inPubMed Google Scholar CONTRIBUTIONS B.G., B.M., H.J., M.E. and P.O. designed the experiment. H.J. examined and
collected the samples. B.G. and H.J. performed the analysis. B.G., H.J. and P.O. performed the statistics. H.J. wrote the first draft of the article and prepared figures and tables. All
authors wrote and revised the manuscript and approved the final version of the manuscript. ETHICS DECLARATIONS COMPETING INTERESTS The authors declare no competing financial interests.
RIGHTS AND PERMISSIONS This work is licensed under a Creative Commons Attribution 4.0 International License. The images or other third party material in this article are included in the
article’s Creative Commons license, unless indicated otherwise in the credit line; if the material is not included under the Creative Commons license, users will need to obtain permission
from the license holder to reproduce the material. To view a copy of this license, visit http://creativecommons.org/licenses/by/4.0/ Reprints and permissions ABOUT THIS ARTICLE CITE THIS
ARTICLE Jasim, H., Olausson, P., Hedenberg-Magnusson, B. _et al._ The proteomic profile of whole and glandular saliva in healthy pain-free subjects. _Sci Rep_ 6, 39073 (2016).
https://doi.org/10.1038/srep39073 Download citation * Received: 07 July 2016 * Accepted: 17 November 2016 * Published: 15 December 2016 * DOI: https://doi.org/10.1038/srep39073 SHARE THIS
ARTICLE Anyone you share the following link with will be able to read this content: Get shareable link Sorry, a shareable link is not currently available for this article. Copy to clipboard
Provided by the Springer Nature SharedIt content-sharing initiative